Links to external sources may no longer work as intended. The content may not represent the latest thinking in this area or the Society’s current position on the topic.
Nucleation: past and future challenges for experiment, theory and simulation
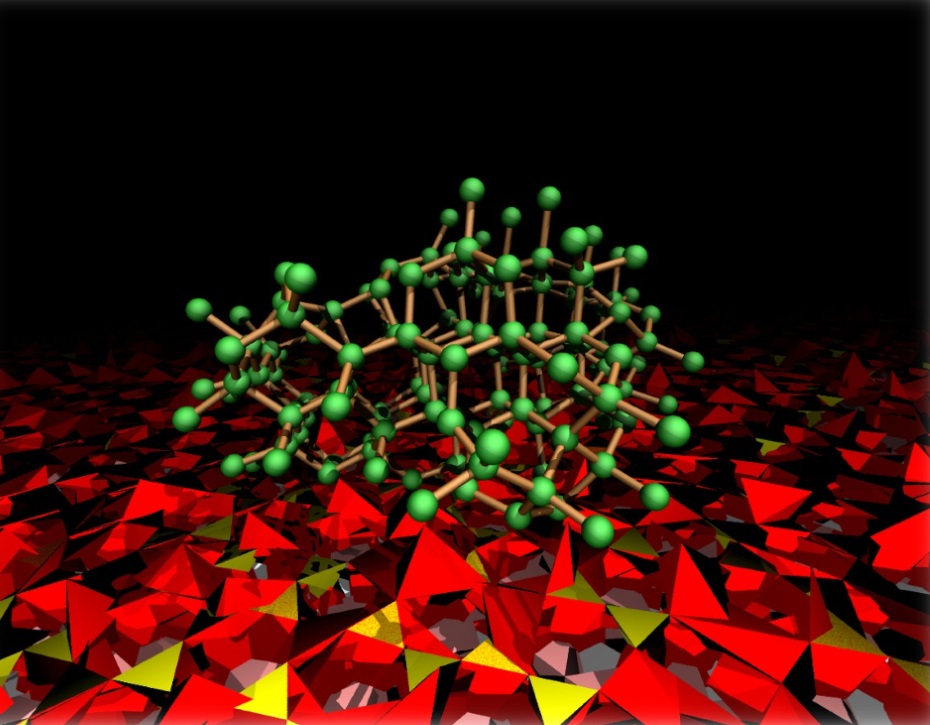
Theo Murphy international scientific meeting organised by Professor Angelos Michaelides, Professor Daan Frenkel ForMemRS, Professor Fiona Meldrum and Dr Gabriele Cesare Sosso.
Nucleation is a fundamental process crucial to a surprising array of different technological and everyday phenomena, from drug design to the formation of clouds.
This meeting brought together the key players in the field, taking stock of the latest developments in experiment and simulation as well as addressing the most pressing challenges to be faced in the near future.
Speaker biographies and abstracts are available below.
Attending this event
This meeting has taken place. Recorded audio of the presentations will be added shortly.
Enquiries: contact the scientific programmes team
Schedule
Chair
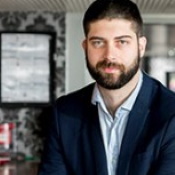
Dr Gabriele C Sosso, University of Warwick, UK
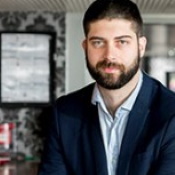
Dr Gabriele C Sosso, University of Warwick, UK
Gabriele C Sosso is an Associate Professor in Computational Physical Chemistry at the University of Warwick. He obtained his PhD in Nanostructures and Nanotechnologies from the University of Milano-Bicocca (Italy) in 2012, under the supervision of Professor Marco Bernasconi. He has worked as a PostDoctoral Research Associate with Professor Michele Parrinello at ETH Zürich and with Professor Angelos Michaelides at UCL. Gabriele is a computational scientist, chiefly interested in the physical chemistry of condensed matter, from supercooled liquids to biological interfaces. His vision is to understand the functional properties and the phase transitions of different classes of systems, from metallic glasses to cellular membranes. His approach consists in performing fundamental research using computer simulations, aimed at rationalise, complement and guide experiments and applications. Particularly relevant in the context of this meeting is Gabriele’s work on crystal nucleation and growth, which encompasses large-scale, machine learning-based simulations of semiconducting materials as well as enhanced sampling simulations of water freezing into ice at complex interfaces.
09:05 - 09:50 |
The structural and energetic sources of hierarchical nucleation pathways
Field and laboratory observations show that crystals commonly form by the attachment of particles that range from multi-ion complexes to fully formed nanoparticles. These hierarchical pathways are diverse, in contrast to those of classical models that consider only the addition of monomeric chemical species. Despite their complexity, a holistic framework for understanding particle-based pathways to crystallisation that extends classical concepts emerges when the coupled effects of complexity of free energy landscapes and the impact of dynamical factors that govern particle formation and interaction are considered. Here I describe that framework and use a series of in situ TEM and AFM studies on inorganic, organic, and macromolecular systems to illustrate the evolution in nucleation and growth processes as these complexities and dynamical factors come into play. The introduction of either size-dependent phase stability associated with the high surface-to-volume ratios of nanoparticles, or high driving force coupled with the existence of metastable polymorphs leads to true two-step pathways characterized by the initial appearance of a bulk precursor phase. The creation of micro-states, which represent local minima in free energy stabilised by configurational factors, can also lead to hierarchical pathways, but the intermediates are transient states that do not appear on a bulk phase diagram. However, small changes in molecular structure can eliminate these transient states, leading to a direct pathway of nucleation. In either of these cases, reduction in molecular mobility, either through reduced temperature or introduction of ion-binding macromolecules, can freeze non-equilibrium states into place for dynamical reasons. ![]() Professor Jim de Yoreo, Pacific Northwest National Laboratory; University of Washington, USA
![]() Professor Jim de Yoreo, Pacific Northwest National Laboratory; University of Washington, USAJim De Yoreo is Chief Scientist for Materials Science at PNNL and Affiliate Professor of Materials Science and Engineering at the University of Washington. He received his PhD in Physics from Cornell University in 1985. Following post-doctoral work at Princeton University, he joined Lawrence Livermore National Laboratory in 1989, where he held numerous positions including Director of the Biosecurity and Nanosciences Laboratory. He joined Lawrence Berkeley National Laboratory in 2007 where he last served as Interim Director of the Molecular Foundry. Dr De Yoreo's research focuses on understanding self-assembly and crystallization in organic, biomolecular, and biomimetic systems through in situ imaging and spectroscopy. He has authored, co-authored, or edited over 200 publications and patents with more than 10,000 citations. He has received numerous awards and fellowships, serves on a number of editorial boards and professional society executive committees, and is the former President of the Materials Research Society. |
|
---|---|---|
09:50 - 10:35 |
Solute precipitation nucleation: advances in theory and simulation methods
Nucleation is the stochastic process that creates the first stable embryo of a new phase to initiate a phase transition. The mechanism is poorly understood because rare event processes like nucleation present special challenges to both experiments and simulations. The difficulties are particularly acute for multi-component condensed phase nucleation processes. New rare events approaches that reveal how thermodynamic and dynamical factors in nucleation will be presented. Particular emphasis will be given to the factors that influence polymorph selection including disparate growth rates of competing polymorphs, and the effects of additives on the free energy landscape. Finally, we will examine assumptions about interfacial free energies as a possible origin for continuing discrepancies between experimental and theoretical nucleation rates. ![]() Professor Baron Peters, University of California, Santa Barbara, USA
![]() Professor Baron Peters, University of California, Santa Barbara, USABaron Peters received his Doctorate in Chemical Engineering (PhD) from the University of California, Berkeley in 2004 under the instruction of Arup Chakraborty and Alexis T. Bell. He pursued postdoctoral studies under Bernhardt L. Trout at the Massachusetts Institute of Technology and also under Berend Smit in the CECAM at the Ecole Normale Superieure in Lyon, France. Baron joined the faculty at UCSB in 2008. He earned an NSF Faculty Early Career Development award in 2009. His research interests include: catalysis, nucleation, and electron transfer; statistical mechanics and electronic structure theory. In particular, he studies nucleation from solution and polymorph selection, catalysis on amorphous supports, and gated electron transfer mechanisms. |
|
11:05 - 11:50 |
In situ and time resolved challenges for nucleation and growth of minerals from solution
The formation of mineral phases control most biogeochemical element cycles on Earth, yet surprisingly, the molecular level reactions that lead to bonds between atoms in minerals to be made or broken - essentially to nucleate, grow, transform or dissolve a mineral phase - are hard to quantify. In the last decade our ability to follow such complex and highly dynamic reactions has massively improved, and novel in situ, time resolved and high-resolution methods now allow us to follow such reactions better. However, we still lack much of the fundamental knowledge about how ions are assembled to form a first nanoparticle both from the theoretical and experimental sense and thus the validations of reaction kinetics and mechanisms that have been inferred from observations of natural processes is still missing in many important Earth systems. I will show how mineral formation and transformations in carbonates [1] and sulphate [2-3] occurs, and what knowledge extracted from in situ, time resolved and high-resolution methods can teach us about nutrient cycling, primary productivity and mineral chemistry in modern or ancient geological settings. [1] Bots et al (2012) Crystal Growth and Design doi: 10.1021/cg300676b ![]() Professor Liane G. Benning, GFZ German Research Centre for Geosciences, Germany; University of Leeds, UK
![]() Professor Liane G. Benning, GFZ German Research Centre for Geosciences, Germany; University of Leeds, UKLiane G. Benning has a PhD from the Swiss Federal Institute of Technology (ETH) in Zurich, Switzerland after which she spent 3 years as a postdoc at Pennsylvania State University (USA). She moved to the University of Leeds (UK) in 1999 as a University Research Fellow and transitioned to a Chair in Experimental Biogeochemistry in 2007. After 16 years at the University of Leeds Liane has recently been appointed as a Professor in Interface Geochemistry at the German Research Centre for Geosciences in Potsdam, Germany where she is building up a new Research Group addressing various aspects related to mineral-fluid-microbe interfaces processes. Together with a slew of postdoctoral fellows and PhD students she focusses on the formation and / or breakdown of mineral phases both in natural settings and in laboratory analogue experiments that mimic various Earth surface conditions. Her team is elucidating molecular level mechanisms of mineral nucleation, growth and transformation using high-resolution electron, X-ray or neutron-based scattering, diffraction and micro-spectroscopic approaches. |
|
11:50 - 12:35 |
Multiscale modelling of polymer-controlled crystallisation of calcium rich minerals
Crystallisation of calcium rich minerals, such as calcium carbonate, calcium oxalate and hydroxyapatite, affects several forms of life, from plants and animals to human beings. These minerals are fundamental constituents of shells and bones, but are also associated to pathology. Understanding and controlling crystallisation of these materials at a molecular level in biological environment would allow medical scientists to design countermeasures to prevent common diseases, such as urinary tract stones and hydroxyapatite crystal deposition. Mineralisation in solution, assisted or inhibited by electrolytes, is a process that entails a broad range of size and time scales. To gain molecular insight by computer simulations thus requires carefully designed multiscale modelling techniques, and data analysis tools. Here we consider the case of calcium oxalate, the main component of kidney stones, and we investigate its nucleation and growth in aqueous solution, in the presence of polypeptides, e.g. poly-glutamate, that have been shown to slow down nucleation, and to select mineral structure and morphology. With a combination of classical and ab initio molecular dynamics, and advanced sampling techniques, we unravel the interaction of poly-glutamate with ions in solution and at the surface of crystalline calcium oxalate. Furthermore, we introduce a Hamiltonian adaptive resolution scheme (H-AdResS), which allows one to concurrently treat a liquid system at different levels of details at controlled thermodynamic conditions, thus making it possible to simulate directly the nucleation and growth of minerals in solution. ![]() Dr Davide Donadio, University of California Davis, USA
![]() Dr Davide Donadio, University of California Davis, USADavide Donadio is a theoretical condensed matter physicist. He earned his PhD in Materials Science in 2003 at the University of Milan, with a thesis on ‘Photoconductivity and photoelasticity of silica’, which featured electronic structure calculations and molecular dynamics simulations of silicate glasses. Eventually he moved to ETH Zurich (Professor Parrinello’s group), where he studied materials at extreme conditions and crystal nucleation. In 2007 he joined Professor Galli’s group at UC Davis and worked on nanoscale heat transport in thermoelectrics. From 2010 to 2015 he lead the independent Max Planck Research Group of ‘Theory of nanostructures’ at the MPI for Polymer Research (Mainz, Germany), investigating non-equilibrium processes at the nanoscale by molecular simulations. In 2014 he was appointed Ikerbasque professor at DIPC (Donostia, Spain). In 2015 he moved to the Department of Chemistry at UC Davis, where he continues his activity on polymer-controlled crystallization, surface chemistry, and nanophononics. He has published about 80 peer-reviewed articles and two book chapters. |
Chair
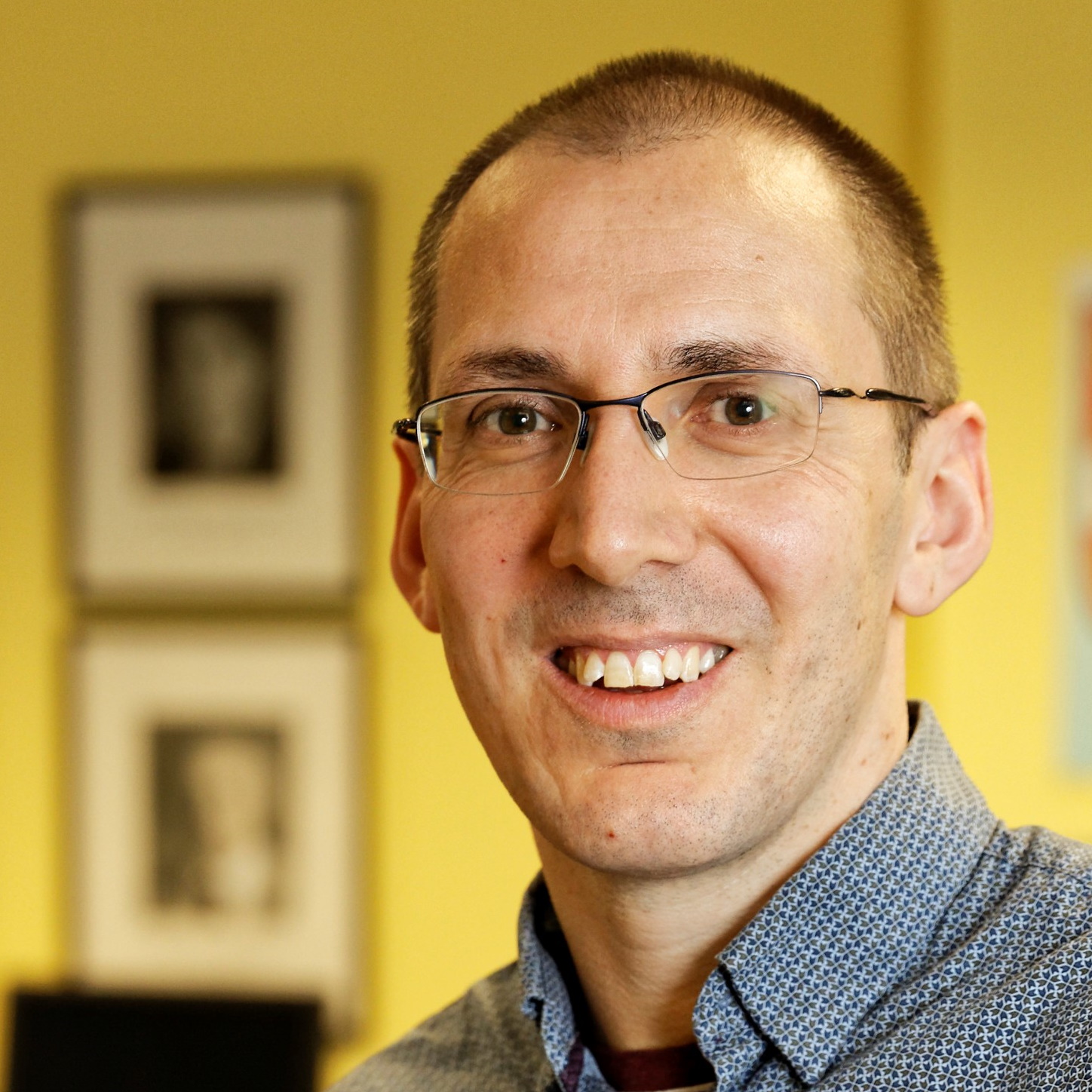
Professor Angelos Michaelides FRS, University of Cambridge, UK
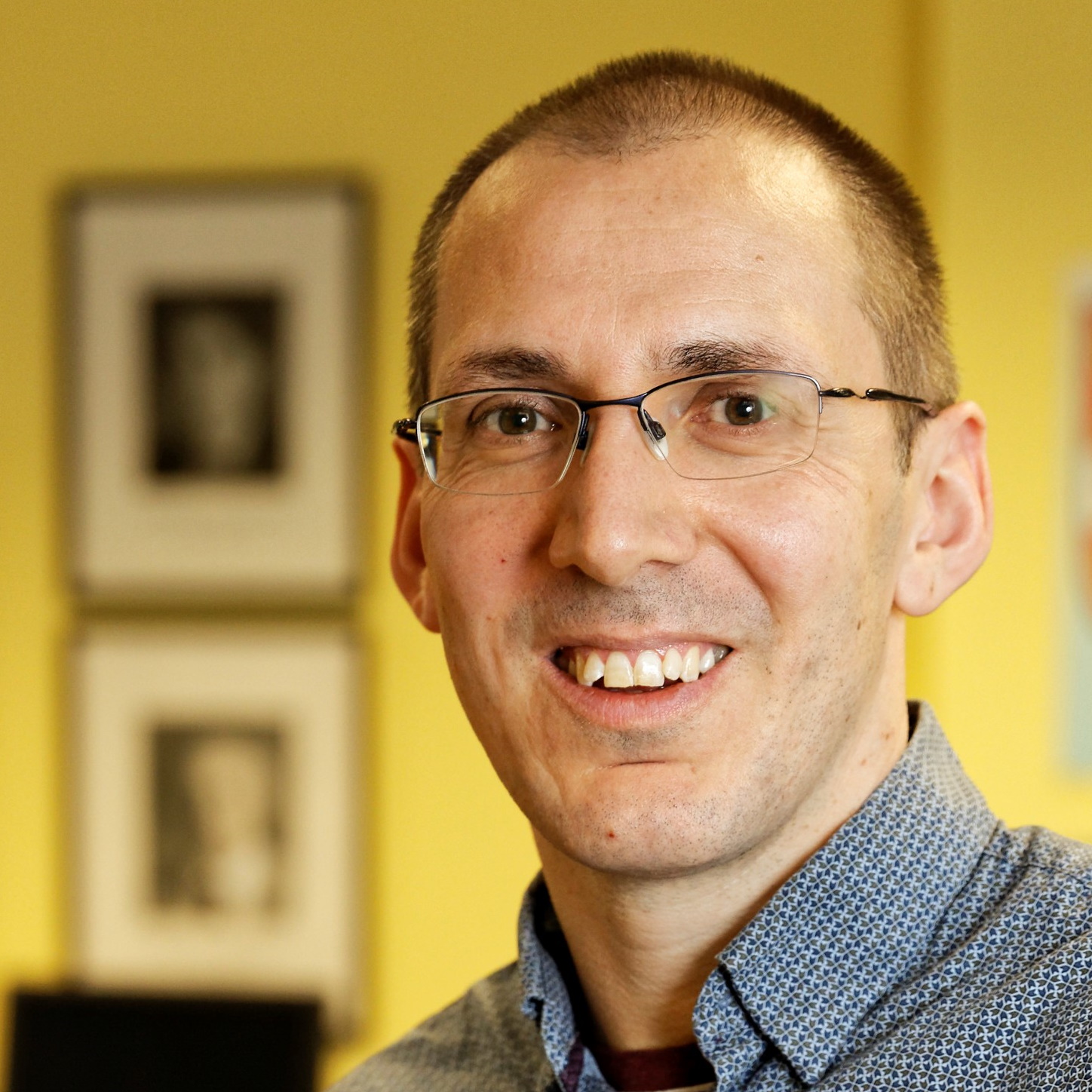
Professor Angelos Michaelides FRS, University of Cambridge, UK
Angelos Michaelides obtained a PhD in Theoretical Chemistry in 2000 from The Queen's University of Belfast. Following this, he worked as a Post-doctoral Research Associate and Junior Research Fellow at the University of Cambridge and then at the Fritz Haber Institute, Berlin as an Alexander von Humboldt Research Fellow and subsequently Research Group Leader. Between 2006 and 2020 he was at University College London where he was Director and Co-Director of the Thomas Young Centre: The London Centre for the Theory and Simulation of Materials and the founding Director of the Materials and Molecular Modelling Hub. Since 2020 he has been the 1968 Professor of Chemistry at the University of Cambridge.
13:30 - 14:15 |
Nucleation processes in aerosols and clouds
Atmospheric clouds strongly impact the weather and climate of our planet. Their properties are dependent upon how they form from preexisting aerosol particles by way of various nucleation pathways. The basics of cloud formation are well understood, but quantitative predictions of cloud properties and precipitation remains challenging, in part because of the diversity and variable abundance of aerosol particles. Here, we will focus on cloud processes that include ice nucleation. The formation of ice crystals is a key step during the initiation of atmospheric precipitation, and ice nucleation normally is the rate-limiting mechanism for ice particle formation. Homogeneous ice nucleation is prevalent for some atmospheric conditions, but often heterogeneous ice nucleation is the dominant pathway. Such heterogeneous ice nucleation is triggered by ice-nucleating particles, which traditionally were thought to consist primarily of mineral dust particles. But more recently, a wide variety of non-crystalline ice nucleators have been identified such as amorphous solids, pollen and bacteria, surfactant monolayers, and even dissolved molecules or molecular clusters. The presentation will discuss homogeneous and heterogeneous ice nucleation processes, covering both theoretical aspects as well as experimental developments, with a focus on the fundamental physical and chemical aspects of the involved processes. ![]() Professor Thomas Koop, Bielefeld University, Germany
![]() Professor Thomas Koop, Bielefeld University, GermanyThomas Koop received his PhD in Chemistry in 1996 at the Max Planck Institute for Chemistry in Mainz. After a postdoc at MIT in 1997-1998 he went to ETH Zurich in 1999, and was awarded his Habilitation in 2004. In the same year he joined the chemistry faculty at Bielefeld University, where he is Professor of Atmospheric and Physical Chemistry. He is also a cofounder and an executive editor of the journal Atmospheric Chemistry and Physics. The Koop Research Group studies the kinetics of phase transitions in aqueous systems, both theoretically and in laboratory experiments. These include homogeneous and heterogeneous ice nucleation processes with relevance to atmospheric cloud formation, deliquescence and efflorescence transitions of water-soluble crystalline aerosol particles, and vitrification processes of aqueous glasses. Another line of research involves studies of the properties and mechanism of action of antifreeze proteins in cryobiology. Koop and his group develop simple experimental methods, procedures for data analysis, as well as physicochemical parameterisations for use in more complex atmospheric models. |
|
---|---|---|
14:15 - 15:00 |
A state between liquid and crystal: locally crystalline but with the structure factor of a liquid
Using computer simulations of a simple model, we study a non-equilibrium state with a liquid-like S(k) but where over 95% of the molecules are in locally crystalline environments. Due to its liquid-like S(k) and slow dynamics, the state is apparently amorphous, although it contains nanocrystalline order. States such as this will have properties that are determined by local structure (possibly including electrical conductivity) that are crystal-like, despite scattering X-rays like liquids. Single crystals of two different polymorphs can form from this state. Which polymorph forms is determined long before the structure factor changes significantly. [Mithen & Sear, CGD 16, p3049 (2016)] ![]() Dr Richard Sear, University of Surrey, UK
![]() Dr Richard Sear, University of Surrey, UKI am mainly a computational physicist, although I work closely with experimentalists, and my current interests are in crystallisation and the dynamics of proteins inside living cells. I am interested in the fundamentals of how crystals form, and have studied crystallising systems from proteins to ice. Typical techniques are computer simulation to look at the microscopic mechanism of nucleation, and statistical models to model the phenomenology of crystallisation observed in experiment. My PhD (1995) was from Sheffield, and after postdocs at AMOLF in Amsterdam and the University of California Los Angeles, I have been at the University of Surrey since the late 1990s. |
|
15:30 - 16:15 |
Using synthetic macromolecules to mimic (and understand) antifreeze proteins
With an ever-ageing population in the Western world, the need for regenerative medicine, especially transplantation is increasing, but the methods to cryopreserve (and hence distribute) these cells and tissue still relies on addition of high concentrations of organic solvents. We seek to create new macromolecular solutions to address this problem. Antifreeze (glyco) proteins are found in many species adapted to live in the polar regions or at high altitudes, in sub-zero temperatures. These act to slow ice growth and also suppress the freezing point. Ice nucleating proteins are also found in many species, including Psuedomonus syringae, which promote frost formation on plants as a feeding mechanism. The exact mechanism (or mechanisms) of action of these proteins is still not clear. The relationships between the different macroscopic properties, such as ice shaping and ice growth inhibition are also not clear. To probe these questions, and to enable translation into applications, we have developed a range of synthetic polymer materials which can reproduce the properties of both antifreeze proteins and ice nucleating proteins, but by using simplified structures, and scalable synthetic methods. We have also developed ‘small molecule’ ice growth inhibitors which also raise question about the exact mechanisms of action. Guided by the above, we have applied these polymers to enhance cellular cryopreservation and enable solvent-freeze cryopreservation in some cases. ![]() Professor Matthew Gibson, University of Warwick, UK
![]() Professor Matthew Gibson, University of Warwick, UKProfessor Matthew I Gibson holds a personal chair in the Department of Chemistry and also the Medical School at the University of Warwick, UK. Matt completed his Degree (2003) and PhD (2007) at the University of Durham (UK), before post-doctoral research at EPFL (Switzerland). Matt is currently a Royal Society Industry Fellow, ERC Consolidator Grant holder and co-founder of Cryologyx Ltd. Matt’s multidisciplinary research group focusses on developing biomaterials to address healthcare challenges. Most recently they have developed tools for COVID-19 diagnostics and polymers to protect biologics during cold storage. |
|
16:15 - 17:00 |
Role of stacking disorder in ice nucleation
Freezing of water is central to the processes that determine Earth's climate. Accurate predictions of changes in weather and climate therefore hinge on good predictions of the rate of ice nucleation. Such rate estimates are based on extrapolations using classical nucleation theory (CNT), which assumes that the structure of nanometer-sized ice nuclei corresponds to that of bulk hexagonal ice, the thermodynamically stable form of ice. Recent simulations with various water models show that ice nucleated and grown under atmospheric temperatures is stacking disordered at all sizes; i.e., it consists of random sequences of cubic and hexagonal ice layers. This implies that either stacking disordered nuclei are more stable than hexagonal ice nuclei, or that they form because of non-equilibrium dynamical effects. Both scenarios challenge central tenets of Classical Nucleation Theory (CNT) and its validity for analysing and predicting ice nucleation rates. Here we use rare events sampling and free energy calculations with the mW water model to show that stacking disordered ice is the stable phase for nuclei with less than at least ~50,000 molecules. Stacking disordered critical nuclei at 230 K are 14.3±0.5 kJmol-1 more stable than hexagonal nuclei, favored by the entropy of mixing of cubic and hexagonal layers. This results in over three orders of magnitude higher nucleation rates with respect to CNT predictions. We find that the correction to CNT nucleation rates is significant over the whole range of temperatures relevant to homogeneous nucleation, and the most pronounced at the warmest conditions. This should have a strong impact on climate models, which are very sensitive to the parameterisation of ice nucleation rates. We conclude that CNT must be corrected for the dependence of the crystallisation driving force on nucleus size when interpreting and extrapolating ice nucleation rates from experimental laboratory conditions to temperatures important to clouds. ![]() Professor Valeria Molinero, The University of Utah, USA
![]() Professor Valeria Molinero, The University of Utah, USAValeria Molinero received a PhD in Physical Chemistry from the University of Buenos Aires in 1999, worked as associate scientist at Caltech and Arizona State University, and joined the faculty at The University of Utah in 2006, where she is Professor of Chemistry and Director of the Henry Eyring Center for Theoretical Chemistry. Molinero is interested in the interplay between microscopic structure, dynamics, and phase transformations in materials. The modelling of water, its anomalies and phase transitions has been a focus in her research in the last years. |
|
17:00 - 18:15 | Poster session |
Chair
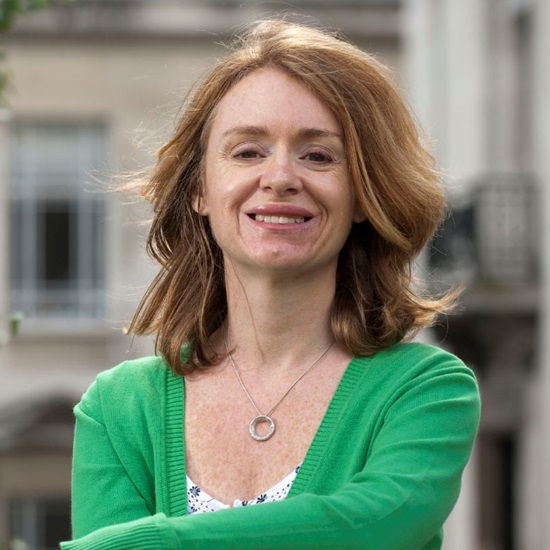
Professor Fiona Meldrum, University of Leeds, UK
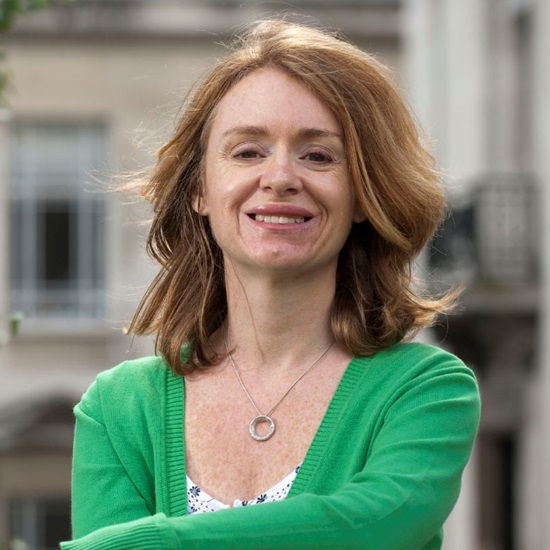
Professor Fiona Meldrum, University of Leeds, UK
Fiona Meldrum obtained her undergraduate degree from the University of Cambridge in 1989, and her doctorate in biological crystallization from the University of Bath in 1992. Following a postdoctoral position at the University of Syracuse, USA she carried out further postdoctoral work at the Max Plank Institute of Polymerforschung, Germany, before joining the Australian National University in Canberra as a Research Fellow. She returned to the UK to take up a lectureship at Queen Mary, University of London in 1998 and moved to the School of Chemistry, University of Bristol in 2003. She joined the University of Leeds in 2009 where she holds a Chair in Inorganic Chemistry. Her research focuses on crystallization, with particular emphasis on biomineralisation and bio-inspired crystal growth, and includes the major themes of additive-directed crystallisation, effects of confinement and topography on crystallisation, and crystals with composite structures and their structure-property relationships.
09:00 - 09:45 |
Clusters, complexes and primary particles in the early stages of bioinspired mineral growth
Biominerals possess shapes, structures and properties not found in synthetic minerals. The dream of exploiting the biological principles of controlled mineral formation in materials chemistry inspires a large community to investigate the underlying mechanisms through biomimetic mineralisation experiments.[1] The defining characteristics of biominerals arise from the interplay of the mineral with a macromolecular matrix, which directs crystal nucleation and growth. Within this three dimensional biomolecular assembly, the developing mineral interacts with acidic macromolecules, either dissolved in the crystallisation medium or associated with an insoluble templating structure.[2] CryoTEM has proven to be a powerful tool to investigate – with great detail – the nucleation and growth of different mineral systems, including calcium carbonate[3], calcium phosphate,[4] iron oxide[5] and silica.[6] It also allows us to study how organic-inorganic interactions at interfaces affect the crystallisation from solution.[7-9] Interestingly we find that all these pathways involve nanometer sized building blocks that have been termed prenucleation clusters,[7-9] prenucleation complexes[4] and primary particles.[5-6] Using time resolved cryoTEM we now also demonstrate how multistep nucleation pathways are altered through the influence of polypeptide based additives.[10] Controlling the pathways of nucleation and growth may help us to ultimately to control the size, shape and orientation of the crystals and optimise them for specific technological applications.[11] [1] Nudelman and Sommerdijk, Angewandte Chemie-International Edition 2012, 51, 6582. ![]() Professor Nico Sommerdijk, Technische Universiteit Eindhoven, The Netherlands
![]() Professor Nico Sommerdijk, Technische Universiteit Eindhoven, The NetherlandsNico Sommerdijk is full professor at Eindhoven University of Technology and head of the Laboratory of Materials and Interface Chemistry. In 1995 he obtained his PhD (Cum Laude) from the University of Nijmegen for his work on chiral amphiphiles. He did postdoctoral work on sol-gel silicates (1995-1997, University of Kent-UK), on bio-inspired crystallisation (1997, Keele University-UK) and on macromolecular self-assembly (1997-1998, Nijmegen-NL). In 1999 he moved to Eindhoven to work on bio-inspired hybrid materials through biomimetic mineralisation and self- organisation. He studies these processes combining (macro)molecular self-assembly and advanced electron microscopy. Professor Sommerdijk’s work has been supported by VIDI and VICI Awards from the Netherlands Science Foundation, and he is winner of the RSC Soft Matter and Biophysics Award 2015. He is director of Centre of Multiscale Electron Microscopy , core member of the Institute for Complex Molecular Systems, and member of the Eindhoven Polymer Laboratories and the Eindhoven Multiscale Institute. |
|
---|---|---|
09:45 - 10:30 |
Challenges for the simulation of nucleation pathways for biominerals
The nucleation of biominerals, such as calcium carbonate and calcium phosphate, has attracted significant attention in the last decade following the proposal of so-called 'non-classical' mechanisms. For the case of calcium carbonate it has been proposed that the pathway to formation under homogeneous conditions can include stable pre-nucleation clusters (PNCs) [1], liquid-liquid phase separation [2] and the formation of amorphous calcium carbonate as a thermodynamically stable phase for nanoparticles in a given size range [3]. Given the difficulties of probing such species in situ with experimental techniques, atomistic simulation is a valuable complementary approach. However, simulation faces a number of challenges of it’s own due to the limitations of molecular dynamics with the currently available computing power. For example, the concentrations of ions at saturation for most biominerals are below 1 mM, meaning that unbiased sampling of ion association in aqueous solution at experimental conditions is unfeasible. In this presentation several challenges for simulation of ion association from ion pairing, through pre-nucleation species, to determining critical nucleus size will be examined. This includes issues spanning the underlying accuracy of the free energy landscape through to how to map cluster stability in terms of a manageable set of collective variables that capture the association/dissociation pathways and connect them to identifiable thermodynamic states. Here examples will be drawn from aqueous calcium systems with carbonate, phosphate and oxalate. [1] D. Gebauer et al, Science, 322, 1819 (2008) ![]() Professor Julian Gale, Curtin University, Australia
![]() Professor Julian Gale, Curtin University, AustraliaProfessor Julian Gale obtained his first degree from the University of Oxford in Natural Sciences (Chemistry), where he continued on to study for a DPhil in the Department of Chemical Crystallography. After a postdoctoral position at the Royal Institution of Great Britain he moved to Imperial College London as a Royal Society University Research Fellow and subsequently Reader in Theoretical and Computational Chemistry. In 2003, he moved to his current location, Curtin University, as a Premier’s Research Fellow and now holds the position of John Curtin Distinguished Professor of Computational Chemistry. Recently he was awarded an Australian Research Council Laureate Fellowship and is a Fellow of the Australian Academy of Science. Research interests include the development and application of computational techniques to problems in areas including materials chemistry, crystallisation, geochemistry and mineralogy. |
|
11:00 - 11:45 |
Nucleation and growth processes of gas clathrate hydrates
Gas clathrate hydrates are crystalline inclusion compounds comprised of a three-dimensional network of hydrogen-bonded water molecules that can trap small gas molecules in the water cavities. The ability to control clathrate hydrate nucleation and growth processes is important in several energy applications, including during the production and transportation of oil/gas in subsea flowlines where gas hydrates can form blockages in the flowline, as well as energy storage of fuels in gas hydrate crystals. The nucleation and growth processes and inter-particle interactions of gas hydrate crystals on gas bubbles and water droplets in water and oil continuous systems are examined at high pressure and low temperature conditions. Addition of polymers and surface-active molecules can be used to modify these processes, e.g. delaying the nucleation and growth processes, or reducing the inter-particle interactions. The clathrate hydrate formation synthesis pathways can be a key strategy to designing higher storage capacity materials, and/or stabilising new stable and metastable crystal structures. Structure metastability has been observed through spectroscopic and computational studies. Examples of the use of different promoter guest molecules, synthesis methods, and pressure conditions are presented for the production of stable and metastable clathrate hydrate phases. These studies can help further our knowledge for developing clathrate materials for storage and other technologies. ![]() Professor Carolyn Ann Koh, Colorado School of Mines, USA
![]() Professor Carolyn Ann Koh, Colorado School of Mines, USACarolyn A. Koh is Professor of Chemical and Biological Engineering and Director of the Center for Hydrate Research at the Colorado School of Mines (CSM). She obtained her BSc (Hons) and PhD degrees from University of West London and postdoctoral training at Cornell University. She was a Reader at King’s College, London before joining the Colorado School of Mines. She has been visiting Professor at Cornell, Penn State and London University. She was a consultant for the Gas Research Institute in Chicago and is a Fellow of the Royal Society of Chemistry, Associate Editor of the Society for Petroleum Engineers Journal, a member of the Editorial Advisory Board of the ACS J. Chem. Eng. Data, US DOE Methane Hydrate Advisory Committee member, and served on the National Academies NRC committee assessing the US DOE National Methane Hydrate Program. She is also an active member of the joint ASME-AIChE Committee on Thermophysical Properties and organised/chaired/co-chaired sessions of the joint ASME-AIChE Thermophysical Properties Conferences. She has been elected co-Chair and Chair of the Gordon Research Conferences on Gas Hydrates in 2016 and 2018, respectively. She has established internationally recognized gas hydrate research programs over the last two decades at King’s College, University of London and the Colorado School of Mines. Her research is focused on understanding the nucleation, crystallisation and inhibition mechanisms and thermophysical properties of natural gas hydrates. She was awarded the Young Scientist Award of the British Association for Crystal Growth, the CSM Outstanding Faculty Member Award, Senior Class (2013) and Young Faculty Research Excellence Award (2012). She has over 140 publications in refereed journals, including Science, Physics Today, J. American Chemical Society, and two books, including Clathrate Hydrates of Natural Gases (the “third edition of a best seller” – quote from CRC Press publishers, co-authored with E.D. Sloan). |
|
11:45 - 12:30 |
Free energy landscape and molecular pathways of ice and gas hydrate nucleation
Gas hydrate and ice are similar in many aspects: both appear 'ice-like', and both form in aqueous environments as a result of ordering of water molecules. However the nucleation processes of two solids could proceed with very different pathways. Employing advanced molecular simulation methods, this talk explores the free energy landscape and molecular pathways of both ice and hydrate nucleation. This talk shows that ice nucleation, both homogeneous and heterogeneous, appears to follow a pathway described very well by classical nucleation theory (CNT). The nucleation of hydrate, on the other hand, has been often found to involve multiple steps, thus appearing non-classical. Indeed, structural analysis show that on average, hydrate formation is facilitated by a 'two-step' like mechanism involving a gradual transition from amorphous to crystalline structure. However analysis also shows the existence of direct nucleation pathways where hydrate crystallises without going through the amorphous stage. Interestingly, the calculated free energy profile was also found to fit reasonably well against CNT. The structural diversity and the CNT-like free energy profile imply that hydrate nucleation could be an entropically driven, kinetic process that proceeds via multiple pathways that have similar free energy profiles. ![]() Professor Tianshu Li, George Washington University, USA
![]() Professor Tianshu Li, George Washington University, USATianshu Li received his PhD in Materials Science from University of California, Berkeley in 2005. From 2006 to 2010, he continued his research as a post-doctoral scientist in Professor Giulia Galli’s group at University of California, Davis. He then joined the faculty of civil and environmental engineering department at the George Washington University in 2010. One of his main research interests is to understand nucleation process through molecular modelling, with current focus on ice and clathrate hydrate. Besides nucleation, his group is also interested in low-dimensional materials and energy materials under extreme conditions. |
Chair
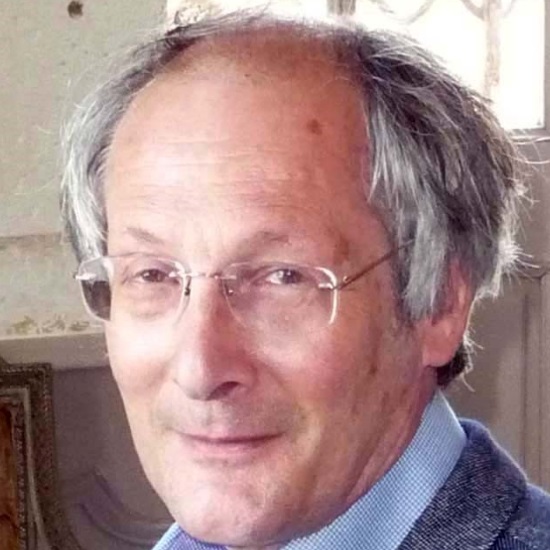
Professor Daan Frenkel ForMemRS, University of Cambridge, UK
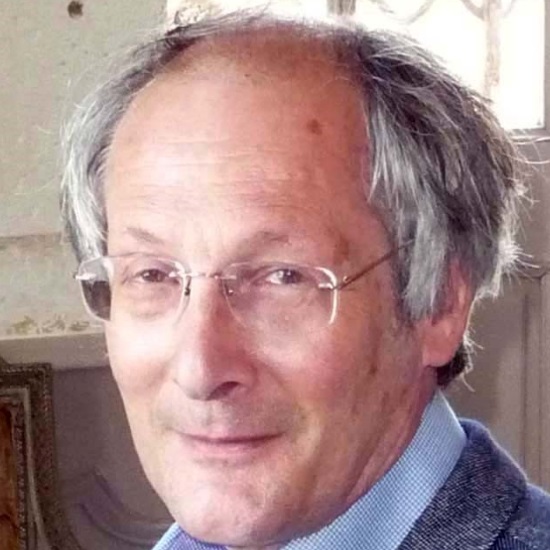
Professor Daan Frenkel ForMemRS, University of Cambridge, UK
Daan Frenkel (1948) received his PhD in Physical Chemistry from the University of Amsterdam in 1977. Until 1980, he was as a postdoc at UCLA. After that, he worked at Shell Research (Amsterdam), the Universities of Utrecht and Amsterdam and at the FOM Institute for Atomic and Molecular Physics. In 2007, he was appointed 1968 Chair of Chemistry (Cambridge). Daan was Head of Cambridge Chemistry Department from 2011 to 2015. He is a Foreign Member of the Royal Society (London), of the American Academy of Arts & Sciences, of the US National Academy of Sciences (USA), of the Netherlands Academy of Sciences, of the Academia Europaea and of the World Academy of Sciences. He is an Honorary Fellow of Trinity College Cambridge. He received numerous international prizes (including the 20161 IUPAP Boltzmann Medal, the Aneesur Rahman Prize of the APS and the Alder-CECAM Prize of the EPS).
13:30 - 14:15 |
Physical determinants of amyloid nucleation
The assembly of normally soluble proteins into large fibrils, known as amyloid aggregation, is associated with a range of pathologies, including Alzheimer's and Parkinson's diseases. Computer simulations, in combination with quantitative experiments, can provide valuable insights into the mechanisms of amyloid formation, helping to bridge experimental scales with microscopic mechanisms. Substantial evidence shows that disordered pre-amyloid oligomers − not the fully grown amyloid fibrils − are cytotoxic and involved in pathological processes. Yet, their relationship to fibrils is not well understood. Using computer simulations, we showed that at physiological conditions, disordered oligomers serve as nucleation centres for fibrils, and are crucial on-pathway species to amyloid formation, governing its kinetics [1]. Moreover, recent experiments have revealed that amyloid fibrils are able to catalyse formation of their copies from soluble peptides. By combining simulations with biosensing and kinetic measurements of the aggregation of Alzheimer’s Aβ peptide, we proposed a mechanistic explanation for the self-replication of protein fibrils. We find that the process is dominated by a single physical determinant − the adsorption of monomeric proteins onto the surface of fibrils [2]. Such mechanistic understanding not only has implications for future efforts to control pathological protein aggregation, but is also of interest for the rational assembly of nanomaterials, where achieving self-replication is one of the unfulfilled goals. [1] A. Šarić, Y. C. Chebaro, T. P. J. Knowles and D. Frenkel, PNAS 111, 17869 (2014) ![]() Dr Anđela Šarić, University of Cambridge; UCL, UK
![]() Dr Anđela Šarić, University of Cambridge; UCL, UKAnđela Šarić is a Human Frontier Science Program Fellow in the Department of Chemistry at the University of Cambridge. She is about to join the University College London Department of Physics & Astronomy and the Institute for the Physics of Living Systems as a junior group leader. Anđela received a PhD in Chemical Physics from Columbia University, New York, and a diploma in Chemistry from the University of Zagreb. She uses computer simulations to address physical mechanisms of biologically-relevant processes where collective behaviour arises. Her research interests thus far included self-assembly on biological membranes, aggregation of active matter, and pathways of amyloid nucleation. |
|
---|---|---|
14:15 - 15:00 |
Kinetics of protein aggregation
Filamentous protein aggregation underlies a number of functional and pathological processes in nature. This talk focuses on the formation of amyloid fibrils, a class of beta-sheet rich protein filament. Such structures were initially discovered in the context of disease states where their uncontrolled formation impedes normal cellular function, but are now known to also possess numerous beneficial roles in organisms ranging from bacteria to humans. The formation of these structures commonly occurs through supra-molecular polymerisation following an initial primary nucleation step. In recent years it has become apparent that in addition to primary nucleation, in secondary nucleation events which are catalysed the presence of existing aggregates can play a significant role in the dynamics of such systems. This talk describes our efforts to understand the nature of the nucleation processes in protein aggregation as well as the dynamics of such systems and how these features connect to the biological roles that these structures can have in both health and disease. ![]() Professor Tuomas Knowles, University of Cambridge, UK
![]() Professor Tuomas Knowles, University of Cambridge, UKTuomas Knowles studied Biology at the University of Geneva and Physics at ETH Zurich from where he graduated in 2004. He obtained a PhD in Biological Physics from the University of Cambridge in 2008 and joined the faculty at the Department of Chemistry at Cambridge in 2010 where he is currently professor of Physical Chemistry and Biophysics. His work is focused on the development and application of experimental and theoretical methods based on the physical sciences to study biomolecular behaviour and interactions in the context of both biological function and malfunction. |
|
15:30 - 16:15 |
Numerical simulation studies of ice nucleation at normal and extreme conditions
Combining simulations of spherical crystal seeds embedded in the metastable fluid with classical nucleation theory (seeding technique), we are able 1) to successfully describe the nucleation rate in a wide range of metastability; 2) to estimate the crystal-fluid interfacial free energy, in good agreement with explicit direct calculations. Among all freezing transitions, that of water into ice is probably the most relevant to biology, physics, geology, or atmospheric science. Making use of the seeding technique, we evaluate the homogeneous ice nucleation rate for several water models (TIP4P/2005,TIP4P/ice, TIP4P and mW) for temperatures between 15 and 35 K below melting. The values agree (within statistical error) with experimental measurements and confirm that water freezing above 20 K below melting has necessarily to happen heterogeneously. Estimating the ice-liquid interfacial free-energy, we conclude that 1) it depends on the chosen water model and is a key factor in the nucleation rate; 2) for all water models it decreases as the temperature decreases; 3) extrapolating our results of the interfacial free-energy to the melting temperature, we obtain a value between 25 and 32 mN/m, in reasonable agreement with experiments. We then establish the ice growth rate for TIP4P/ICE and mW water, and use the Avrami’s expression to estimate the crystallisation time. We find a crossover between a nucleation-controlled and a growth-controlled crystallisation regime, and argue that this could explain the apparent discrepancy observed among experimental values of the nucleation rate for temperatures below 230K. The avoidance of water freezing is the holy grail in the cryopreservation of biological samples, food and organs. Performing computer experiments to investigate ice nucleation at high pressures, we find a slowing down of the nucleation rate mainly due to the increase of the ice I-water interfacial free energy with pressure. ![]() Dr Chantal Valeriani, Universidad Complutense de Madrid, Spain
![]() Dr Chantal Valeriani, Universidad Complutense de Madrid, SpainChantal Valeriani studied Physics at the University of Rome (La Sapienza), performed her Masters project in the group of Professor Sciortino, and in 2007 obtained her PhD in Chemistry at the University of Amsterdam, working on understanding nucleation phenomena by means of numerical simulations in the group of Professor Daan Frenkel. After having spent three months investigating charged colloids in Professor Dijkstra's group (Utrecht University), she moved to Professors Cates, Poon and Pusey's Soft Matter group (University of Edinburgh) where she held a Marie Curie Individual fellowship. She focused on devitrification of colloidal glasses and on self-assembly of active particles. In 2011 she joined Professor's Vega group at the Universidad Complutense de Madrid as a Juan de La Cierva and Marie Curie Career Integration fellow, where she studied properties of water at extreme conditions and ice nucleation. In 2014 Chantal was awarded a Ramon and Cajal tenure track Assistant Professorship, and works as an independent researcher in the Applied Physics Department of the Universidad Complutense de Madrid. Her current research encompasses the study of the anomalous behaviour of metastable water and the assembly from out-of-equilibrium fluids (either ordered assemblies as in crystalline clusters, or disordered as in glasses and active particles) using a wide variety of numerical techniques. |
|
16:15 - 17:00 | Panel discussion and closing remarks |