Links to external sources may no longer work as intended. The content may not represent the latest thinking in this area or the Society’s current position on the topic.
Fusion energy using tokamaks: can development be accelerated?
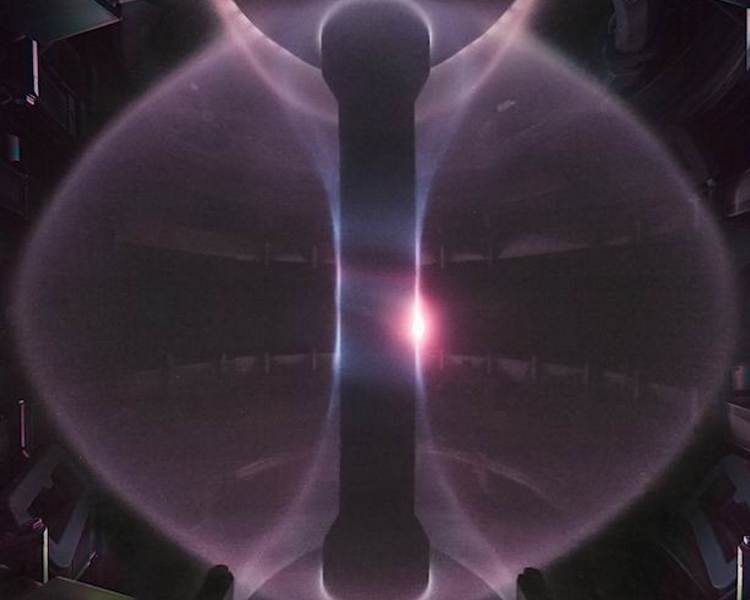
Scientific discussion meeting organised by Professor Colin Windsor FRS, Professor Dennis Whyte, Dr Jack Connor FRS, Dr Melanie Windridge and Professor Guenter Janeschitz.
Fusion power is one of the few options for abundant, safe, carbon-free energy. Steady progress has been made using tokamaks, but the flagship ITER remains years ahead. It is time for a discussion on whether new technologies, techniques and materials, such as high temperature superconductors, spherical tokamaks and composite materials could lead to faster development.
The schedule of talks and speaker biographies are available below. Recorded audio of the presentations is also available below. An accompanying journal issue for this meeting was published in Philosophical Transactions of the Royal Society A.
Attending this event
This meeting has taken place.
Enquiries: contact the Scientific Programmes Team
Organisers
Schedule
Chair
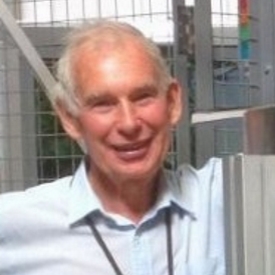
Professor Colin Windsor FRS, Tokamak Energy UK Ltd, UK
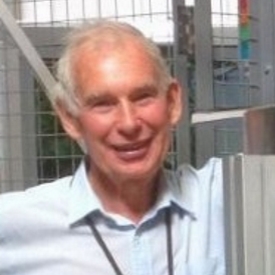
Professor Colin Windsor FRS, Tokamak Energy UK Ltd, UK
Colin Windsor studied at Oxford, gaining his DPhil in 1963, and was a post-doctorial fellow at Yale. Joining Harwell he performed experiments on neutron scattering for many years before joining Culham Fusion Laboratory in 1994. He worked on neural net control of the COMPASS-D tokamak, on the spherical tokamak START and on the JET tritium campaign of 1997. In retirement he has used neural networks for disruption prediction, mine detection using radar, and for signature recognition. He is a Fellow of the Royal Society (1995), the Institute of Non-Destructive Testing (1994), and the Institute of Physics (1975) who in 1986 awarded him their Duddell medal for developments in instrumentation. In 1990 he was made an Honorary Professor of Physics at Birmingham University. In 2013 he became a consultant to Tokamak Energy, a private company dedicated to accelerating the development of fusion power. He has worked on the key problem of the centre column shield neutronics with papers on the energy deposition, the shield modelling and the radiation damage.
09:10 - 09:40 |
Alternatives to tokamaks: a faster-better-cheaper route to fusion energy?
The tokamak may be the most successful type of device in fusion research but it is far from the only one. A few other parallel strands have been pursued for decades in government labs, but recently, in part because of slow progress in tokamak development, a new breed of fusion start-up companies has emerged to seek a faster-cheaper-better route to fusion energy. Stellarators, which look superficially like tokamaks but contain plasma differently, are currently championed by Germany’s Wendelstein 7-X which recently began operation. Inertial confinement fusion uses lasers to intensely compress small capsules of fuel and spark a burst of fusion. The leader in this field, the US National Ignition Facility, is currently struggling to get the spark to light but variants such as direct-drive and fast-ignition are waiting in the wings. Magneto-inertial fusion, which uses magnetic confinement followed by explosive compression, is being pursued by US national labs Los Alamos and Sandia, as well as some fusion start-ups. Others are banking on self-confining balls of plasma known as spheromaks or field-reversed configurations, or on electrostatic confinement in a device known as a Polywell. Dan Clery hopes to show there are more ways than one to skin a fusion cat. ![]() Daniel Clery, Science magazine
![]() Daniel Clery, Science magazineDaniel Clery has a BSc in theoretical physics from York University, UK. He has worked as an editor for the Institution of Electrical Engineers (now the Institution of Engineering and Technology) and the Institute of Physics on its Physics World magazine. He had two spells with New Scientist magazine, first editing technology news and later as news editor. For many years Clery was Science magazine’s European news editor and more recently senior correspondent, covering astronomy. Clery has written for a range of other publications, including Popular Science, the Bulletin of the Atomic Scientists, Huffington Post, the Financial Times, The European, and Cosmos. In June 2013 he published a popular history of the development of fusion research, entitled A Piece of the Sun: The Quest for Fusion Energy. |
|
---|---|---|
09:40 - 09:55 | Discussion | |
09:55 - 10:25 |
The European Fusion Roadmap towards fusion electricity
The European roadmap to the realisation of fusion electricity breaks the quest into eight missions. For each mission, it reviews the current status of research, identifies open issues, and proposes a research and development programme. ITER is the key facility of the roadmap as it is expected to achieve most of the important milestones on the path to fusion power. The Fusion Roadmap is tightly connected to the ITER schedule and the vast majority of fusion resources in Horizon 2020 are dedicated to ITER and its accompanying experiments. Parallel to the ITER exploitation in the 2030s, the construction of the demonstration power plant DEMO needs to be prepared. DEMO will for the first time supply fusion electricity to the grid. The design, construction and operation of DEMO require full involvement of industry to ensure that, after a successful DEMO operation, industry can take responsibility for commercial fusion power. ![]() Professor Tony Donné, EUROfusion, Germany
![]() Professor Tony Donné, EUROfusion, GermanyTony Donné has been involved in fusion research since 1985. Since June 2014 he has been Programme Manager (CEO) of EUROfusion, the consortium coordinating the R&D in the field of fusion science and technology in Europe and which is additionally responsible for the (pre-)conceptual design of the DEMO device. Until June 2014 he worked at the Dutch Institute for Fundamental Energy Research; from 2009 – 2014 as Head of the Fusion Research Division. Tony was director of the Dutch-Russian 'Center of Excellence on Fusion Physics and Technology' and programme director of the ITER-NL consortium. He is professor at Eindhoven University of Technology in Diagnostics and Heating of Fusion Plasmas. A substantial part of his scientific career was devoted to the design and use of plasma diagnostics at various fusion devices. He coordinated during 10 years the international activities in the field of diagnostics for ITER. |
|
10:25 - 10:40 | Discussion |
Chair
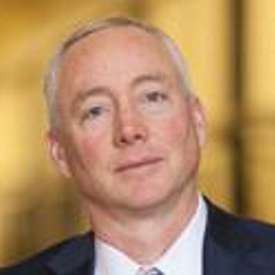
Professor Dennis Whyte, Massachusetts Institute of Technology, USA
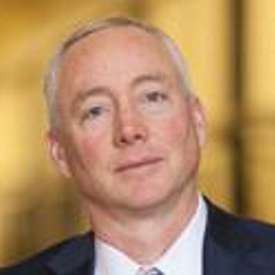
Professor Dennis Whyte, Massachusetts Institute of Technology, USA
A recognized leader in the field of fusion research using the magnetic confinement of plasmas for energy production, Professor Whyte’s work in magnetic fusion specializes on the interface between the plasma and materials.
Professor Whyte has over 300 publications and as an educator is heavily involved in student design activities through courses. Recently he has been working with students to advance surface and material measurement techniques of fusion and reactive power plant designs for pilot plants.
He has served as leader of the Boundary-Plasma Interface Topical Group of the US Burning Plasma Organization and is a Fellow of the American Physical Society.
11:05 - 11:35 |
An economical viable fusion reactor based on the ITER experience
This is Professor Janeschitz’s personal vision and outlook towards a fusion reactor based on his extensive experience from being part of the ITER design and now construction as well as leading the largest fusion technology program worldwide (KIT) for seven years. In particular he will to discuss how a fusion reactor can be economically viable without employing too advanced (SCFI) physics and technology. It certainly will be a pulsed machine (~ 20000 sec pulses) with thermal energy storage (turbine is steady state). He will also discuss the optimum machine size and toroidal field for such a machine and why he thinks that high field and smaller plasmas may not necessarily make a fusion reactor more competitive. When one extrapolates from today’s knowledge on ITER construction, even considering that ITER can be built much cheaper, it is clear that a fusion power plant will cost more than 10 or more likely more than 15 billion Euro / Dollar (the first of a kind even ~ 30 billion). Therefore in order to have an economically attractive fusion reactor it needs to produce a large amount of power (in the order of 2.5 GW electric). The possible size (R~10 m) and reasonably conservative physics basis of such a machine will be briefly described in the presentation. If we are successful in achieving advanced physics in a burning plasma, eg in ITER, then we can make the machine slightly smaller, but the principle arguments for a large machine will not change significantly. Key technologies and their status will be discussed with particular emphasis on a realistic blanket and divertor design and the size and issues of a T-plant for such a machine as well as the challenges which have to be overcome beyond what is needed for ITER. At the end a simple economic consideration will be discussed to show that a large machine could be economically acceptable even in today’s environment, in particular in competition to renewables. ![]() Professor Guenter Janeschitz, ITER Organisation, France
![]() Professor Guenter Janeschitz, ITER Organisation, FranceProfessor Guenter Janeschitz received his Diplom Ingenieure from the Technical University Vienna, and his PhD in physics from the Atominstitut at the Technical University Vienna in 1983. Following his PhD studies, he had various roles at IPP Garching and then spent a year with General Atomics in San Diego. In 1989 he returned to Europe to join the JET programme, becoming Head of the Fusion Programme in FZK in 2002. He moved to the ITER Organisation in 2008, and has been Deputy Head of the ITER Central Integration Office since 2015. |
|
---|---|---|
11:35 - 11:50 |
Discussion
|
|
11:50 - 12:20 |
The UKAEA Strategy for delivering fusion power
The talk will outline the key challenges which must be addressed to deliver fusion power and overview the facilities which UKAEA have now, and plan for the future, to address these challenges. UKAEA is helping UK industry to win key contracts for delivery of systems and components to ITER, and the talk will also explore how this connection to industry is vital for delivering fusion power. ![]() Professor Ian Chapman, UK Atomic Energy Authority, UK
![]() Professor Ian Chapman, UK Atomic Energy Authority, UKIan Chapman became CEO of the UK Atomic Energy Authority and Director of the Culham Centre for Fusion Energy in October 2016. He has held a number of international roles in fusion, including membership of the Princeton Plasma Physics Advisory board, the Chinese Academy of Sciences Plasma Physics advisory board and chairing the IAEA International Fusion Research Committee. His research has been recognised with a number of international awards, including the Institute of Physics Paterson Medal in 2013, the European Physical Society Early Career Prize in 2014, the American Physical Society Stix Award in 2017 and the Royal Society Kavli Medal in 2019. He was made a Fellow of the Institute of Physics in 2013 and became a visiting Professor at Durham University in 2015. |
|
12:20 - 12:35 | Discussion |
Chair
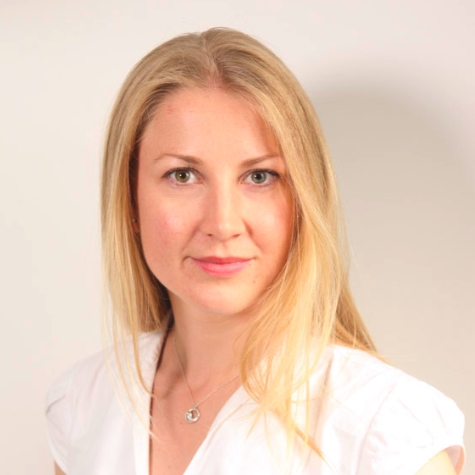
Dr Melanie Windridge, Tokamak Energy UK Ltd, UK
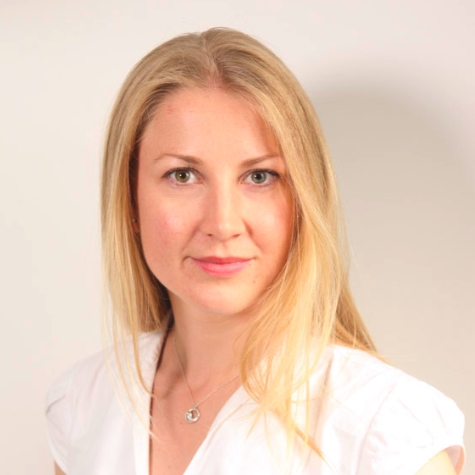
Dr Melanie Windridge, Tokamak Energy UK Ltd, UK
Melanie is Communications Consultant for Tokamak Energy. She has a PhD in Plasma Physics from Imperial College London during which time she worked on vertical stability in the MAST tokamak at Culham Centre for Fusion Energy. Subsequently she worked with Swiss inventions company Iprova and is named inventor on various patents.
Melanie’s communication work is wide-ranging and she regularly engages with the media, education institutions and the general public to talk about science and the role it can play in shaping the future. She is an ambassador for the government-led Your Life campaign encouraging more students into science and maths careers.
Melanie is the author of an introductory book on fusion energy, entitled Star Chambers: the race for fusion power, and of the narrative popular science book Aurora: In Search of the Northern Lights, for which she was awarded the Institute of Physics Rutherford Plasma Physics Communication Prize 2017.
13:30 - 14:00 |
Technical challenges to fusion power
The technical challenges to fusion power are wide and varied, from advanced engineering and manufacturing methods, through novel materials in extreme environments to the swirling, writhing plasma we are seeking to contain. This talk will focus on just one area, that is rich in physics and sits at the heart of any fusion reactor – the plasma. In a tokamak, the plasma is confined in a toroidal geometry by magnetic field. Professor Wilson will explore how turbulence degrades the confinement, and is the main driver for pushing up the size of fusion reactors. However, there are circumstances when the turbulence is suppressed, resulting in regions of steep pressure gradient in the plasma. While good for confinement, the associated free energy drives filamentary plasma eruptions, like miniature solar flares, which on a device like ITER would cause excessive damage. This has led to methods to control these eruptions, which we shall review. The heat and particles that leak from the magnetic confinement system are handled in a region that is remote from the plasma core, called the divertor. Design of the divertor is one of the biggest challenges facing fusion, driving innovative solutions such as the 'Super-X' divertor on the UK’s MAST-U tokamak which will begin operation during 2018. ![]() Professor Howard Wilson, University of York, UK
![]() Professor Howard Wilson, University of York, UKHoward Wilson joined the UK Atomic Energy Authority in 1988 as a theoretical plasma physicist after graduating with a PhD in theoretical high energy particle physics from Cambridge. He moved to York in 2005, where he was appointed to a Chair in Plasma Physics, and then appointed as Director of the York Plasma Institute in 2011. He has been Director of the EPSRC Centre for Doctoral Training in the Science and Technology of Fusion Energy since 2012 (led by York, in partnership with the universities of Durham, Liverpool, Manchester and Oxford). Most recently, he was appointed as UKAEA Programme Director in 2017, a post which he holds alongside his Chair at the University of York. He has served on a number of international fusion programme advisory committees including for the Helmholtz Association, Research Laboratory for the Physics of Advanced Plasmas, St Petersburg, the KSTAR tokamak (South Korea) and Australia National University. In his research career, he has published over 160 papers in peer-reviewed journals. He served as co-editor for the journal Euro-physics Letters, and now is an editorial board member for the IAEA Nuclear Fusion journal. He has been a Fellow of the Institute of Physics since 2004. International awards include a Royal Society Wolfson Research Merit Award (2011), and the American Physical Society John Dawson Award for Excellence in Plasma Physics Research (2013). |
|
---|---|---|
14:00 - 14:15 |
Discussion
|
|
14:15 - 14:45 |
JT-60SA and Japanese tokamak programmes
The construction of JT-60SA by Japan and Europe is progressing on schedule towards its first plasma in September 2020. As of February 2018, more than 90% of the component manufacture has been completed and closure of the vacuum vessel is close to be accomplished. The objectives of the JT-60SA project are i) contribution to the success of ITER, ii) complementation of ITER for DEMO in all major areas of plasma development, and iii) fostering the next generation who are leading ITER and DEMO. JT-60SA is a highly-shaped large superconducting tokamak (maximum plasma current 5.5 MA, major radius ~3 m, aspect ratio ~2.6, elongation ~1.8, triangularity ~ 0.4) with a variety of plasma control actuators including high power heating (41 MWx100 s). This device is capable of confining break-even-equivalent class deuterium plasmas lasting for a duration (typically 100 s) longer than the time scales characterizing the key plasma processes in the ITER and DEMO-relevant plasma regimes. JT-60SA also pursues fully non-inductive steady-state operations with high plasma pressure (beta-value) exceeding the no-wall ideal MHD stability limit. The JT-60SA Research Plan ver.3.3 has been documented by ~ 400 scientists in Japan and the EU. Nationally coordinated research is being carried out in Japanese universities using various specialized 8 Spherical Tokamak (ST) devices towards realization of a component test facility and a power reactor. Research topics pursued on these devices include plasma current start-up using RF waves and coaxial helicity injection etc, advanced fuelling using compact toroid injection, ultra-high-beta plasma formation using magnetic reconnection heating by plasma merging. Including JT-60SA, tokamak research in Japan systematically studies highly-shaped high-beta plasmas with non-inductive current drive. ![]() Dr Yutaka Kamada, National Institutes for Quantum and Radiological Science and Technology, Japan
![]() Dr Yutaka Kamada, National Institutes for Quantum and Radiological Science and Technology, JapanDr Kamada is Director of the Department of Advanced Plasma Research, Naka Fusion Institute, National Institutes for Quantum and Radiological Science and Technology (QST), andJapanese Project Manager of the JT-60SA Project. He is also Chair of ITER Science and Technology Advisory Committee (STAC) and the representative of Japan on the ITPA (International Tokamak Physics Activity) Coordinating Committee. He graduated from the University of Tokyo, Department of Nuclear Engineering in 1988, then started tokamak experimental studies at JT-60U. The main research fields are; development of steady-state high integrated performance tokamak plasmas, study on high beta plasmas and study on edge and pedestal physics. |
|
14:45 - 15:00 | Discussion |
Chair
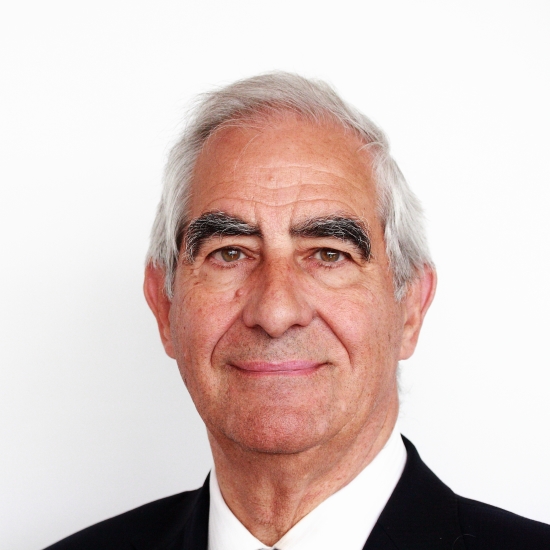
Sir Keith O'Nions HonFREng FRS, Cambridge Enterprise, University of Cambridge, UK
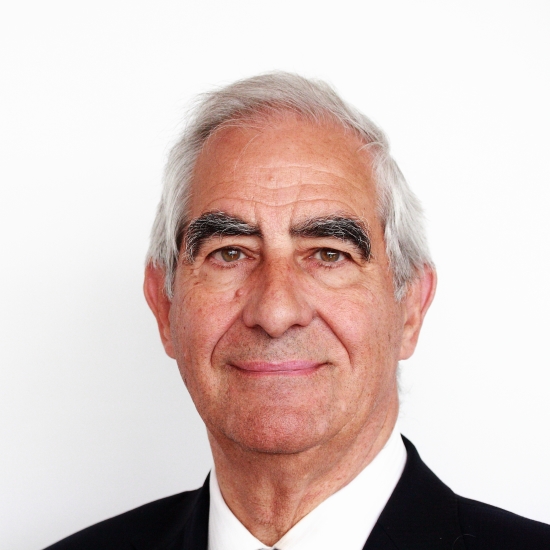
Sir Keith O'Nions HonFREng FRS, Cambridge Enterprise, University of Cambridge, UK
Speaker biography not available
15:30 - 16:00 |
On the size of tokamak fusion power plants
Studies for conventional tokamak power plants usually end up with a major radius R0 of the order 8-9 m, ie significantly beyond the largest existing tokamak, JET (R0 = 3m), and also in excess of the size of ITER (R0 = 6.2 m). This can be understood from a simple 0-D scaling [1] by the need to operate in a state of large power amplification Q=Pfus/PAUX > 30, where Pfus is the fusion power and PAUX the auxiliary heating power to compensate for residual plasma energy loss. However, these considerations assume a technical limit of the confining magnetic field B in line with the limits of the ITER design. Recent advances in High Temperature Superconducting Coil technology have led to proposals based on higher B, leading to more compact devices, i.e. smaller R0 [2], [3]. In this contribution, Professor Zohm argues that the definition of tokamak ‘size’ should include the magnetic field to remove the ambiguity in the discussion of ‘size dependence’ of the performance of fusion power plants [3], [4]. He also analyses the possibilities that higher B would offer, using an extension of the 0-D model used in [1]. Different routes of taking advantage of higher field are discussed. It is shown that, one also has to consider consistently the assumptions about plasma performance, such as confinement quality, operational limits or exhaust schemes. Finally, Professor Zohm discusses some of the significant implications for future R&D needed to make higher magnetic field in reactor-grade devices a reality. [1] H. Zohm, Fusion Sci. Technology 58 (2010) 613. ![]() Professor Hartmut Zohm, Max-Planck-Institute of Plasma Physics, Germany
![]() Professor Hartmut Zohm, Max-Planck-Institute of Plasma Physics, GermanyProfessor Hartmut Zohm received his PhD in Physics from Heidelberg University in 1990. In 1991, he got the Otto-Hahn-Medal by the Max-Planck Society for his PhD work. In 1996, he received his Habilitation at Augsburg University and became a professor in Plasma Research at Stuttgart University. Since 2000, he is a director at Max-Planck-Institute for Plasma Physics. Professor Zohm’s main fields of interest are the magnetohydrodynamic stability of fusion plasmas and their heating by Electron Cyclotron Resonance Heating. By combining these two fields, he pioneered active stabilisation of neoclassical magnetic islands, which set a major performance limit to the tokamak. For this work, he received the John Dawson Award by the APS in 2014 and the Hannes Alfvén Prize in 2016. His present field is the study of tokamak physics on the ASDEX Upgrade tokamak which is operated by his department. More recently, he became involved in the European studies for a demonstration fusion power plant (DEMO). |
|
---|---|---|
16:00 - 16:15 | Discussion | |
16:15 - 16:30 |
Smaller and quicker with STs and HTS
Research in the 1970s and 80s by Sykes, Peng, Jassby and others showed the theoretical advantage of the spherical tokamak (ST) shape. Experiments on START and MAST at Culham throughout the 1990s and 2000s, alongside other international STs like NSTX at the Princeton Plasma Physics Laboratory, confirmed their increased efficiency (namely operation at higher beta) and tested the plasma physics in new regimes. However, whilst interesting devices for study, the perceived technological difficulties due to the compact shape initially prevented STs being seriously considered as viable power plants. Then, in the 2010s, high temperature superconductor (HTS) materials became available as a reliable engineering material, fabricated into long tapes suitable for winding into magnets. Realising the advantages of this material and its possibilities for fusion, Tokamak Energy proposed a new spherical tokamak path to fusion power and began working on demonstrating the viability of HTS for fusion magnets. The company is now operating a compact tokamak with copper magnets, R0~0.4m, R/a~1.8, and target Ip=2MA, Bt0=3T, whilst in parallel developing a 5T HTS demonstrator tokamak magnet. Here Dr Windridge will discuss why HTS can be a game-changer for tokamak fusion. She will outline Tokamak Energy’s solution for a faster way to fusion and discuss plans and progress, including benefits of smaller devices on the development path and advantages of modularity in power plants. She will indicate some of the key research areas in compact tokamaks and introduce the physics considerations behind the ST approach, to be further developed in the talk by Alan Costley. ![]() Dr Melanie Windridge, Tokamak Energy UK Ltd, UK
![]() Dr Melanie Windridge, Tokamak Energy UK Ltd, UKMelanie is Communications Consultant for Tokamak Energy. She has a PhD in Plasma Physics from Imperial College London during which time she worked on vertical stability in the MAST tokamak at Culham Centre for Fusion Energy. Subsequently she worked with Swiss inventions company Iprova and is named inventor on various patents. Melanie’s communication work is wide-ranging and she regularly engages with the media, education institutions and the general public to talk about science and the role it can play in shaping the future. She is an ambassador for the government-led Your Life campaign encouraging more students into science and maths careers. Melanie is the author of an introductory book on fusion energy, entitled Star Chambers: the race for fusion power, and of the narrative popular science book Aurora: In Search of the Northern Lights, for which she was awarded the Institute of Physics Rutherford Plasma Physics Communication Prize 2017. |
|
16:30 - 16:45 |
Towards an ST fusion pilot plant
System code and analytical studies have shown that in addition to the conventional large size, high aspect ratio approach to realising fusion power, there could be an approach based on the low aspect ratio spherical tokamak at much smaller size [1]. Small devices would enable accelerated tokamak development because they offer relatively rapid and less expensive development cycles. However, small devices require novel technology and advanced engineering. High temperature superconductors are potentially the enabling technology because they can provide and withstand the necessary high fields used in the toroidal magnets. Other areas are important too, for example plasma start-up and ramp down with a limited, or without, a solenoid, divertor loads, and stresses in the magnet structure. Tokamak Energy is pursuing a development programme that aims to realise this alternative route to fusion power. In this presentation, the physics basis of the approach will be outlined and the key technology and engineering aspects will be highlighted and potential solutions identified. A technology roadmap to deal with the physics and engineering challenges on this path to fusion is under development and will be briefly introduced. [1] A. E. Costley, J. Hugill and P Buxton, 2015, ‘On the power and size of tokamak fusion pilot plants and reactors’, Nuclear Fusion 55, 033001. ![]() Dr Alan Costley, Tokamak Energy UK Ltd, UK
![]() Dr Alan Costley, Tokamak Energy UK Ltd, UKAlan Costley studied physics at Brunel University and Imperial College graduating with a PhD in 1976. Early professional years were spent at the National Physical Laboratory where he developed techniques in sub-millimeter wave spectroscopy. In 1983 he joined the JET project at the Culham Laboratory as leader of one of the diagnostic groups. From 1994 – 2009 he was Head of the Diagnostics in ITER. He now works as a consultant and in recent years has consulted for the ITER Organisation, the Princeton Plasma Physics Laboratory, and Tokamak Energy Ltd. He was awarded the Charles Vernon Boys Prize of the Institute of Physics for distinguished research in experimental plasma physics by a young researcher. In 2008 he was elected a Fellow of the American Physical Society. He has published about 300 papers including about 40 invited papers at international conferences mainly in the areas of plasma physics and plasma diagnostics. |
|
16:45 - 17:00 | Discussion |
Chair
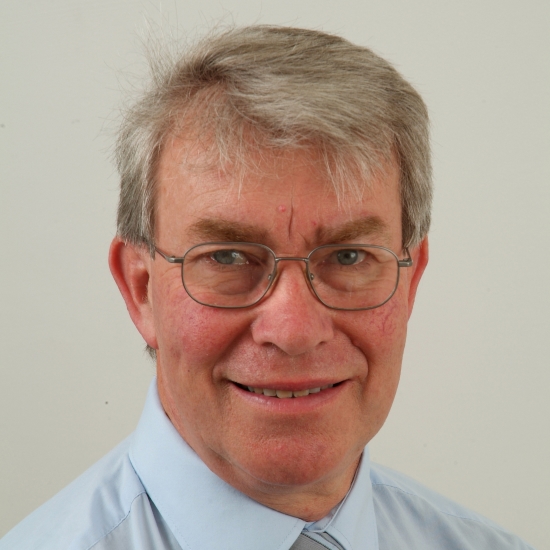
Dr Jack Connor FRS, Culham Centre for Fusion Energy and Tokamak Energy UK Ltd
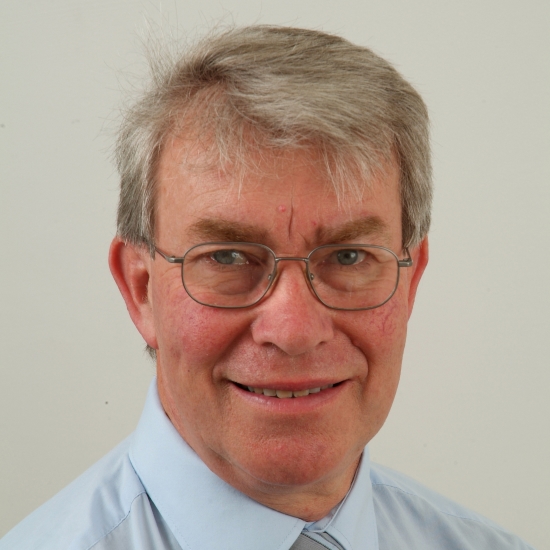
Dr Jack Connor FRS, Culham Centre for Fusion Energy and Tokamak Energy UK Ltd
Jack Connor FRS received a BSC in Mathematical Physics and a PhD in elementary particle physics from the University of Birmingham. He then joined Culham Laboratory’s Theory Division in 1967, becoming head of the Theory Division in 1989. He was elected as a Fellow of the Insitute of Physics in 1992. He was promoted to a senior level with the UKAEA in 2004. After he retired from Culham in 2007, he became a visiting professor at Imperial College London. Jack was elected to the Fellowship of the Royal Society in 2010. He won the Hannes Alfven Prize of the EPS in 2007 and the Payne-Gaposchkin Prize of the IoP in 2008. Jack has written seminal papers on bootstrap current, invariance principles and energy confinement scaling laws, impurity transport, ballooning theory, micro-stability and tearing mode stability in toroidal geometry, neoclassical tearing modes, and peeling-ballooning theory for ELMs. He has been involved with the Advisory Panel for Tokamak Energy, the Varenna Theory Workshop, the EU-US Transport Task Force, EU Ad Hoc Groups on Theory and Computing, and ITER transport modelling activity.
09:00 - 09:30 |
Fusion Nuclear Science Facility and Pilot Plant studies at PPPL
A Fusion Nuclear Science Facility (FNSF) could play an important role in the development of fusion energy by providing the nuclear environment needed to develop fusion materials and components. The spherical torus/tokamak (ST) is a leading candidate for an FNSF due to its potentially high neutron wall loading and modular configuration. A key consideration for the choice of FNSF configuration is the range of achievable missions as a function of device size. Possible missions include: providing high neutron wall loading and fluence, demonstrating tritium self-sufficiency, and demonstrating electrical self-sufficiency. Progress in ST-FNSF missions vs configuration studies including dependence on plasma major radius R for a range 1m to 2.2m are described for devices with copper toroidal field coils. Further, an A=2, R = 3m device incorporating high-temperature superconductor toroidal field coil magnets capable of high neutron fluence and both tritium and electrical self-sufficiency is also presented following systematic aspect ratio studies. Lastly, previous pilot plant studies have shown that electricity gain is proportional to the product of the fusion gain, blanket thermal conversion efficiency, and auxiliary heating wall-plug efficiency. The interplay between a range of physics and technology innovations for enabling compact tokamak/ST pilot plants will also be described. ![]() Dr Jonathan Menard, Princeton Plasma Physics Laboratory, USA
![]() Dr Jonathan Menard, Princeton Plasma Physics Laboratory, USADr Menard is the Research Director for the National Spherical Torus eXperiment – Upgrade (NSTX-U) and is responsible for guiding the scientific research program of NSTX-U working with the NSTX-U national research team. His research interests include the equilibrium and stability properties of spherical torus (ST) and tokamak plasmas, advanced operating scenarios in the ST, and the development of next-step options for fusion energy. |
|
---|---|---|
09:30 - 09:45 | Discussion | |
09:45 - 10:15 |
Small, modular and economically attractive fusion enabled by HTS superconductors
The origin, development and new opportunities of an accelerated strategy for fusion energy based on the high-field approach are developed. In this approach confinement devices are designed at the maximum possible value of vacuum magnetic field strength, B. The integrated electrical, mechanical and cooling engineering challenges of high-field on coil (Bcoil), large-bore electromagnets are described. These engineering challenges are confronted because of the profound science advantages provided by high-B: high fusion power density, ~B4, in compact devices, thermonuclear plasmas with significant stability margin, and, in tokamaks, access to higher plasma density. Two distinct high-field strategies were previously considered. The first was compact, cryogenically-cooled copper devices (BPX, IGNITOR, FIRE) with Bcoil>20 T, while the second was a large-volume, Nb3Sn superconductor device with Bcoil < 12 T; with the second path exclusively chosen ca 2000 with the ITER construction decision. The reasoning, advantages and challenges of that decision are discussed. Yet since that decision, a new opportunity has arisen: compact, Rare Earth Barium Copper Oxide (REBCO) superconductor-based devices with Bcoil > 20 T; a strategy that essentially combines the best components of the two previous strategies. Recent activities examining the technology and science implications of this new strategy are examined. On the technology side, REBCO superconductors have now been used to produce Bcoil>40 T in small-bore electromagnets, enabled by rapid progress in manufactured REBCO conductor quality, coil modularity and flexible operating temperature range. Specific tokamak designs on this path will be described. ![]() Professor Dennis Whyte, Massachusetts Institute of Technology, USA
![]() Professor Dennis Whyte, Massachusetts Institute of Technology, USAA recognized leader in the field of fusion research using the magnetic confinement of plasmas for energy production, Professor Whyte’s work in magnetic fusion specializes on the interface between the plasma and materials. Professor Whyte has over 300 publications and as an educator is heavily involved in student design activities through courses. Recently he has been working with students to advance surface and material measurement techniques of fusion and reactive power plant designs for pilot plants. He has served as leader of the Boundary-Plasma Interface Topical Group of the US Burning Plasma Organization and is a Fellow of the American Physical Society. |
|
10:15 - 10:30 | Discussion |
Chair
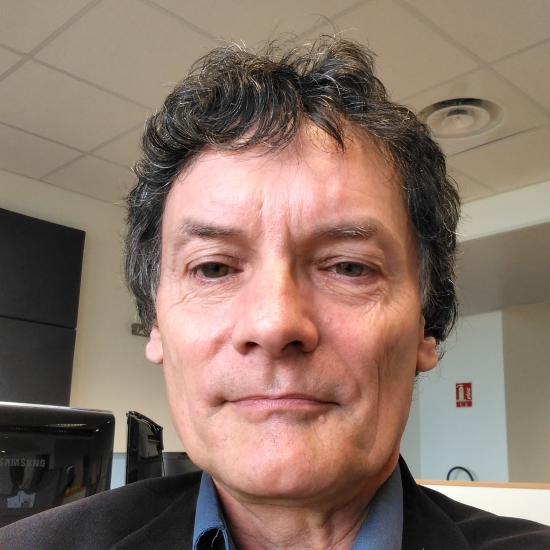
Mr Tom Todd, Culham Centre for Fusion Energy (retired), UK
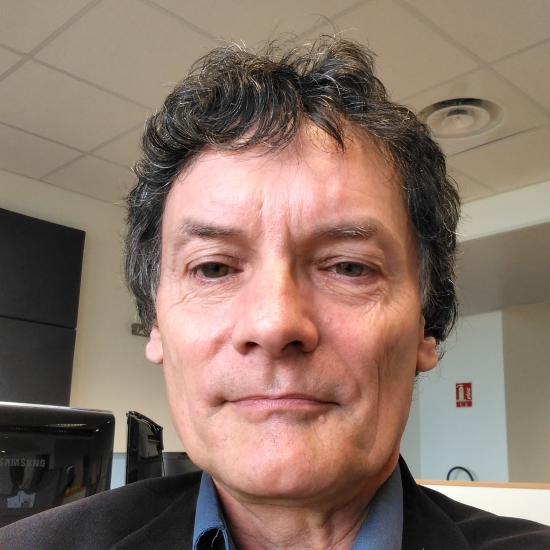
Mr Tom Todd, Culham Centre for Fusion Energy (retired), UK
Tom Todd has worked in fusion for almost 40 years. At Culham this included the superconducting levitron, small stellarators and tokamaks, with a secondment to the DIII-D tokamak in San Diego, and later Culham's CLEO and Compass experiments plus serving on design reviews for proposed fusion devices including ITER. Further roles were in tokamak diagnostics and technology transfer to industry. He helped to design the START and MAST spherical tokamaks, chairing the MAST Advisory Committee, then in 1999 went to Dounreay to manage the Design Services Department for nuclear decommissioning. In 2004 he became Chief Engineer at Culham, responsible for JET and MAST, then in 2011 Chief Technologist. Retired from UKAEA in 2014, he is now a fusion systems consultant and lecturer. A Fellow of the Institute of Mechanical Engineers and a member of the Institute of Physics, his publication list comprises around 160 papers.
11:00 - 11:30 |
Engineering challenges for Accelerated Demonstrators
There are numerous engineering challenges in realising fusion as an economic source of power; some have been discussed for decades, such as the degradation of materials under irradiation by the neutron spectrum created by the burning plasma and some have become more visible since the advent of international studies on demonstration reactors, such as the need for efficient remote maintenance schemes. These have been explored in various detail and with different approaches that may depend upon the size of the fusion device under consideration. For example, it is well known that the stress and critical current density in the central column magnet is a limiting factor in reducing the size of tokamaks. Similarly, the power loading (neutron, plasma and radiative) on the first wall limits the feasible fusion power for a given size of tokamak. Then there are a number of challenges that the various DEMO concept designs have revealed and yet another set that remain relatively unexplored and these could prove to be obstacles to the timely development of fusion regardless of the size of the tokamak considered. The use of uncommon materials for structural and functional purposes, for example, raises issues not just of component manufacture and standards but also of availability in the required quantities and quality. Interpreting ‘accelerating fusion’ in a wider sense, issues relating to the design and construction of small tokamaks, large tokamaks and areas in common will be analysed, particularly in respect of developmental timescales. ![]() Dr Elizabeth Surrey, Culham Centre for Fusion Energy, UK
![]() Dr Elizabeth Surrey, Culham Centre for Fusion Energy, UKDr Elizabeth Surrey is the Head of Technology at CCFE where she is responsible for the UK contribution to the Power Plant Physics and Technology Programme within Eurofusion and for the UK Technology Growth programme within the EPSRC Fusion Programme. Under her leadership CCFE has taken a significant role in the EU DEMO concept design, leading the integration studies, remote maintenance, safety, engineering design codes and standards and the socio-economic studies supporting power plant development in addition to contributing in many areas of engineering design and scientific research. Elizabeth has worked at Culham for 30 years in various areas encompassing industrial and defence applications of particle beams, including OEM of injector systems for commercial cyclotrons, and neutral beam injectors for fusion. Her current research interests include fusion power plant design, advanced materials and manufacture, the application of virtual engineering to fusion and fusion strategy. |
|
---|---|---|
11:30 - 11:45 | Discussion | |
11:45 - 12:15 |
Materials for fusion, with special reference to the spherical tokamak design
Fusion energy systems present unique materials challenges. Extreme temperatures, intense levels of radiation damage, rapid sputtering of plasma-facing surfaces, tritium retention and transmutation of elements within key engineering structures are just a few of the difficulties that need to be managed. Approaches include the use of highly refractory materials, materials that have the potential to self heal, and the use of liquid layers to absorb damage. Spherical tokamak designs which incorporate superconducting magnets present unique issues. The sections of the toroidal field coils that lie within the central column are more exposed to heat and radiation than in a ring-shaped torus design. The superconducting magnets require careful shielding from radiation damage, and this shielding must be compact, yet highly efficient. Recent progress with the development of cerment-based shielding materials will be described. A further issue with fusion engineering is the lack of a well-developed regulatory environment. It not yet clear what standards will be required to be met in order to obtain an operating licence for such a plant. This is an issue which needs to be addressed as soon as possible, otherwise progress in the field may be inhibited. ![]() Professor George Smith FRS, Trinity College Oxford, UK
![]() Professor George Smith FRS, Trinity College Oxford, UKProfessor George Smith, FRS, FIMMM, FInstP, FRSC, CEng, is a materials scientist with special interest in the study of the materials at the atomic level, particularly materials for energy systems. From 2000 - 2005 he was Head of the Department of Materials at Oxford University. He was a member of the MatUK Energy Materials Working Group, and led the writing of their report for the DTI on Nuclear Energy Materials. He has particular interests in the long-term safety and stability of the materials used in current-generation nuclear reactors, and those planned for use in future fusion energy systems. He retired from his academic post in Oxford in 2010, but continues to be active in research as an Emeritus Professor of Materials, and acts as an external consultant to a private sector fusion energy company, Tokamak Energy Ltd. |
|
12:15 - 12:30 | Discussion |
Chair
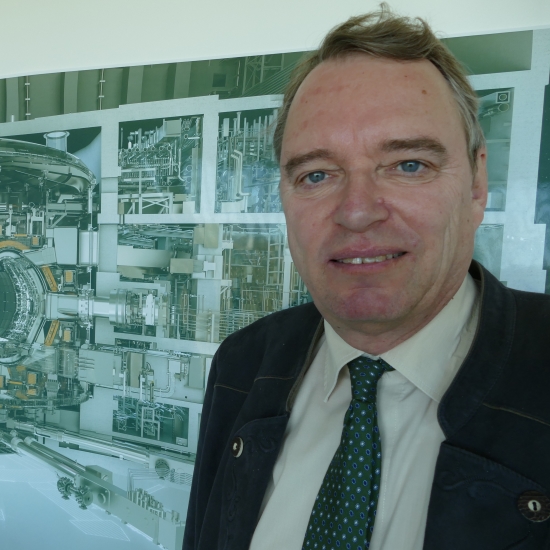
Professor Guenter Janeschitz, ITER Organisation, France
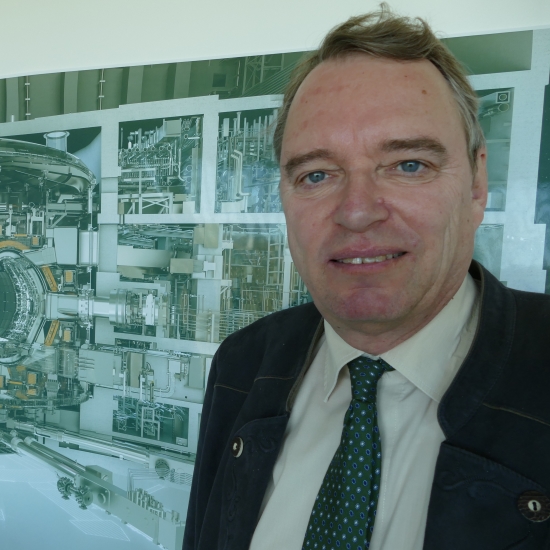
Professor Guenter Janeschitz, ITER Organisation, France
Professor Guenter Janeschitz received his Diplom Ingenieure from the Technical University Vienna, and his PhD in physics from the Atominstitut at the Technical University Vienna in 1983. Following his PhD studies, he had various roles at IPP Garching and then spent a year with General Atomics in San Diego. In 1989 he returned to Europe to join the JET programme, becoming Head of the Fusion Programme in FZK in 2002. He moved to the ITER Organisation in 2008, and has been Deputy Head of the ITER Central Integration Office since 2015.
13:30 - 14:00 |
What is the value proposition of fusion energy?
Fusion faster? If we had a working DEMO fusion plant today, how would it fare in the dynamic of the energy transition? What would it offer to the world that cannot be obtained cheaper and/or more reliably from the combination of existing carbon-free technologies: generation, conversion and storage? Why would governments invest in First Generation (Gen1) fusion plants? These questions can be analysed, even if we don’t yet know if the outstanding technical issues of fusion energy will be solved. Because of the large overnight investment cost, the intrinsic complexity of the reactor and the long construction time, Gen1 fusion power plants will have a high financial and technical risk profile. Moreover, the first fusion power plants are likely to have a low availability, even apart from the shut down periods for divertor and blanket replacement. So, it is far from obvious that a DEMO today would present a compelling business case. However, we don’t have a working DEMO plant today. The ITER – DEMO roadmap leads to a first generation of fusion plants towards 2070. To put this into perspective: 10 Gen1 fusion power plants represent an effective electric power output comparable to wind power around 2000. Before we address the question how we can develop fusion power faster, we need to articulate why we develop fusion power in the first place: the value proposition. Or else be satisfied with the academic challenge: can we make it work? and worry about the economics when we get there. ![]() Professor Niek Lopes Cardozo, Eindhoven University of Technology, The Netherlands
![]() Professor Niek Lopes Cardozo, Eindhoven University of Technology, The NetherlandsNiek Lopes Cardozo is professor of 'Science and Technology of Nuclear Fusion' in the Applied Physics department of Eindhoven University of Technology. He has more than 35 years experience in fusion research, authored 100+ papers and supervised 30+ PhD theses. Former leader of the Dutch fusion research programme (2001-2009), he was member of the top-level European fusion governance committees, including as vice-chair of the Governing Board of the European Domestic Agency for ITER ‘Fusion for Energy’. He received the 2003 Koninklijke/Shell prize for sustainable development and energy research. His group runs an interdisciplinary 2-year Fusion Master program, with 20+ international students per year. He has initiated the European Fusion Education Network FUSENET (60+ members) and the consortium ITER-NL, in which Dutch research institutes collaborate with industry for the ITER project. As major responsibility beside his university position, he chaired the Dutch Organisation for Fundamental Research on Matter (FOM), the physics branch of the national science council NWO, from 2010 to 2017. In that role he also chaired the interdisciplinary NWO research theme Sustainable Energy and was member of the leading energy related committees in the Netherlands. In recent years his research focus has been on the prospects of fusion energy, in view of the outstanding technical challenges on the one hand and the progress in other energy technologies on the other. |
|
---|---|---|
14:00 - 14:15 | Discussion | |
14:15 - 14:45 |
The economics of size
The cost of energy produced by fusion, like fission or, indeed, other non-carbon sources, will be dominated by servicing the capital charge. Therefore, a fusion power plant must maximise the output for the capital spent, the plant availability and its lifetime in order that the cost of energy is minimised. Experience with fission power plants has demonstrated that the scale of investment should be a fraction of the annual turnover of the operating companies so that the commercial risk is reduced and the decision-taking process expedited. This, together with the ‘Learning Curve’ cost reductions arising from Many Of A Kind construction, is the primary driver for the interest in Small Modular Reactors. Fusion has advantages in respect of safety, long term environmental impact and plentiful, well distributed fuel. The implications for the fusion load assembly requirements are: that it should have ‘high capital efficiency’, or a high value of the ratio of average plasma pressure to magnetic field pressure(β); that it should have the lowest possible, ‘recirculating’ power required to keep it operating by maximising the plasma current driven by the plasma pressure (‘bootstrap current’); that the plasma current during burn not depend on transformer action; and that it should be of a physical size that factory production of all the major components be possible, so that the ‘Learning Curve’ economies be realised. This would also reduce maintenance duration and the cost of the associating tooling. The talk will describe a concept for Modular Fusion[1] power plants that, in principle, satisfies all these requirements using Spherical Tokamaks, with High Temperature Superconducting magnets. Clearly, these requirements are best met if the fusion load assemblies are as physically small as possible. However, the most significant ‘size’ of the paper’s title is that of the capital investment. Preliminary cost estimates of Modular Fusion plants, based on Tokamak Energy’s approach, indicate that the requirement on economic ‘size’ can be met. [1] MP Chuyanov and MP Gryaznevich, Fusion Engineering and Design 122(2017)p238 ![]() Dr Paul Thomas, Tokamak Energy Ltd, UK
![]() Dr Paul Thomas, Tokamak Energy Ltd, UKDr Thomas obtained a PhD in high energy theory from University of Manchester in 1974. After post-docs in University of California Irvine and Cambridge University, he joined the UKAEA in 1978. In 1980, he joined the JET team and left as acting Deputy Director in 2000. Dr Thomas then went to the CEA, Centre d'Etudes at Cadarache, where he worked on Tore-Supra. In 2008, he was appointed Head of Heating, Diagnostics and Controls at Fusion for Energy in Barcelona. In 2010, Dr Thomas returned to Cadarache to join the ITER Team as Head of Heating and Current Drive Division. He was appointed to the post Director of the CHD Department from which he retired in 2015. Since then, Dr Thomas has been a consultant at Tokamak Energy. |
|
14:45 - 15:00 | Discussion |
Chair
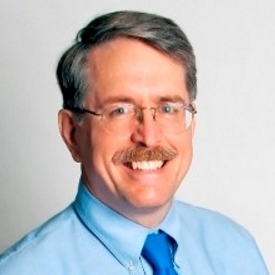
Dr Michael C Zarnstorff, Princeton Plasma Physics Laboratory, USA
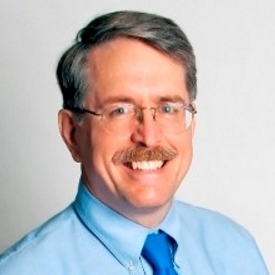
Dr Michael C Zarnstorff, Princeton Plasma Physics Laboratory, USA
Michael Zarnstorff is the Deputy Director for Research at the Princeton Plasma Physics Laboratory. He is an experimental plasma physicist with interests in the physics of plasma confinement and configuration optimization. Mike received his PhD in Physics from the University of Wisconsin-Madison (1984), was named a PPPL Distinguished Research Fellow in 1995, and taught in the Astrophysics Department at Princeton University. In 2008, he received the APS Dawson Award for Excellence In Plasma Physics Research. His research included the first observation and systematic study of the bootstrap current, investigations of neoclassical and turbulent transport, transport barriers, and the confinement and stability of different magnetic field configurations. He authored or co-authored over 400 research publications. He led the National Compact Stellarator Experiment physics group, was one of the leaders of the TFTR research program, and collaborated on tokamak and stellarator experiments in the US, Germany, Japan, and the UK. Mike is a Fellow of the American Physics Society and has served as a Division of Plasma Physics Distinguished Lecturer.
15:30 - 16:30 | Open discussion: a road map to accelerated fusion | |
---|---|---|
16:30 - 17:00 |
Summary and closing remarks
![]() Professor Colin Windsor FRS, Tokamak Energy UK Ltd, UK
![]() Professor Colin Windsor FRS, Tokamak Energy UK Ltd, UKColin Windsor studied at Oxford, gaining his DPhil in 1963, and was a post-doctorial fellow at Yale. Joining Harwell he performed experiments on neutron scattering for many years before joining Culham Fusion Laboratory in 1994. He worked on neural net control of the COMPASS-D tokamak, on the spherical tokamak START and on the JET tritium campaign of 1997. In retirement he has used neural networks for disruption prediction, mine detection using radar, and for signature recognition. He is a Fellow of the Royal Society (1995), the Institute of Non-Destructive Testing (1994), and the Institute of Physics (1975) who in 1986 awarded him their Duddell medal for developments in instrumentation. In 1990 he was made an Honorary Professor of Physics at Birmingham University. In 2013 he became a consultant to Tokamak Energy, a private company dedicated to accelerating the development of fusion power. He has worked on the key problem of the centre column shield neutronics with papers on the energy deposition, the shield modelling and the radiation damage. ![]() Matt Ridley, House of Lords, UK
![]() Matt Ridley, House of Lords, UKMatt Ridley's books have sold over a million copies, been translated into 31 languages and won several awards. His books include The Red Queen, The Origins of Virtue, Genome, Nature via Nurture, Francis Crick, The Rational Optimist and The Evolution of Everything. His TED talk "When Ideas Have Sex" has been viewed more than two million times. He writes a weekly column in The Times (London) and writes regularly for the Wall Street Journal. He was elected to the House of Lords in February 2013. He served on the science and technology select committee 2014-2017 and is currently on the ad-hoc committee on artificial intelligence. |