David Eisner, Dario DiFrancesco and Denis Noble reflect on their original paper in the Royal Society's Philosophical Transactions.
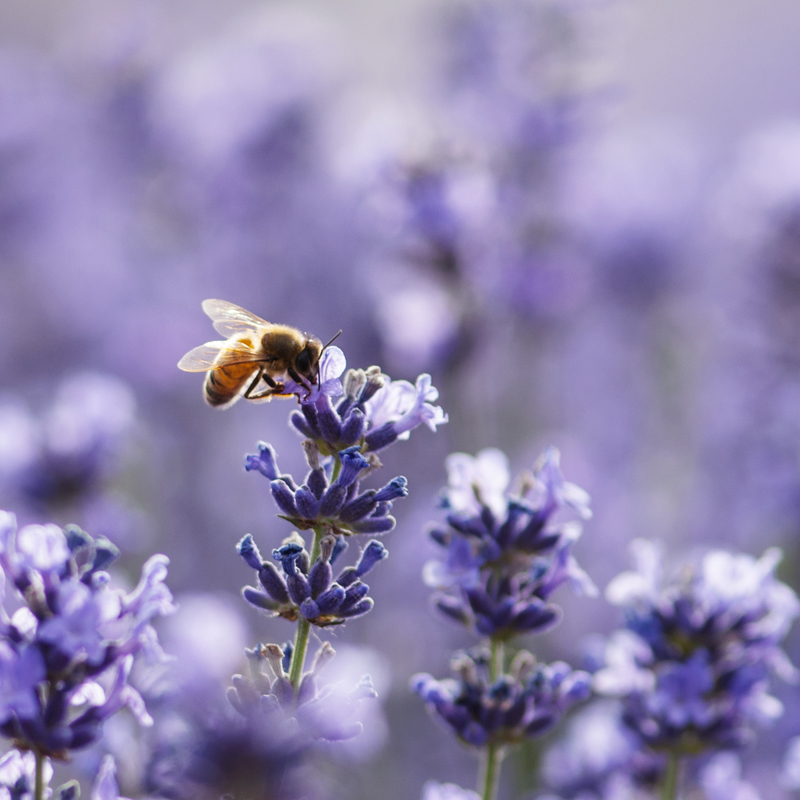
In 1985 Dario DiFrancesco and Denis Noble produced their classic paper in Philosophical Transactions of the Royal Society entitled “A model of cardiac electrical activity incorporating ionic pumps and concentration changes”. This work had a profound impact on the field and its publication has been celebrated in the anniversary edition of Phil Trans B with an article written by David Eisner and colleagues. David Eisner recently met up with Dario and Denis to reflect on their original work, the scientific environment at the time and how the field has developed.
David Eisner (DE). What was the major breakthrough that this paper made? What challenges did it overcome?
Dario DiFrancesco (DD). Two major breakthroughs had revolutionized the concept behind cellular electrical activity in the late 70s: 1) the discovery of a novel mechanism for generating pacemaker activity and controlling pacing rate (the funny current), and 2) a reconsideration of the mechanisms governing calcium (Ca) flow across the membrane, with a major function exerted by the Na-Ca exchange with a Na-Ca stoichiometry of 3:1 which made the exchanger generate an electric current and therefore able to affect electrical activity.
The discovery of the funny current in the natural pacemaker tissue of the heart, the sino-atrial node (SAN), had been followed by the re-interpretation of the IK2 current in the Purkinje fibres. Taken together, this provided solid evidence that the pacing mechanisms in all pacing cardiac regions were based on the same components, and established a new concept of cardiac pacemaking associated with the development of an inward current, rather than the decay of an outward current during the diastolic phase of the action potential. Literally a true “revolution”, in the sense that the original concept had been turned upside-down.
The model also included, for the first, time activity-dependent changes in ion concentrations. It was particularly curious for me, for example, to see how previous experiments which had been interpreted as providing evidence for a pure K current decaying during diastole, could be reproduced by the real process of an increasing inward (funny) current. Indeed, the model reproduced all these experiments with remarkable accuracy! This was really a surprising, and very gratifying, result.
DE. How was the model tested and did it stand up to this testing?
Denis Noble (DN). The first validation is the voltage and sodium dependence of the electric current carried by the exchanger. We used a modification of equations developed by LJ Mullins. But we could not know that within a year Junko Kimura (one of our former students) and Akinori Noma in Japan would produce experimental results that bear an almost uncanny resemblance to the Mullins equations. Frankly I think we were lucky. We could not have known that would be the case, and such luck is rare enough in biological modelling work.
The second validation has been the painstaking work of our interviewer, David Eisner, and his colleagues showing that the great majority of calcium efflux is indeed carried by the sodium-calcium exchanger.
But it is also fair to say that we missed some key features. It was left to Don Hilgemann, starting from our model, to deal with intracellular calcium buffering and to link the calcium dynamics more correctly to the initiation of contraction.
Perhaps the best tribute to the extent to which the model has stood the test of time is just how many models developed subsequently have taken our model as the starting point.
DE. To what extent can modelling replace or reduce animal experiments?
DD. This is an important issue for all physiologists. Modelling can reduce but cannot replace animal experimentation, at least at present. One of the aims of modelling is to simulate real life, and this process keeps improving with time along with the complexity of the numerical approach.
However we are still far from being able to simulate biological processes, even in the simplest possible system like a cell. How an organism reacts to a change in the environment or in any of the thousands of elementary components active in the cell itself is often unpredictable.
There are special situations, however, where the numerical approach can indeed provide information able to predict the cell/tissue response, and modelling of cardiac electrical activity is an example. For example, it is relatively straightforward to predict what kind of effect might be caused by a drug acting specifically on an ion channel or transporter. Comparing data from model computations with and without the drug effect on its specific target can thus help identify the effect on the whole cell or tissue, reducing the need to run screening experiments on animals.
Modelling and experimental data are most of the time complementary to each other and need to be run in parallel. There are reciprocal advantages in validating experimental data with modelling and verifying modelling with experimental data, and progress in one type of approach helps progress of the other.
DE. What tools did you have available to you and how does this compare to now?
DN. We had to use punched card programs to upload programs onto large mainframe computers that had only a small fraction of the computing power of a modern desktop or laptop. We had to leave the cards to be loaded overnight and hope to return in the morning to see some results. All the data was exported as printed numbers on rolls of paper. We had to convert all of this into graphs by hand. Just two years later, in 1987, we were able to use one of the first IBM PCs. The results would appear on the screen as a series of dots taking hours to produce what can now be done in just a few milliseconds. I think I have lived through about 40 Moore’s laws, the theory that computing power doubles every 18 months. So I have seen a 240-fold increase in computing power. That’s an increase of roughly one trillion.
DE. What discoveries are happening now in this field? What single question would you like to see answered?
DD. My interest continues to be the heart but one should also take note of the ever growing modelling work in fact in many other fields. The use of numerical modelling has kept increasing since our 1985 paper was published. Significant changes have been made to intracellular compartmentation and distribution and homeostasis of ions, particularly of Ca ions given their importance in the overall cell repetitive activity. This is based on a continuous development of more and more advanced techniques for measuring ion concentrations inside the cell with high time resolution, techniques that were not available in 1985. Technical advancements have also involved the subject of cell-to-cell communication and the measurement of electrical signal propagation along complex structures such as the heart.
With the parallel explosion of the digital era and the once unthinkable computational speed and memory storage capabilities of today’s computers, it is now possible to imagine that we will one day be able to reconstruct the electrical and mechanical activity of the entire heart by coupling single-cell models as in a virtual heart, a project that is already being pursued.