New research published in Journal of the Royal Society Interface examines how the unique muscle arrangement of seahorse tails affects the mechanics of grasping, using in-silico simulations. Dr Dries Marzougui from Ghent University provides an overview of their work.
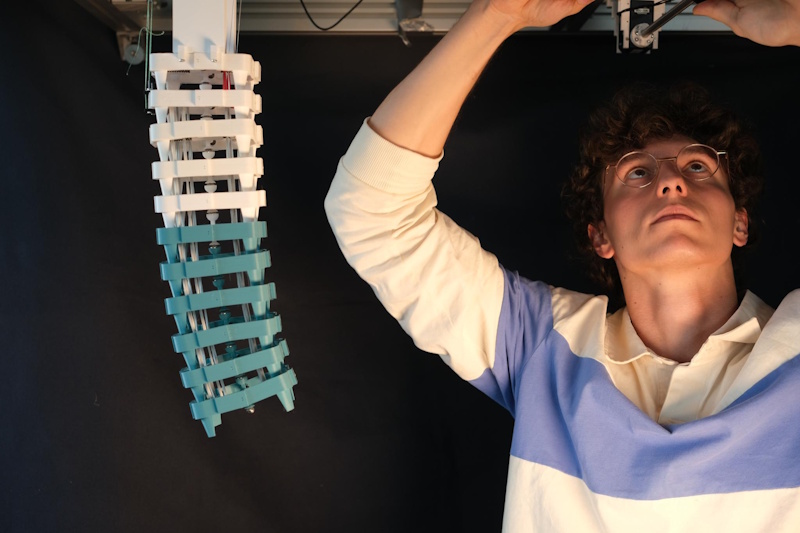
Please can you introduce your study and tell us what it’s about?
Seahorses have a distinctive tail muscle architecture that allows them to efficiently grasp and anchor onto objects. This prehensile ability is essential for their survival, enabling them to resist water currents, hold onto mates during reproduction, and remain camouflaged from predators. Unlike any other fish, seahorses possess long, parallel muscle sheets that can extend across up to eleven vertebral segments. This study examines how this unique muscle arrangement affects the mechanics of grasping. Using in-silico simulations validated by a 3D-printed prototype, we demonstrate the complementary roles of these elongated muscles alongside shorter, intersegmental muscles. Additionally, we show that muscles spanning more vertebrae generate greater contractile forces and enhance force-to-torque transmission. Our findings highlight the functional advantages of this specialized muscle-tendon structure, providing new insights into the evolutionary adaptations that shaped the seahorse’s remarkable prehensile tail.
What is the importance of taking a cross-disciplinary approach to your research?
A cross-disciplinary approach is essential when testable live data—such as seahorses with short hypaxial myosepta—are unavailable. Using biomimetic robotic models allows us to isolate specific anatomical traits, artificially modify them, and systematically analyse their functional performance in controlled conditions. This method allows us to examine structures both within and beyond the bounds of nature, vastly increasing the investigative scope. In doing so, we not only address core evolutionary questions, but also gain valuable engineering insights. Specifically, the mechanics of prehensile biological systems, such as the seahorse tail, can inspire new robotic designs that integrate strength and flexibility—a combination that is still missing in modern robotic manipulators.
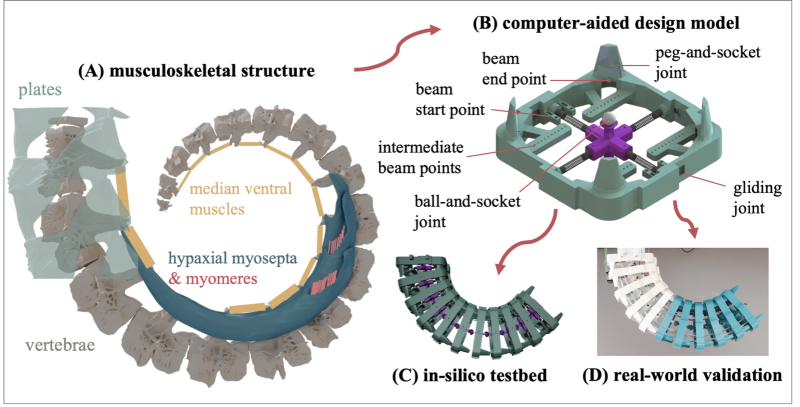
How was your experience publishing with J. R. Soc. Interface?
Publishing with J. R. Soc. Interface was a great experience. The journal strongly promotes interdisciplinary research, making it a perfect fit for our study, which bridges biology and robotics. The review process was both fast and constructive, with insightful feedback that helped refine our work. One aspect we particularly appreciated is the journal’s commitment to Open Science. By encouraging the sharing of data and materials, it fosters transparency and reproducibility, values that align closely with our research philosophy.
What are the next steps for this line of research?
Building on our findings, we are now developing seahorse tail-inspired robots that bridge the gap between rigid industrial robots and flexible soft robots. Traditional industrial robots excel in high-load tasks but lack flexibility, while soft robots can conform to objects but struggle with precision and payload capacity. The seahorse tail’s remarkable combination of bendability and rigidity, a result of its varying plate and vertebra size connected by flexible tissue, thereby serves as an interesting foundation for creating novel robotic grippers, arms, and spines that surpass current limitations.
At the level of adaptive evolution, our model enables us to further explore the functional implications of the transition from ancestral tail morphologies to the complex musculoskeletal architecture observed in seahorses. Similarly, we will investigate closely related taxa, such as pipehorses, to better understand the evolution and functional diversity of these prehensile tails.
Images:
Dries during the building/design process. Credit: Prof. dr. ir. Francis Wyffels.
Figure 1. Overview of our pipeline. (A) 3D reconstruction of a μCT scan of the seahorse tail (Hippocampus reidi) depicting its skeletal structure and ventral muscles. (B) The biomimetic transfer of a seahorse tail unit to a computer-aided design model, capturing the main skeletal geometries and incorporating the same primary joints. The routing points of the beams that mimic the ventral muscles are annotated. (C) The in-silico instantiation of our musculoskeletal model, which serves as our primary testbed due to its complete controllability and observability. (D) The real-world instantiation of our musculoskeletal model, which serves as a validation tool for our in-silico results. The prototype was 3D-printed in two colours of PLA (white and blue), based on availability.
Keep up to date with the latest issues of Journal of the Royal Society Interface by signing up for content alerts, and browse previous issues on the journal website.