Links to external sources may no longer work as intended. The content may not represent the latest thinking in this area or the Society’s current position on the topic.
Integrating Hebbian and homeostatic plasticity
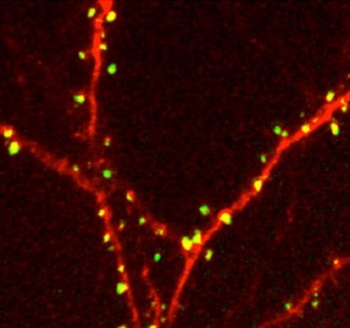
Scientific discussion meeting organised by Professor Kevin Fox FMedSci and Professor Michael Stryker.
This meeting brought together computational and experimental neuroscientists to discuss how interactions between Hebbian plasticity and homeostatic plasticity occur and how they can be detected and interrogated at the systems level.
Organiser and speaker biographies are available below, together with the schedule of talks and abstracts. Alternatively you can download the draft programme (PDF).
Position papers
Position papers are available to download below. Three of the speakers contributed their thoughts on the state of the field and the major issues to be addressed at the meeting. The position papers are provided by the speakers and the Royal Society takes no responsibility for their content.
-
Professor Wulfram Gerstner | Cooperation across timescales between and Hebbian and homeostatic plasticity (PDF)
-
Professor Gina Turrigiano | The Dialectics of Hebb and Homeostasis within Neural Circuits (PDF)
Attending this event
This meeting has taken place. Recorded audio of the presentations can be found below, and papers from the meeting will be published in a future issue of Philosophical Transactions B.
Enquiries: Contact the events team.
Organisers
Schedule
Chair
Professor John Lisman, Brandeis University, USA
Professor John Lisman, Brandeis University, USA
Professor John Lisman is a full professor at Brandeis University, where he has taught since 1974. He graduated cum laude in physics from Brandeis University, received his PhD in physiology from MIT, and was a postdoctoral fellow with Professor George Wald at Harvard University. He has received prestigious awards, most notably the Jacob Javits award from the National Institute of Health and American Association for the Advancement of Science (AAAS) Fellowship.
His laboratory is interested in the molecular basis of memory, particularly the processes that underlie the storage of memory. To study this problem his laboratory uses both theoretical and experimental approaches. The working hypothesis is that memory is stored by the complex of CaMKII with the NR2B subunit of the NMDAR. Importantly, dissociating this complex after LTP induction can reverse LTP, thus demonstrating its role LTP maintenance.
In a separate line of work, Lisman has investigated the mechanisms that underlie schizophrenia. A well-established symptom of this disease is enhanced EEG power in the delta frequency range. Lisman’s work points to a mechanism for generating delta in the thalamus, mechanism that involves T-type Ca channels and NR2C.
09:05 - 09:30 |
Spine-size fluctuations enable stable cell assembly learning in recurrent circuit models
Cortical circuits rewire in an experience-dependent way. A major biological mechanism underlying this is Hebbian plasticity. In models of recurrently connected networks, ongoing Hebbian plasticity is often unstable in nature because of its positive feedback, eg a tightly coupled and coherently active group of neurons tends to drive other neurons well and expand the group (Kunkel et al., 2011). This typically fuses multiple memory patterns and results in a deficiency in learning/memory performance. The biological mechanism that stabilises Hebbian plasticity is unknown. Here we combine experimentally observed fluctuations of spine sizes (Yasumatsu et al., 2008) with spike-timing-dependent plasticity in recurrently connected neural networks. We show that an appropriate level of spine fluctuations is sufficient to stabilise memory patterns without fusing, and maintains a physiological volume distribution of spines in the presence of ongoing Hebbian plasticity. In addition to stabilising Hebbian plasticity, we posit that abnormal spine fluctuations impair learning/memory performance. Our theory explains how high spine turnover rates, experimentally observed in several animal models for autism (Isshiki et al., 2014), cause slow learning and impairs memory performance. ![]() Dr Taro Toyoizumi, RIKEN Brain Science Institute, Japan
![]() Dr Taro Toyoizumi, RIKEN Brain Science Institute, JapanTaro Toyoizumi is a lab head at RIKEN Brain Science Institute. He received his BS from Tokyo Institute of Technology in 2001, and his MS and PhD from the University of Tokyo in 2003 and 2006, respectively. Toyoizumi studied at the Center for Theoretical Neuroscience at Columbia University as a JSPS and Patterson Trust Postdoctoral Fellow. He then came to RIKEN Brain Science Institute as a Special Postdoctoral Researcher in 2010, and was promoted to Lab Head in 2011. Toyoizumi received the International Neural Network Society, Young Investigator Award in 2008. |
|
---|---|---|
09:45 - 10:15 |
Homeostasis and assembly formation in spiking networks
Homeostatic mechanisms homogenise activity within spiking networks, ensuring that any potential for unstable network activity is corrected. However, strict homogeneity in a recurrent cortical circuit precludes any rich dynamics, as well as the computations they support. Thus, there is a tension between the need for stability and the desire for rich circuit structures that often flirt with instability. We explore this problem by studying how homeostasis through plasticity of inhibitory connections interacts with plasticity of excitatory connections during assembly formation in models of cortex. We show that homeostatic inhibition is essential for stabilising assembly formation both with rate-based and timing-based plasticity rules. The key requirement is that homeostatic mechanisms should not operate on the timescale of learning – for rate based excitatory plasticity the homeostatic mechanisms should be slower, for spike-timing based mechanisms homeostasis should be faster. ![]() Dr Brent Doiron, University of Pittsburgh, USA
![]() Dr Brent Doiron, University of Pittsburgh, USABrent Doiron received his PhD in physics from the University of Ottawa in 2004, and after a postdoctoral fellowship at New York University, he has been a professor in the mathematics department at the University of Pittsburgh since 2007. His work has focused on circuit models of sensory processing, ranging over visual, olfactory, somtaosensory, auditory and electrosensory systems. He has recently focused on the genesis and impact of neural variability on both stimulus representation and learning dynamics. |
|
11:00 - 11:30 |
Homeostasic control in recurrent networks
We present a systematic analysis of homeostatic control in networks of neurons. It reveals two important aspects of homeostatic control. First, we consider networks of neurons with homeostasis and show that homeostatic control that is stable for single neurons, can destabilise activity in otherwise stable recurrent networks. This instability can be prevented by dramatically slowing down the homeostatic control. Next, we consider the case that homeostatic feedback is mediated via a cascade of multiple intermediate stages. Counter-intuitively, the addition of extra stages in the homeostatic control loop further destabilises activity in single neurons and networks. We thus reveal previously unconsidered constraints on homeostasis in biological networks, and provide a possible explanation for the slow time-constants of homeostatic regulation observed experimentally. Dr Mark van Rossum, University of Edinburgh, UK
Dr Mark van Rossum, University of Edinburgh, UKMark van Rossum is a Reader in the School of Informatics at the University of Edinburgh and is director of Neuroinformatics Doctoral Training Centre. He has a PhD from the University of Amsterdam and has held research positions at the University of Pennsylvania and Brandeis University. His group has published on a wide range of research interest including neural plasticity, STDP, memory, homeostasis, visual coding, network modelling, biophysics, and novel data analysis tools. He has interdisciplinary research collaborations with physiologists, anatomists, psychologists and engineers from across the world. Mark is always interested in new collaborative projects with a computational component. |
|
11:45 - 12:15 |
Homeostatic control during Hebbian changes: a question of time scales
When looking at the interaction of homeostatic and Hebbian plasticity we make a puzzling observation: while homeostasis of synapses reported in experiments is slow, homeostasis of synapses in most computational models is rapid, or even instantaneous. Even worse, most existing plasticity models cannot maintain stability in simulated networks with the slow homeostatic plasticity reported in experiments. To solve this paradox, we suggest that there are both fast and slow forms of homeostatic plasticity with distinct functional roles. Theory predicts that there must be a fast form of synaptic homeostasis (on the time scale of seconds or minutes), in order to render Hebbian plasticity intrinsically stable. This fast form might be experimentally observable as heterogeneous synaptic plasticity. Furthermore, slower forms of homeostatic plasticity are important for fine-tuning neural circuits. Taken together we suggest that learning and memory relies on an intricate interplay of diverse plasticity mechanisms on different timescales which jointly ensure stability and plasticity of neural circuits. 1. F. Zenke, E.J. Agnes and W. Gerstner. 2015. Diverse synaptic plasticity mechanisms orchestrated to form and retrieve memories in spiking neural networks. Nature Communications 6, 6922. 2. F. Zenke, G. Hennequin and W. Gerstner. 2013. Synaptic Plasticity in Neural Networks Needs Homeostasis with a Fast Rate Detector. PLOS Computational Biology 9:e1003330 DOI:10.1371/journal.pcbi.1003330 ![]() Professor Wulfram Gerstner, École polytechnique fédérale de Lausanne, Switzerland
![]() Professor Wulfram Gerstner, École polytechnique fédérale de Lausanne, SwitzerlandWulfram Gerstner is Director of the Laboratory of Computational Neuroscience, LCN at the EPFL. He studied physics at the universities of Tubingen and Munich and received a PhD from the Technical University of Munich. His research in computational neuroscience concentrates on models of spiking neurons and spike-timing dependent plasticity, and on the problem of neuronal coding in single neurons and populations. He has a joint appointment at the School of Life Sciences and the School of Computer and Communications Sciences at the EPFL. He teaches courses for physicists, computer scientists, mathematicians, and life scientists. |
Chair
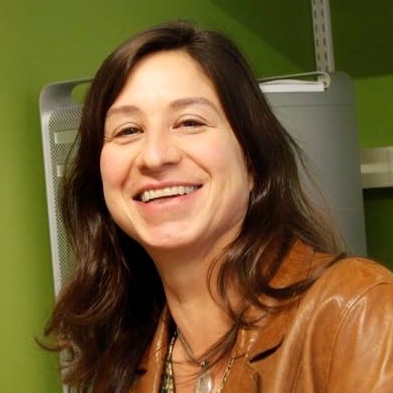
Professor Gina Turrigiano, Brandeis University, USA
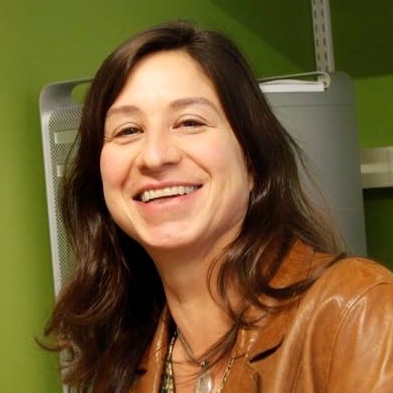
Professor Gina Turrigiano, Brandeis University, USA
Gina Turrigiano received her BA from Reed College in 1984 and her PhD from University of California San Diego in 1990. She then trained as a postdoc with Eve Marder at Brandeis University before joining the faculty in 1994. She is now a full professor in the Department of Biology, the Volen Center for Complex Systems, and the Center for Behavioral Genomics at Brandeis. She has received numerous awards for her research including a MacArthur foundation fellowship, an NIH director’s pioneer award, the HFSP Nakasone Award, and election to the American Academy of Arts and Sciences and the National Academy of Sciences. Her scientific interests include mechanisms of synaptic and intrinsic plasticity and the experience-dependent refinement of neocortical microcircuitry.
13:30 - 14:00 |
Cocaine-induced synaptic plasticity in the striatum: Hebbian and homeostatic mechanisms
Drugs of abuse, such as cocaine, induce changes in reward circuitry, which manifest as long lasting changes in behavior. The synaptic changes are likely due to a combination of both Hebbian and homeostatic types of plasticity. As the pro-inflammatory cytokine tumor necrosis factor alpha (TNF) is required for some forms of homeostatic plasticity in the cortex and hippocampus but is not required for Hebbian forms of plasticity, we tested the TNF-dependence of cocaine-induced striatal plasticity. Repeated administration of cocaine results in an initial weakening of glutamatergic synapses in the nucleus accumbens, followed by a synaptic strengthening during abstinence from drug. We show that this biphasic plasticity is comprised of two parts. Cocaine substantially elevates dopamine, which directly potentiates synapses on D1-expressing accumbens neurons. However, cocaine also activates microglia in the nucleus accumbens and increases TNF production. TNF acts to depress synapses preferentially on D1-expressing neurons in the accumbens, antagonizing cocaine-induced synaptic plasticity and reducing behavioural sensitization. During abstinence, microglia de-activate and TNF levels drop, revealing the underlying potentiation. Importantly, a weak TLR4 agonist can re-activate microglia, increase TNF production, depress synaptic strength in the accumbens, and suppress cocaine-induced sensitization. Thus, microglia act adaptively to maintain circuit function in the face of cocaine-induced disruption, and suggests this is a homeostatic response to aberrant Hebbian-type plasticity induced by cocaine. ![]() Dr David Stellwagen, McGill University, Canada
![]() Dr David Stellwagen, McGill University, CanadaDavid Stellwagen PhD is an Associate Professor in the Department of Neurology and Neurosurgery at McGill University, and a member of the Centre for Research in Neuroscience. He studied Neuroscience at Brown University, working with Dr Mark Bear, and received a PhD from the University of California, Berkeley, under the supervision of Dr Carla Shatz. He received post-doctoral training with Dr Rob Malenka, at the Stanford Medical School, where they first described the pro-inflammatory cytokine tumor necrosis factor alpha (TNF) as a critical mediator of homeostatic plasticity. His lab investigates the role of this form of synaptic plasticity in both normal brain function and during various disease states, including neurodegenerative disease, addiction, and developmental disorders such as autism. |
|
---|---|---|
14:15 - 14:45 |
Cholinergic modulation of NMDA receptor function mediated through an astrocyte intermediate
A transformation in thinking about plasticity occurred a quarter of a century ago when it was demonstrated that astrocytes release chemical transmitters that can modulate synaptic transmission and plasticity. In this presentation I will summarise this work as well as highlight recent studies which have shown the importance of astrocyte derived D-serine in the control of NMDA receptor function and, as a consequence, learning and memory. While synaptic plasticity is an essential feature of pre and postsynaptic neurons, through gliotransmission at the tripartite synapse astrocytes tune the synaptic system for effective scaling of the magnitude of the plastic event. ![]() Professor Philip Haydon, Tufts University School of Medicine, USA
![]() Professor Philip Haydon, Tufts University School of Medicine, USAPhilip G. Haydon PhD is the Annetta and Gustav Grisard Professor and Chair of Neuroscience at TUSM. For twenty-five years his research has focused on roles played by glial cells in the modulation of neurons and recently into the use of glial targets as therapeutic interventions for brain disorders. He graduated from the Department of Physiology, University of Leeds, UK with a BSc (Hons) and PhD in 1979 and 1982, respectively and then moved to the USA for postdoctoral studies. He obtained his first faculty position at Iowa State University where his studies of glial-neuron interactions commenced in 1991. In 1994 he published the first paper demonstrating the release of gliotransmitters and demonstrated that astrocytes could modulate neuronal activity through the release of the excitatory transmitter glutamate. In 1999 he coined the concept of ‘The Tripartite Synapse’ to recognise the important role played by glia in the modulation of synaptic transmission. Subsequently, while at the University of Pennsylvania School of Medicine, he integrated molecular genetics into his studies and demonstrated the importance of glial cells in the control of sleep. Since moving to Tufts University School of Medicine in 2008 his research has focused on the importance of glial cells in Alzheimer’s disease. Through a Tufts University spin-off company, GliaCure, Inc., he has successfully moved his preclinical studies into clinical trials for Alzheimer’s disease. He has received prestigious awards including an Alfred P. Sloan Scholarship, the McKnight Innovator Award and the Jacob Javits Award from the NINDS of the NIH. |
|
15:30 - 16:00 |
Synaptic signalling of retinoic acid
A neuron’s ability to change its responsiveness to synaptic inputs based on prior experience is an essential feature of the nervous system. The known forms of long-term synaptic plasticity can be grossly divided into two main categories: Hebbian and homeostatic. Our work established a critical role of retinoic acid (RA) in a form of homeostatic synaptic plasticity that is induced by prolonged reduction in synaptic excitation. Acting through a distinct molecular mechanism, RA is capable of rapidly changing excitatory as well as inhibitory synaptic strength. One of the main open questions is whether and how homeostatic synaptic plasticity intersects with Hebbian synaptic plasticity, and how such interaction impacts animal learning. In this talk, I will describe a recent study in which we examined the impact of RA-dependent synaptic signalling on Hebbian plasticity and hippocampal-dependent learning. Exposure of adult animals to an enriched environment (EE) engaged hippocampal RA signalling, which altered excitatory synaptic strength in the hippocampal CA1 neurons. Unexpectedly, although EE exposure did not alter LTP magnitude in WT animals, it significantly enhanced LTP in RARalpha KO hippocampus. By contrast, EE exposure significantly enhanced LTD in WT hippocampus, and such enhancement was prevented by RARalpha deletion. We also examined the behavioural consequences of altered Hebbian plasticity and found that hippocampal-dependent learning was altered in EE-exposed RARalpha knockout animals. Taken together, our study for the first time established an in vivo function of synaptic RA signalling in adult animals and demonstrated that RA-dependent experience-induced synaptic modification acts as a form of meta-plasticity to impact Hebbian plasticity and learning. ![]() Dr Lu Chen, Stanford University, USA
![]() Dr Lu Chen, Stanford University, USADr Lu Chen obtained her PhD in the Department of Biological Sciences at the University of Southern California. She is currently an associate professor in the Department of Neurosurgery and Department of Psychiatry and Behavioral Science at Stanford University School of Medicine. Dr Chen’s main research interest is to understand the cellular and molecular mechanisms that underlie synapse function during behaviour in the developing and mature brain, and how synapse function is altered during mental retardation. Recent work from her lab focuses on understanding synaptic signalling mechanisms involved in homeostatic synaptic plasticity, and the behavioural consequences of functional interactions between homeostatic and Hebbian synaptic plasticity. Additionally, she is investigating synaptic dysfunction in animal models of psychiatric disorders (i.e. fragile-X syndrome). |
|
16:15 - 16:45 |
Homeostatic plasticity of inhibition
Inhibitory synaptic transmission is critical for normal cortical functions. In sensory cortices, changes in the functional connectivity between inhibitory interneurons and pyramidal cells dictate the maturation of cortical function in tune with the environment. In order for inhibitory network to provide appropriate level of control over neural activity, it is critical that they undergo homeostatic regulation depending on the demand of the cortical circuitry. We found that homeostatic regulation of inhibitory synapses occurs via two distinct mechanisms during development. In immature circuits, inhibitory synapses adapt to overall changes in sensory experience by postsynaptic mechanisms. In contrast, in mature circuits we uncovered a novel mechanism that allows selectively control of action-potential independent release without changes in evoked inhibition. Such adaptation endows the mature cortical circuit to provide homeostatic control of overall neural activity without compromising information coding capabilities. ![]() Dr Hey-Kyoung Lee, Johns Hopkins University, USA
![]() Dr Hey-Kyoung Lee, Johns Hopkins University, USADr Hey-Kyoung Lee received her BS in Biology from Yonsei University (Korea) in 1992. In 1997, she completed her graduate studies under the mentorship of Dr Mark Bear, and received a PhD in Neuroscience from Brown University (USA). Her thesis work focused on the cellular and molecular mechanisms of bidirectional synaptic plasticity. Dr Lee received postdoctoral training in Neuroscience with Dr Richard Huganir at the Johns Hopkins School of Medicine (USA), where she studied the role of AMPA receptor phosphorylation in synaptic plasticity. In 2003, Dr Lee joined the University of Maryland College Park as an Assistant Professor of Biology. She was awarded the Sloan Fellowship in 2004, and was nominated as one of the ‘Yonsei 100 Women Leaders’ in 2006. In 2009, she was awarded the Junior Faculty Award from the College of Chemical and Life Sciences, and received tenure from the University of Maryland and promoted to Associate Professor. Dr Lee joined the Department of Neuroscience at Johns Hopkins University in 2011, and was promoted to tenured Professor in 2015. |
Chair
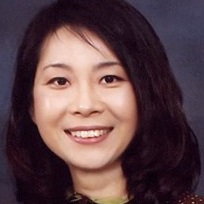
Dr Hey-Kyoung Lee, Johns Hopkins University, USA
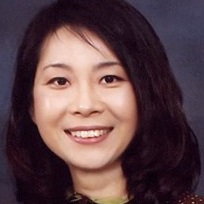
Dr Hey-Kyoung Lee, Johns Hopkins University, USA
Dr Hey-Kyoung Lee received her BS in Biology from Yonsei University (Korea) in 1992. In 1997, she completed her graduate studies under the mentorship of Dr Mark Bear, and received a PhD in Neuroscience from Brown University (USA). Her thesis work focused on the cellular and molecular mechanisms of bidirectional synaptic plasticity. Dr Lee received postdoctoral training in Neuroscience with Dr Richard Huganir at the Johns Hopkins School of Medicine (USA), where she studied the role of AMPA receptor phosphorylation in synaptic plasticity. In 2003, Dr Lee joined the University of Maryland College Park as an Assistant Professor of Biology. She was awarded the Sloan Fellowship in 2004, and was nominated as one of the ‘Yonsei 100 Women Leaders’ in 2006. In 2009, she was awarded the Junior Faculty Award from the College of Chemical and Life Sciences, and received tenure from the University of Maryland and promoted to Associate Professor. Dr Lee joined the Department of Neuroscience at Johns Hopkins University in 2011, and was promoted to tenured Professor in 2015.
09:05 - 09:30 |
Firing rate homeostasis is gated by sleep/wake states
Homeostatic mechanisms stabilise neural circuit function by keeping firing rates (FRs) within a set-point range, but whether individual neocortical neurons regulate firing around a cell-autonomous set-point, and whether this process is restricted to certain behavioural states such as sleep or wake, is unknown. I will start by discussing the mechanisms of synaptic scaling, a form of cell-autonomous homeostatic plasticity, and the role of this plasticity in generating firing rate set-points in vivo. I will then discuss new work in which we follow the process of FR homeostasis in individual visual cortical neurons in freely behaving rodents as they cycled between sleep and wake states. When FRs are perturbed by visual deprivation, over time they returned precisely to a cell-autonomous set-point, and this restoration of firing occurred selectively during periods of active waking and was suppressed by sleep. Longer natural waking periods result in more FR homeostasis, as does artificially extending the length of waking. This exclusion of FR homeostasis from sleep raises the possibility that memory consolidation or some other sleep-dependent process is vulnerable to interference from homeostatic plasticity mechanisms. ![]() Professor Gina Turrigiano, Brandeis University, USA
![]() Professor Gina Turrigiano, Brandeis University, USAGina Turrigiano received her BA from Reed College in 1984 and her PhD from University of California San Diego in 1990. She then trained as a postdoc with Eve Marder at Brandeis University before joining the faculty in 1994. She is now a full professor in the Department of Biology, the Volen Center for Complex Systems, and the Center for Behavioral Genomics at Brandeis. She has received numerous awards for her research including a MacArthur foundation fellowship, an NIH director’s pioneer award, the HFSP Nakasone Award, and election to the American Academy of Arts and Sciences and the National Academy of Sciences. Her scientific interests include mechanisms of synaptic and intrinsic plasticity and the experience-dependent refinement of neocortical microcircuitry. |
|
---|---|---|
09:45 - 10:10 |
Hebbian and homeostatic plasticity and the role of TNF-α in the visual cortex
![]() Professor Michael Stryker, University of California, San Francisco, USA
![]() Professor Michael Stryker, University of California, San Francisco, USAMichael Stryker studied at Deep Springs College and the University of Michigan and did research in the laboratory of James Olds. After two years in East Africa as a hydraulics engineer with the Peace Corps, he entered Peter Schiller's laboratory at MIT for PhD studies, followed by a postdoctoral fellowship with David Hubel and Torsten Wiesel at the Harvard Medical School. He joined the Physiology Department and the nascent neuroscience programme at UCSF as an assistant professor in 1978, where he served as department chair for 12 years and has remained except for sabbaticals at Oxford and as the Galileo Professor of Science at Esculoa Normale Superiore in Pisa. He has also served as Co-director of the UCSF Neuroscience Program in and as Director of the Boyer Program in Biological Science overseeing all the UCSF basic science graduate programs. He holds the W.F. Ganong Chair of Physiology at UCSF and has been honoured by election to the American Academy of Arts and Sciences and the US National Academy of Sciences and by the W. Alden Spencer Award and the Pepose Award in Vision Science. |
|
10:50 - 11:15 |
Diversity of homeostatic and Hebbian plasticity properties in cortical neurones
Not all neurones are created equal when it comes to plasticity. Either by virtue of their different afferent pathways or their different synaptic receptors, neurones in different layers of the cerebral cortex show different degrees and types of synaptic plasticity. For principal neurons in the adult somatosensory cortex, layer 4 is relatively aplastic while layer 2/3 and layer 5 are highly plastic. Recent studies show that the diversity does not end there. The two major subdivisions of layer 5 pyramidal cells, regular spiking (RS) and intrinsic bursting (IB) cells also show different types of plasticity and rely on Hebbian and homeostatic mechanisms to different degrees. RS neurones show experience-dependent depression following whisker trimming, which slowly recovers homeostatically back to baseline despite the maintained deprivation. The homeostatic rebound is TNFa dependent. Potentiation of spared whisker responses is absent in these cells. In contrast, IB cells do show potentiation of spared whisker responses comprising both TNF a and a-CaMKII-autophosphorylation dependent components1. As LTP is absent in aCaMKII-autophosphorylation mutants2 and synaptic upscaling is absent in TNF a knockouts3, these findings suggest the two synaptic mechanisms are distributed differently between the two cell types. In the light of these results, we looked again at plasticity in layer 2/3. Previous studies had shown that LTP and experience-dependent potentiation are absent in the barrel cortex of aCaMKII-autophosphorylation mutants2. Our present studies show that in addition, following depression of deprived whisker responses, layer 2/3 cells show a homeostatic rebound that is prevented by a soluble TNF a scavenger. In summary, all three cortical cell types show varying degrees of TNF a dependent homeostatic plasticity, but, while layer 2/3 cells show both Hebbian depression and potentiation, in layer 5 Hebbian depression and potentiation are segregated between RS and IB cells respectively. 1. S. D. Greenhill et al. 2015. Neuron 88, 539-552. 2. N. Hardingham et al. 2003.J Neurosci 23, 4428-4436. 3. D. Stellwagen, R. C Malenka. 2006. Nature 440, 1054-1059. ![]() Professor Kevin Fox FMedSci, Cardiff University, UK
![]() Professor Kevin Fox FMedSci, Cardiff University, UKKevin Fox PhD MAE FMedSci is a Professor of Neuroscience and a member of the School of Biosciences and the Neuroscience and Mental Health Research Institute at Cardiff University. He studied Electrical Engineering at the University of Bath before receiving his PhD in Neurophysiology from the University of London. His research programme is funded by the Medical Research Council in the UK. His lab is interested in the systems, cellular and molecular mechanisms of cortical plasticity. Work in the lab has focused on the visual and somatosensory cortex as model systems for understanding plasticity. Current research addresses structural plasticity mechanisms in layer 2/3 neurons and functional plasticity in layer 5 neurons, particularly the differences inherent in Regular spiking and Intrinsic bursting cells of cortical layer 5. The overall aim of his lab is to understand plasticity to the level where it can be safely manipulated for therapeutic benefit. |
|
11:30 - 11:55 |
Rapid homeostasis by control of inhibition
Cortical plasticity involves both Hebbian mechanisms that alter neural tuning in response to experience, and multiple homeostatic mechanisms that maintain cortical firing rates within a stable operating regime. We have investigated the circuit and cellular mechanisms for homeostasis in response to brief sensory manipulations in whisker somatosensory cortex (S1). Whisker deprivation induces a rapid homeostatic increase in local circuit excitability in L2/3 of S1 that preserves sensory-evoked spike rates despite loss of excitatory synaptic input. This occurs within 1 day of deprivation, and precedes classical changes in receptive fields and maps. These changes do not involve synaptic scaling, but instead reflect a preferential reduction in whisker-evoked inhibition in L2/3 pyramidal cells, due to reduced activation of parvalbumin (PV) interneuron circuits. Deprivation-induced disinhibition occurs in both feedforward and recurrent inhibitory networks. Sustained deprivation drives disinhibition by reducing excitatory synaptic input to L2/3 PV neurons. In contrast, brief deprivation drives rapid disinhibition by reducing intrinsic excitability of PV neurons. This is evident in elevated spike threshold, increased spike width and damped near-threshold excitability. These findings add to a growing body of evidence that PV interneurons are a critical nexus for homeostatic plasticity in sensory cortex. This single site of plasticity in the cortical microcircuit can control average firing rate in local cortical networks, regulate sensory gain, and gate subsequent Hebbian plasticity for reorganization of the whisker map. ![]() Dr Daniel Feldman, University of California, Berkeley, USA
![]() Dr Daniel Feldman, University of California, Berkeley, USADan Feldman is an Associate Professor of Neurobiology at UC Berkeley, in the Department of Molecular & Cell Biology, and the Helen Wills Neuroscience Institute. His laboratory studies neural coding, circuit function, and plasticity in cerebral cortex, using the whisker somatosensory system in rodents as a model system. He also studies neural circuit dysfunction in autism. He is Director of the Neuroscience PhD programme at Berkeley. |
|
12:10 - 12:35 |
Homeostatic mechanisms in the mouse visual cortex in vivo
Homeostatic synaptic scaling is thought to occur cell-wide. We used repeated in vivo two-photon imaging in mouse visual cortex after sensory deprivation to investigate the spatial extent of synaptic scaling. We used increases in spine size as a proxy for synaptic scaling in vivo in both excitatory and inhibitory neurons and found that after sensory deprivation, increases in spine size are restricted to a subset of dendritic branches, which we confirmed using immunohistochemistry. We found that the branches that had increases in spine size also had a lower spine density. Within a given dendritic branch, the degree of spine size increases was proportional to recent spine loss within that branch. Using simulations, we showed that this compartmentalised form of synaptic scaling better retained the previously established input-output relationship in the cell. ![]() Dr Tara Keck, University College London, UK
![]() Dr Tara Keck, University College London, UKTara Keck received a BA in engineering at Harvard University and a PhD in biomedical engineering at Boston University, where Keck studied the role of inhibition in the hippocampus with John White. Keck then did postdoctoral work with Tobias Bonhoeffer and Mark Hübener at the Max Planck Institute of Neurobiology in Munich, Germany, where she studied the role of structural plasticity in the remapping of receptive fields in the visual cortex. In 2010, Keck moved to the MRC Centre for Developmental Neurobiology as an MRC Career Development Fellow, and then moved her lab to the Department of Neuroscience, Physiology and Pharmacology at University College London in 2014. Keck’s lab studies the mechanisms of homeostatic plasticity in vivo. |
Chair
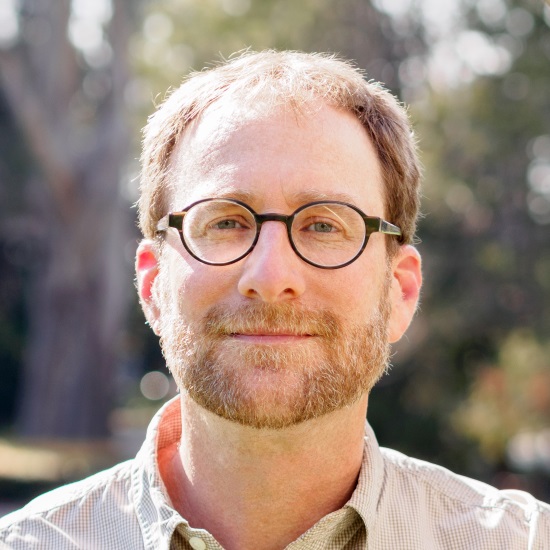
Dr Daniel Feldman, University of California, Berkeley, USA
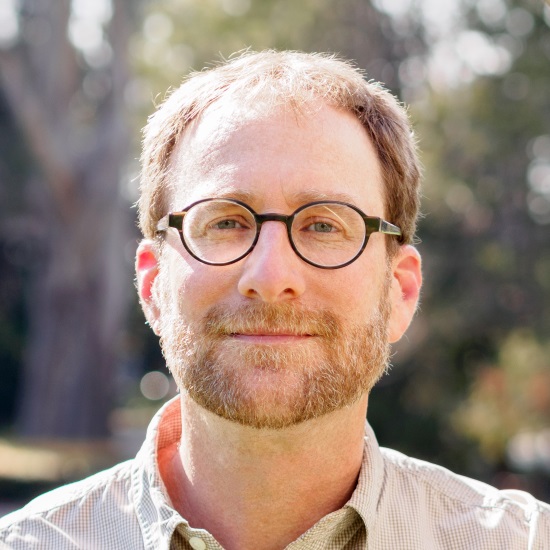
Dr Daniel Feldman, University of California, Berkeley, USA
Dan Feldman is an Associate Professor of Neurobiology at UC Berkeley, in the Department of Molecular & Cell Biology, and the Helen Wills Neuroscience Institute. His laboratory studies neural coding, circuit function, and plasticity in cerebral cortex, using the whisker somatosensory system in rodents as a model system. He also studies neural circuit dysfunction in autism. He is Director of the Neuroscience PhD programme at Berkeley.
Professor Mark Hübener, Max Plank Institute of Neurobiology, Germany
Professor Mark Hübener, Max Plank Institute of Neurobiology, Germany
Mark Hübener heads the ‘Visual System Plasticity’ lab in the Department Synapses – Circuits – Plasticity at the Max Planck Institute of Neurobiology in Martinsried, Germany. He is also Affiliate Professor of Neurobiology at the Ludwig Maximilians University of Munich. After studying Biology in Tübingen and Hamburg, he carried out his PhD work with Jürgen Bolz on structure-function relationships in the visual cortex at the Miescher Lab of the Max Planck Society in Tübingen. In 1993 he joined the Department of Tobias Bonhoeffer at the Max Planck Institute of Neurobiology, where he has been a group leader since 2002. He has made important contributions to our understanding of the diversity of morphological cell types in the visual cortex. His group has pioneered functional imaging from mouse visual cortex and superior colliculus employing intrinsic as well as two-photon calcium imaging. His main interests are the functional and structural determinants of plasticity in the visual system.
13:50 - 14:15 |
LTP, STP, and scaling: electrophysiological, biochemical, and structural mechanisms
Synapses are complex because they perform multiple functions, including at least six mechanistically different forms of plasticity (STP, early LTP, late LTP, LTD, distance-dependent scaling, and homeostatic scaling). The ultimate goal of neuroscience is to provide an electrophysiologically, biochemically, and structurally specific explanation of the underlying mechanisms. This review summarises the still limited progress towards this goal. Several areas of particular progress will be highlighted: 1) STP, a Hebbian process that requires small amounts of synaptic input, appears to make strong contributions to some forms of working memory; 2) The rules for LTP induction in the stratum radiatum of the hippocampus have been clarified: induction does not depend obligatorily on backpropagating Na spikes but, rather, on dendritic branch-specific NMDA spikes. Thus, computational models based on STDP need to be modified; 3) Late LTP, a process that requires a dopamine signal (neoHebbian), is mediated by trans-synaptic growth of the synapse, a growth that occurs about an hour after LTP induction; 4) There is no firm evidence for cell-autonomous homeostatic synaptic scaling; rather, homeostasis is likely to depend on a) cell-autonomous processes that are not scaling, b) synaptic scaling that is not cell autonomous but instead depends on population activity, or c) metaplasticity processes that change the propensity of LTP vs LTD; 5) The evidence for distance-dependent scaling along the primary dendrite is now firm, and a plausible structural-based mechanism is suggested; 6) Recent super-resolution studies indicate that glutamatergic synapses are modular (module size 70-80nm), as predicted by theoretical work. Modules are trans-synaptic structures and have high concentrations of PSD-95 and AMPAR. These modules function as quasi-independent loci of AMPA-mediated transmission and can probably be independently modified during plasticity (eg, some modules may be silent but become functional after LTP induction). These new discoveries open the door for understanding the structure/function relationships that underlie the multiple forms of synaptic plasticity at individual synapses. Professor John Lisman, Brandeis University, USA
Professor John Lisman, Brandeis University, USAProfessor John Lisman is a full professor at Brandeis University, where he has taught since 1974. He graduated cum laude in physics from Brandeis University, received his PhD in physiology from MIT, and was a postdoctoral fellow with Professor George Wald at Harvard University. He has received prestigious awards, most notably the Jacob Javits award from the National Institute of Health and American Association for the Advancement of Science (AAAS) Fellowship. His laboratory is interested in the molecular basis of memory, particularly the processes that underlie the storage of memory. To study this problem his laboratory uses both theoretical and experimental approaches. The working hypothesis is that memory is stored by the complex of CaMKII with the NR2B subunit of the NMDAR. Importantly, dissociating this complex after LTP induction can reverse LTP, thus demonstrating its role LTP maintenance. In a separate line of work, Lisman has investigated the mechanisms that underlie schizophrenia. A well-established symptom of this disease is enhanced EEG power in the delta frequency range. Lisman’s work points to a mechanism for generating delta in the thalamus, mechanism that involves T-type Ca channels and NR2C. |
|
---|---|---|
14:30 - 14:55 |
Effects of dark exposure on mouse visual cortex plasticity
Changes in the excitatory-inhibitory (E/I) balance, mediated by maturation of parvalbumin positive (PV) GABAergic interneurons, have been identified as a key factor controlling the critical period of experience-dependent plasticity. Interventions that slow PV cell maturation, such as dark rearing, also delay the time course of the critical period. In both rats and cats brief dark exposure (DE) later in life can restore plasticity and enable recovery of vision through a previously deprived eye. We studied the effects of DE on ocular dominance and single-cell responses in the binocular zone of mouse V1, during recovery from long-term monocular deprivation. We employed chronic intrinsic signal imaging and two-photon calcium imaging. We also examined the density of PV neurons and of perineuronal nets (PNNs), a known structural brake on plasticity. After reopening of the deprived eye one group was placed in a dark room for 7 days and subsequently transferred to standard cages in a 12h day/night cycle while the other group was placed immediately in standard cages. Animals were imaged 5 times at weekly intervals. In terms of ocular dominance, mice that had experienced DE exhibited more rapid recovery (within 1 week) than those that had, not but the end points were not significantly different. Single cells calcium signals revealed greater recovery of orientation selectivity after DE. The proportion of PV cells surrounded by PNNs was smaller in mice that had experienced DE. Our results show that DE boosts experience-dependent plasticity by restoring the visual cortex to a more juvenile-like state. Professor Frank Sengpiel, Cardiff University, UK
Professor Frank Sengpiel, Cardiff University, UKFrank Sengpiel studied Biology at the Ruhr University in Bochum, Germany. A final-year project under the supervision of Klaus-Peter Hoffmann started him off in visual neuroscience. He went to Oxford to study for a DPhil under the supervision of Colin Blakemore which he completed in 1994. His thesis was on ‘Mechanisms of binocular integration in the mammalian primary visual cortex’ and he has maintained an interest in this topic ever since. Frank Sengpiel spent 3 years as a research fellow at Magdalen College, Oxford, before moving back to Germany for a post-doc in the lab of Tobias Bonhoeffer at the Max Planck Institute of Neurobiology. He returned to the UK in 2000 to take up senior lectureship at Cardiff University where he was promoted to a personal chair in 2007. He is currently Head of the Neuroscience Division in the School of Biosciences. |
|
15:30 - 15:55 |
Cell-specific restoration of stimulus preference after monocular deprivation in visual vortex
Monocular deprivation (MD) evokes a prominent shift of neuronal responses in the visual cortex towards the open eye, accompanied by functional and structural synaptic rearrangements. This shift is reversible, but it is unknown whether the recovery happens at the level of individual neurons or whether it reflects a population effect. We used ratiometric Ca2+ imaging to follow the activity of the same excitatory layer 2/3 neurons in mouse visual cortex over months during repeated episodes of ocular dominance (OD) plasticity. We observed robust shifts towards the open eye in most neurons. Nevertheless, these cells faithfully returned to their pre-deprivation OD during binocular recovery. Moreover, the initial network correlation structure was largely recovered, suggesting that functional connectivity may be regained despite prominent experience-dependent plasticity. ![]() Dr Tobias Rose, Max Plank Institute for Neurobiology, Germany
![]() Dr Tobias Rose, Max Plank Institute for Neurobiology, GermanyTobias Rose leads the project group ‘Structural and Functional Plasticity of Individual Synapses’ in the department ‘Synapses – Circuits – Plasticity’ at the Max Planck Institute of Neurobiology in Martinsried, Germany. He is also a principal investigator in the collaborative research center ‘Assembly and Function of Neuronal Circuits’. For his PhD (2003-2006) he studied the biophysics of regulated exocytosis of endocrine cells at the European Neuroscience Institute, Göttingen. As a postdoc he then worked together with Thomas Oertner at the Friedrich Miescher Institute in Basel, Switzerland, where he explored the developmental aspects of synaptic vesicle turnover in intact tissue using newly designed tools. In 2010 he joined the department of Tobias Bonhoeffer. Together with three graduate students in his project group he develops new optophysiological chronic imaging approaches to study network stability and the relevance of structural synaptic rearrangements during experience-dependent plasticity both in vivo and in vitro. |
|
16:10 - 17:00 |
General summary and discussion
![]() Dr Tara Keck, University College London, UK
![]() Dr Tara Keck, University College London, UKTara Keck received a BA in engineering at Harvard University and a PhD in biomedical engineering at Boston University, where Keck studied the role of inhibition in the hippocampus with John White. Keck then did postdoctoral work with Tobias Bonhoeffer and Mark Hübener at the Max Planck Institute of Neurobiology in Munich, Germany, where she studied the role of structural plasticity in the remapping of receptive fields in the visual cortex. In 2010, Keck moved to the MRC Centre for Developmental Neurobiology as an MRC Career Development Fellow, and then moved her lab to the Department of Neuroscience, Physiology and Pharmacology at University College London in 2014. Keck’s lab studies the mechanisms of homeostatic plasticity in vivo. ![]() Dr Taro Toyoizumi, RIKEN Brain Science Institute, Japan
![]() Dr Taro Toyoizumi, RIKEN Brain Science Institute, JapanTaro Toyoizumi is a lab head at RIKEN Brain Science Institute. He received his BS from Tokyo Institute of Technology in 2001, and his MS and PhD from the University of Tokyo in 2003 and 2006, respectively. Toyoizumi studied at the Center for Theoretical Neuroscience at Columbia University as a JSPS and Patterson Trust Postdoctoral Fellow. He then came to RIKEN Brain Science Institute as a Special Postdoctoral Researcher in 2010, and was promoted to Lab Head in 2011. Toyoizumi received the International Neural Network Society, Young Investigator Award in 2008. |