A new collection of Royal Society Biographical Memoirs celebrates the pioneers who uncovered the structures, functions, and relationships of proteins and genetic material.
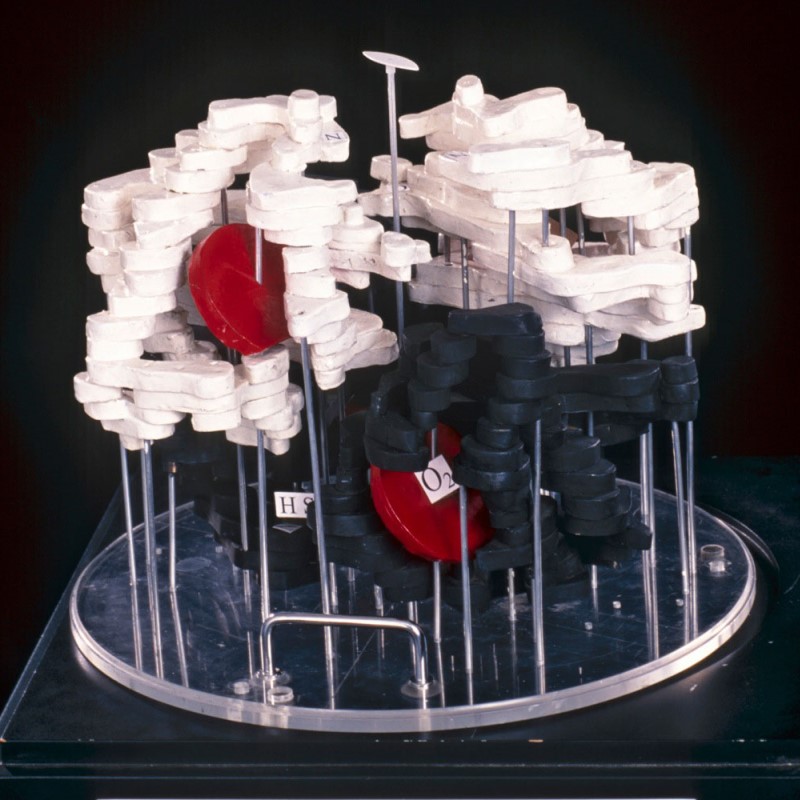
The twin fields of structural biology and genetics/genomics have spawned a global biotechnology industry and made it possible to read the ‘book of life’, the genetic instructions that control how organisms grow and respond to their environments. A new collection of Biographical Memoirs of Fellows of the Royal Society describes how these fields developed through the contributions of many of the leading pioneers in these fields in the 20th century. Here, Georgina Ferry, science writer and biographer, traces this history.
Both the origins of the term ‘molecular biology’ and its exact meaning are topics of dispute. However, it is possible to trace two distinct threads that ultimately led to our present understanding of the molecular components of the body, and especially how information in the genetic material of living organisms is interpreted by cellular machinery to produce the proteins that implement their growth, renewal and eventual decay.
One branch depended on the development of physical techniques that made it possible to interrogate biological material and discern its structure at nanometre scales. The other deployed studies of microorganisms to tease out first the identity of the genetic material, and then the biochemistry of its expression.
The originators of the structural biology tradition were the father and son team of William Henry Bragg and his son Lawrence. Their successful demonstration that by passing X-rays through crystals of material such as rock salt and capturing the diffracted image one might infer the arrangement of atoms within the crystal launched the field of X-ray crystallography.
Original Bragg X-ray spectrometer, developed by William Bragg at Leeds University, 1910-1926. Science Museum Group Collection. © The Board of Trustees of the Science Museum. Released under licence CC BY-NC-SA 4.0.
Bragg the elder encouraged his colleagues at University College, London, to work with organic material. Moving from London to Leeds in 1928, his former student William Astbury began to use the technique to investigate the structure of protein-rich biological fibres such as horn and hair, and in 1938 he made the first X-ray studies of DNA fibres with his student Florence Bell.
Meanwhile John Desmond Bernal left UCL for Cambridge and set up an X-ray lab in the Cavendish Laboratory, with a mission to solve biological structures such as proteins. Working with his student Dorothy Crowfoot (later Dorothy Crowfoot Hodgkin), in 1934 he became the first to obtain an X-ray diffraction image of a crystalline protein, the digestive enzyme pepsin. Crowfoot returned to Oxford to set up her own lab and immediately followed up with the first images of the hormone insulin. Two years later Max Perutz arrived in Bernal’s lab as a graduate student from Vienna and began work on crystals of haemoglobin, the oxygen-carrying protein that gives blood its red colour. Because of the size and complexity of protein molecules, the X-ray data obtained by these pioneers remained uninterpretable for more than two decades. But steady technological advances gave hope.
In parallel with these developments, the first advances had been made in understanding the molecular biology of the gene. Its nature was uncertain until Oswald Avery’s 1944 experiment with pneumococcal bacteria proved that DNA was the ‘transforming principle’ that could convert one strain into another. By this time George Beadle and his colleague Edward Tatum, working with a mould, had established the ‘one gene, one enzyme’ principle, that genes act by directing the production of specific enzymes. In 1945 Max Delbrück and colleagues set up the Phage Group (pictured above, Caltech 1949) , an informal network that studied the genetics of viruses that infect bacteria, thereby answering many questions in genetics.
Max Delbruck with the Phage Group. Left to right: Jean Jacques Weigle, Ole Maaloe, Elie Wollman, Gunther S. Stent, Max Delbruck, and G. Soli. Caltech, 1949. From the Caltech archives.
In 1946 the Medical Research Council funded Perutz to head a small research unit within the Cavendish Laboratory, working on the molecular structure of biological systems. The members of the Cambridge unit were dismayed when Linus Pauling of CalTech solved the structure of the alpha helix, a structural motif common to proteins, in 1951, despite important work by Francis Crick on helical diffraction theory. However, the arrival of Jim Watson at the unit persuaded Crick to focus instead on DNA. Partly thanks to access to crystallographic data on DNA collected at King’s College, London by Maurice Wilkins and Rosalind Franklin, Crick and Watson built a broadly correct model of the DNA double helix in 1953. Largely unsung, Struther Arnott, working at King’s with Wilkins, spent the 1960s correcting errors in the model. Crick, Watson and Wilkins won the Nobel prize for physiology in 1962, while researchers soon afterwards succeeded in cracking the genetic code.
X-ray diffraction exposure of B type DNA, AKA ‘photo 51’, produced at King’s (Wellcome Collection. King’s College London Archives. Licence CC BY-NC 4.0.), and a model of the double helix DNA structure it would help solve (Science Museum Group Collection. © The Board of Trustees of the Science Museum. Licence CC BY-NC-SA 4.0.).
Another microbiologist, Arthur Kornberg at Washington University in St Louis isolated the enzyme DNA polymerase I in 1956. Meanwhile Jacques Monod and François Jacob at the Pasteur Institute in Paris demonstrated that regulatory genes control the expression of enzymes in bacteria.
By this time the first protein structures were on the way to being solved. In Perutz’s unit, the biochemist Vernon Ingram made haemoglobin crystals containing additional heavy atoms, a trick that Bernal had suggested in the 1930s to create informative differences in the diffraction images. John Kendrew developed the first programs for crystallographic computing and solved the structure of myoglobin at atomic resolution, as well as founding the Journal of Molecular Biology, which he edited for nearly 30 years. Perutz also solved the structure of haemoglobin (his 1959 wooden model is pictured top, © MRC Laboratory of Molecular Biology), and he and Kendrew shared the Nobel prize for chemistry in 1962.
The successes of the unit led the MRC to establish a new, purpose-built Laboratory of Molecular Biology (LMB) on the site of Addenbrooke’s Hospital south of Cambridge. It became a magnet for structural biologists, including Aaron Klug, John Finch, David Blow, Richard Perham, Thomas Steitz and Kyoshi Nagai.
The original LMB building, Cambridge, circa 1962, and John Kendrew (left) and Max Perutz with the 'forest of rods' model of myoglobin in the LMB model room. © MRC Laboratory of Molecular Biology.
David Phillips, then working under Lawrence Bragg at the Royal Institution in London, contributed to the myoglobin solution by working with Uli Arndt to develop the first diffractometers, which automated the collection of the diffraction data. He went on to solve the structure of a third protein, lysozyme, in 1965, afterwards moving to Oxford with his then student Louise Johnson. Hodgkin, who won her own Nobel in 1964 for her solutions of penicillin and vitamin B12, finally solved insulin in 1969 with the help of a team led by Guy Dodson.
Dorothy Hodgkin's model of insulin, c.1969. The white balls represent zinc, which was introduced to help solve the crystal structure. © The Board of Trustees of the Science Museum. Released under licence CC BY-NC-SA 4.0.
The establishment of the LMB in Cambridge made it possible to bring genetics and structural biology together. Fred Sanger, who had sequenced the protein insulin in the 1950s, went on to develop one of the first methods of sequencing DNA. Sydney Brenner formed a close working partnership with Francis Crick, contributing to the cracking of the genetic code, helping to discover messenger RNA, and later establishing the roundworm Caenorhabditis elegans as a model organism for geneticists. John Sulston joined the worm lab in the late 1960s and started a worm genome sequencing project.
Example of autoradiography X-ray film used in early DNA sequencing (Michele Studer, Wellcome Collection. CC BY 4.0). John Sulston in the laboratory with a roundworm background, c. 1985. © MRC Laboratory of Molecular Biology.
As the first director of the Wellcome Trust’s Sanger Centre (now Wellcome Sanger Institute), Sulston went on to lead the British contribution to the human genome project, which was completed in 2003.
All the memoirs discussed here are available to read in the new Structural and molecular biology special collection. All memoirs are published online as they are ready before being compiled into two volumes per year. Sign up for alerts or keep an eye on our website for new memoirs as they appear.