Links to external sources may no longer work as intended. The content may not represent the latest thinking in this area or the Society’s current position on the topic.
Quantum light for investigating complex molecules and materials
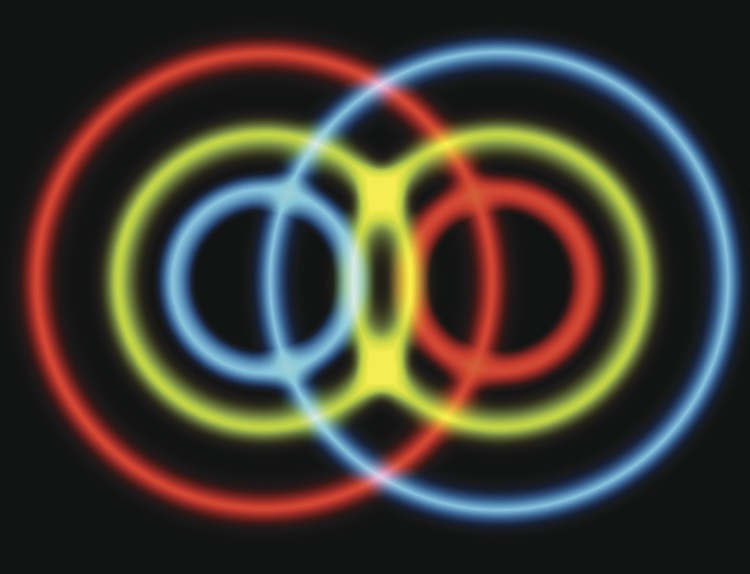
Theo Murphy international scientific meeting organised by Professor Alexandra Olaya-Castro, Professor Shaul Mukamel and Professor Graham Fleming FRS.
Quantum light such as entangled photon pairs is a key resource for quantum technologies but its applications have a much wider scope. This meeting aimed to bridge and accelerate the developments in the fields of quantum optics and spectroscopy by discussing the fundamentally new opportunities that quantum light opens in the investigation of ultrafast phenomena in complex molecules and materials.
Recorded audio of the presentations will be available on this page soon.
Enquiries: contact the Scientific Programmes team
Organisers
Schedule
Chair
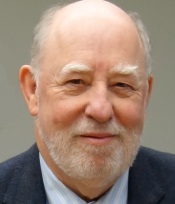
Professor Graham Fleming FRS, University of California Berkeley, USA
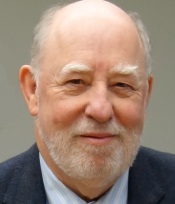
Professor Graham Fleming FRS, University of California Berkeley, USA
Graham Fleming is Professor of Chemistry at the University of California, Berkeley. He is a leading expert in Ultrafast Spectroscopy and its application to chemical, biological and material systems. He has had an extensive career in administrative positions including Vice Chancellor for Research (UC Berkeley) and Deputy Laboratory Director (Lawrence Berkeley National Laboratory). He has published more than 525 papers, is a member of the National Academy of Science, the American Philosophical Society, a fellow of the Royal Society, the American Academy of Arts and Science and a Foreign fellow of the Indian National Science Academy.
09:00 - 09:05 | Welcome by the Royal Society and lead organiser | |
---|---|---|
09:05 - 09:35 |
Novel nonlinear spectroscopy and imaging of molecules with quantum light
Quantum light opens up new avenues for spectroscopy by utilizing parameters of the quantum state of light as control knobs and through the variation of photon statistics by coupling to matter. Nonlinear optical signals induced by quantized light fields and entangled photon pairs will be surveyed and classified. When a molecule interacts with an external field, the phase information is imprinted in the state of the field in a detectable way. Entangled-photon pairs are not subjected to the classical Fourier limitations on their joint temporal and spectral resolution. Combined time and frequency resolution not possible by classical light can therefore be achieved. A novel quantum diffraction-based imaging technique whereby one photon of an entangled pair is diffracted of a sample and detected in coincidence with its twin is presented. Imaging is possible with weak quantum fields, avoiding damage to delicate biological samples. Strong coupling of molecules to the quantum vacuum field of micro cavities that can be used to manipulate their photophysical and photochemical reaction pathways and polariton relaxation are demonstrated. For photosynthetic antennae. [1] “Quantum phase-sensitive diffraction and imaging using entangled photons”, Shahaf Asban, Konstantin E. Dorfman, and, Shaul Mukamel. PNAS (2019) 116, 11673-11678 ![]() Professor Shaul Mukamel, University of California Irvine, USA
![]() Professor Shaul Mukamel, University of California Irvine, USAShaul Mukamel, currently the Chancellor Professor of Chemistry at the University of California, Irvine, received his Ph.D. in 1976 from Tel Aviv University. Following postdoctoral appointments at MIT and the University of California, Berkeley, he has held faculty positions at Rice University, the Weizmann Institute, and the University of Rochester. He is the recipient of the Sloan, Dreyfus, Guggenheim, and Alexander von Humboldt Senior Scientist award, the OSA Lippincort Award and the APS Plyler Award for Molecular Spectroscopy. He is a fellow of the American Physical Society and the Optical Society of America. His interests focus on developing computational techniques for the design of novel ultrafast laser pulse sequences for probing electronic and vibrational dynamics in molecules. Biophysical applications include folding and dynamical fluctuations in proteins, hydrogen bonding, long-range electron and energy transfer in photosynthetic complexes, and signatures of chirality. Other areas are attosecond x-ray spectroscopy, excitons in semiconductor nanostructures, many-body effects in quantum optics. He is the author of over 650 publications in scientific journals and the textbook, Principles of Nonlinear Optical Spectroscopy (Oxford University Press, 1995). |
|
09:35 - 09:50 | Discussion | |
09:50 - 10:20 |
Sub shot noise transmission spectroscopy and imaging with correlated photon pairs
Optics is routinely used to measure physical parameters. Quantum mechanics can describe how each strategy is limited in precision, as quantified by the resources used to perform each type of optical measurement — the fundamental noise floor of optical measurements using a laser is the shot noise limit. Application of quantum states of light can reduce noise for optical measurement below this limit. In this presentation, I will discuss recent demonstrations using correlated photon pairs to reach and surpass this limit in practice for transmission estimation [1,2], and how it can be applied to transmission spectroscopy [3] and imaging [4]. We will discuss how resources are accounted in experiments, the physical principles used and we will cover steps being taken to take the proof of principle post-selected experiments into practical use outside of the quantum optics laboratory, including a recent work on passive noise suppression of pulsed fibre laser emission to the shot noise limit [5]. References ![]() Dr Jonathan Matthews, Department of Physics, University of Bristol, UK
![]() Dr Jonathan Matthews, Department of Physics, University of Bristol, UKJonathan Matthews is an Associate Professor in Quantum Technology at QETLabs (University of Bristol) where he leads a quantum enhanced sensing activity. His early research efforts focused on integrated quantum photonics, e.g. silica waveguides to control photonic quantum states for primitive quantum information demonstrators and higher refractive index contrast waveguide arrays for multi-photon quantum walks. His current efforts focus on discrete and continuous variables strategies to perform quantum sensing and imaging, and the development of CV capabilities in silicon photonics. His research is supported by an EPSRC early career fellowship, an ERC starting grant and the Quantic quantum-imaging hub. |
|
10:20 - 10:35 | Discussion | |
10:35 - 11:00 | Coffee break | |
11:00 - 11:30 |
Quantum light spectroscopy in organic and biological molecules
This talk will focus on the investigations of nonlinear optical effects utilizing quantum light. Illustrations of absorption and fluorescence of the entangle two photon process in organic and biological systems will be presented. Investigations of the entanglement characteristics and their effect on the nonlinear excitation process will be discussed. The results and discussion will be directed toward further understanding of the nature of the interaction of entangled light with organic matter with a view of possible applications. ![]() Professor Ted Goodson, Department of Chemistry, University of Michigan, USA
![]() Professor Ted Goodson, Department of Chemistry, University of Michigan, USATheodore Goodson III (b: April 5, 1969) received his BA in liberal arts from Wabash College in 1991 and his PhD from the University of Nebraska-Lincoln in 1996. He was a postdoctoral assistant at the University of Chicago and Postdoctoral fellow at Oxford University (physics). He served on the faculty at Wayne State University from 1998 to 2004 and moved to the University of Michigan where he is the Richard Barry Bernstein Professor of Chemistry and Professor of Applied Physics. Dr. Goodson’s research centers on the investigation of ultra-fast, nonlinear optical, and quantum optical properties in organic multi-chromophore and metal cluster systems for particular optical and electronic applications in the condensed phase. This has included contributions to the understanding of ultra-fast exciton migration in organic dendrimers and novel molecular aggregates, correlations of ultra-fast dynamics in organic polymers with photovoltaic performance, the nature of ultra-fast electronic processes in in small (magic number) metal clusters, ultra-fast two photon effects for the determination of DNA-drug binding modes, ultra-fast nonlinear optical detection of remote IEDs, ultra-fast processes of the aggregation process in the formation of amyloids, the interaction of organic molecules with non-classical (entangled) light, the quantum optical response of biological macromolecules. His research has been translated in to technology in the areas of two-photon organic materials for eye and sensor protection, large dielectric and energy storage effects in organic macromolecular materials, the remote detection of energetic (explosive) devices by nonlinear optical methods, quantum optical communications and remote chemical sensing, tissue imaging and photodynamic therapy with small metal clusters. He has published over 170 scientific papers and one book (Solar Fuels) and has given more than 250 invited talks. Professor Goodson has been awarded numerous awards including the Distinguished University Professor Award, the National Science Foundation American Innovation Fellowship, The Percy Julian Award (NOBCChE), Fellow of the American Chemical Society, Army Young Investigator, National Science Foundation CAREER Award, Alfred P. Sloan Research Fellowship, Camille Dreyfus Teacher-Scholar Award to name a few. He has been a Senior Editor for The Journal of Physical Chemistry since 2007. He has done extensive work with the University of Michigan President’s office to enhance efforts for diversity in the STEM fields at the University of Michigan and beyond. |
|
11:30 - 11:45 | Discussion | |
11:45 - 12:15 |
Challenges in measurement of two-photon absorption cross-sections with nonclassical light
We are investigating methods to advance the accuracy and reliability of molecular spectroscopy with nonclassical light. Molecular two-photon absorption (TPA) of correlated photon pairs produced by spontaneous parametric down-conversion (SPDC) is expected to occur in a linear excitation regime, and has been reported to occur at many orders of magnitude lower photon flux than classical TPA. A major challenge in observing nonclassical excitation of molecules is to discriminate TPA from one-photon losses. Standard transmittance measurements are insufficient because single-photon loss due to scattering, absorption, or misalignment all scale linearly with flux. In contrast, a distinction between TPA and single-photon loss in a sample can be made by measuring changes in the photon statistics of the transmitted beam. The second order intensity correlation function, g(2), is insensitive to linear losses, yet changes in g(2) are related to the strength of the two-photon interaction. We performed comprehensive transmittance experiments for Zinc tetraphenylporphyrin (ZnTPP) in solution using an 810 nm pulsed SPDC source. Prima facie, the transmittance changes are consistent with a cross section of ~10-17 cm2. However, we observed only a weak dependence on signal-idler photon time delay and a small change in g(2), indicating that the cross section for correlated TPA by ZnTPP is much smaller than previously reported. ![]() Dr Ralph Jimenez, JILA, National Institute of Standards and Technology, Boulder, USA
![]() Dr Ralph Jimenez, JILA, National Institute of Standards and Technology, Boulder, USARalph Jimenez earned his PhD in Physical Chemistry at the University of Chicago and was a postdoc at the University of California, San Diego and a Senior Research Associate at the Scripps Research Institute before joining NIST and JILA in 2003. His research focuses on investigating the photophysics of fluorescent proteins and biosensors and the directed evolution of their photophysical properties by combining protein engineering methods with microfluidics technologies. Recently, his group has been exploring the potential for quantum measurement techniques to enhance the sensitivity and information content of molecular spectroscopy and imaging. |
|
12:15 - 12:30 | Discussion |
Chair
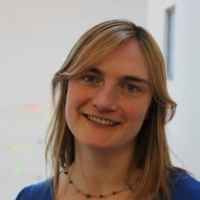
Professor Ruth Oulton, University of Bristol, UK
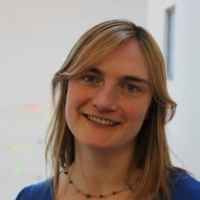
Professor Ruth Oulton, University of Bristol, UK
Ruth Oulton’s field of research involves the study of nanoscale semiconductor devices that enable the exchange of “quantum” information between a single electron and a single photon. The idea is that they can use the rules of quantum mechanics to perform computing and measurements in a completely new way. She take ideas from quantum theory and information science, bring them together with what we are beginning to understand about semiconductors on the nanoscale, and to make working quantum devices that engineers will use as part of their everyday toolkit. In her recent work she studies single electron spins in atomic-like systems, and studies how the angular momentum of the photon and spin exchange information, and how photonic design can influence this. In other interdisciplinary side projects she studies the role of photonic structures in plants such as seaweed and begonias.
13:30 - 14:00 |
Manipulating the character and shape of ultrashort quantum light states
A fully controlled state and mode engineering of nonclassical light is one of the major challenges in the path of future quantum technologies. ![]() Dr Marco Bellini, National Institute of Optics (CNR-INO), Italy
![]() Dr Marco Bellini, National Institute of Optics (CNR-INO), ItalyMarco Bellini is a Research Director at the Istituto Nazionale di Ottica (CNR-INO) in Florence, Italy. Although his main current interests are in the field of Quantum Optics, in the course of his scientific career he has been involved in very different experimental activities, ranging from high-precision atomic and molecular spectroscopy in the far infrared, to coherent sources in the extreme ultraviolet. He is an expert in frontier experimental research with ultrashort laser pulses, both in the regime of high-intensity laser-matter interactions, and in that of quantum effects with single-photon-level light intensities. Some of his main achievements were in the field of supercontinuum and high-order harmonic generation (his collaboration with Professor TW Hänsch contributed to the invention of the optical frequency comb and to the 2005 Nobel Prize in Physics), and in the production and characterization of nonclassical light states for fundamental tests. |
|
---|---|---|
14:00 - 14:15 | Discussion | |
14:15 - 14:45 |
Exploiting quantum correlations for spectroscopy, imaging and remote sensing
In this talk I will review recent work on sensing technologies focussing on pair photon correlations applied to rangefinding and remote sensing. These experiments primarily exploit the time (energy) correlations between photons [1] and were recently popularised as quantum illumination [2]. I will review recent experimental results showing the possibility of 3D imaging and covert rangefinding using parametric pair photon sources. I will also review work towards practical photon limited gas sensing using near infra-red wavelengths [3] and extensions to longer wavelengths using non-linear interferometry [4] in bulk and chip scale experiments [5, 6]. [1] J.G. Rarity et al Applied Optics 29, 2939 (1990). [2] S. Lloyd, Science 321, 1463 (2008). [3] M Quatrevalet, JGR, et al, IEEE J. Selected Topics in Quantum Electronics 23, 157 (2017). [4] T.J. Herzog, JGR et al Physical Review Letters, 72, 629 (1994). [5] T. Ono, JGR et al, Optics letters 44 (5), 1277-1280 (2019). [6] L. Rosenfield JGR et al arXiv:1906.10158. ![]() Professor John Rarity FRS, University of Bristol, UK
![]() Professor John Rarity FRS, University of Bristol, UKProfessor John Rarity FRS is head of Quantum Engineering Technology Labs (Physics and E&EE) and Photonics Group (E&EE) at the University of Bristol (since 2003). He is an international expert on quantum optics, communications and sensing exploiting single photons and entanglement. He pioneered early experiments in path entanglement, quantum key distribution and quantum metrology. Current research focusses on non-linear waveguide sources of pair photons, integrated quantum photonics, sub-shot noise imaging schemes, long wavelength quantum sensing, free space quantum communications, multi-photon entanglement and nanocavities for spin photon interfaces. |
|
14:45 - 15:00 | Discussion | |
15:00 - 15:30 | Tea break | |
15:30 - 16:00 |
Infrared metrology with visible light
Infrared (IR) optical range is important for material characterization and sensing. Also, imaging in the IR range yields superior image contrast due to a significant reduction of scattering losses. Thus IR metrology is widely used in petrochemical, pharma, biomedical, homeland security, and other areas. [1] D. Kalashnikov, A. Paterova, S. Kulik, L. Krivitsky, Nature Photonics 10, 98 (2016). [2] A. Paterova, S. Lung, D. Kalashnikov, L. Krivitsky, Scientific reports 7, 42608 (2017). [3] A. Paterova, H. Yang, Ch. An, D. Kalashnikov, L. Krivitsky, New Journal of Physics 20, 043015 (2018). [4] A. Paterova, H. Yang, Ch. An, D. Kalashnikov, L. Krivitsky, Quantum Science & Technology 3, 025008 (2018). [5] A. Paterova, H. Yang, C. An, D. Kalashnikov, L. Krivitsky, Opt. Express 27, 2589-2603 (2019). ![]() Dr Leonid Krivitskiy, Agency for Science, Technology and Research (A*STAR), Singapore
![]() Dr Leonid Krivitskiy, Agency for Science, Technology and Research (A*STAR), SingaporeLeonid started his professional career in 2003 as a postdoctoral researcher at the National Metrology Institute (Turin, Italy). In 2005 he received Alexander von Humboldt fellowship and moved to Max Planck Institute for the Science of Light (Erlangen, Germany). In 2006 he received the H.C. Oersted fellowship at Technical University of Denmark (Lyngby, Denmark). In 2008 he started his group at Agency for Science Technology and Research (A*STAR) in Singapore being awarded A*STAR investigatorship grant. His research interests include quantum and nonlinear optics, quantum information processing, and quantum metrology. Leonid holds MSc and PhD degrees from Lomonosov Moscow State University and MBA degree from the National University of Singapore. |
|
16:00 - 16:15 | Discussion | |
16:15 - 16:45 |
Single photon spectroscopy of atoms and solids
The absorption and reflection of light allows us to determine the properties of the world around us. Alex S Clark will present two recent experiments using single photons to perform absorption spectroscopy. The first uses photons generated by four-wave mixing in optical fiber. Two pump photons (~750nm) are annihilated to simultaneously generate time-correlated pairs of photons at widely spaced wavelengths (~650nm signal and ~900nm idler). The researchers tune the pump wavelength to scan idler photons across the absorption band-edge of gallium arsenide placed in the idler beam path. The transmission of the idler photons alone does not reveal the absorption due to overlapping Raman noise, but when correlated with detected signal photons, the absorption is revealed, a form of ‘ghost-spectroscopy’. The second experiment uses a bright pump laser to saturate rubidium atoms in a vapour cell, while a counter-propagating and cross-polarized single photon level probe reveals narrow transmission peaks corresponding to the hyperfine excited state energy levels of the atoms. The pump and probe are independently tunable, meaning atoms moving in all directions can be addressed by compensating the counter-propagating optical frequencies for the relevant Doppler shifts. This experiment forms a basis for building an atomic quantum memory. ![]() Dr Alex S Clark, Imperial College London, UK
![]() Dr Alex S Clark, Imperial College London, UKDr Alex S Clark is a Royal Society University Research Fellow working on organic molecular quantum photonics at Imperial College London. He heads the Quantum Nanophotonics Project in the Centre for Cold Matter, is Work Package Leader on Interfaces in the EPSRC Programme Grant Quantum Science with Ultracold Molecules (QSUM) and leads a team working on coupling molecules to integrated waveguides and cavities in the QuantERA Consortium Organic Quantum Integrated Devices (ORQUID). His current research interests lie in the use of molecules to build new quantum technology and explore quantum science, including creating on-demand photon sources, quantum memories, photonic quantum gates and hybrid interfaces to link disparate quantum systems. Dr Clark has recently joined QUANTIC - the UK Quantum Hub for Imaging, in which he is working on imaging biological cells using induced coherence and nonlinear interferometry. |
|
16:45 - 17:00 | Discussion | |
17:00 - 18:15 | Poster session |
Chair
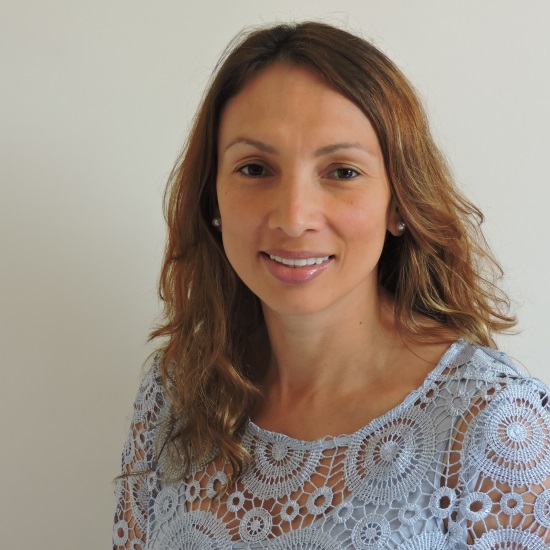
Professor Alexandra Olaya-Castro, University College London (UCL), UK
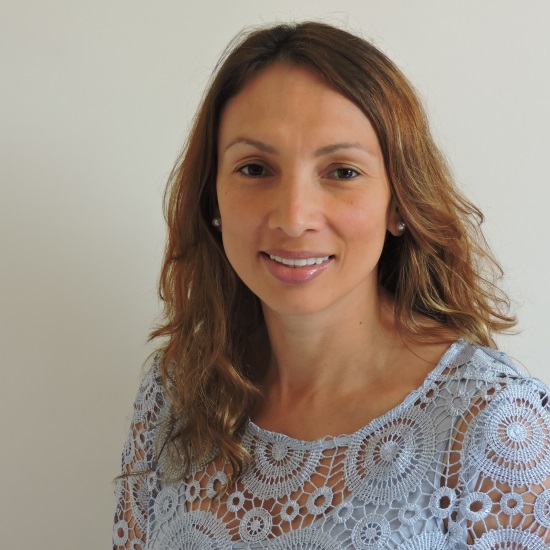
Professor Alexandra Olaya-Castro, University College London (UCL), UK
Alexandra Olaya-Castro earned a DPhil in Physics from the University of Oxford in 2006 and subsequently obtained a Junior Research Fellowship at Trinity College, University of Oxford. In 2008 she moved to University College London with an EPSRC Career Acceleration Fellowship. She became a Lecturer in 2011, was promoted to Reader in 2015 and became a Professor of Physics in 2018. Her research interests are focused on theoretical approaches to bridge quantum science and biophysics. She is a leading expert in the area of quantum effects in biomolecules and her theoretical contributions to this field made her the recipient of the 2016 Maxwell Medal and Prize by the Institute of Physics in London.
09:00 - 09:30 |
TBC
|
|
---|---|---|
09:30 - 09:45 | Discussion | |
09:45 - 10:15 |
Quantum Spectroscopy with entangled photon absorption and emission
Quantum spectroscopy aims to exploit quantum properties of radiation. The use of entangled photons promises nonlinear spectroscopies at low photon fluxes and the circumvention of certain classical Fourier uncertainties that restrict the simultaneous time and frequency resolution. Much less attention has been paid to the spectroscopic information contained in the light emitted from a molecular sample. In this talk, we propose photon correlation measurements as a new spectroscopic tool. We will analyse the information contained in photon correlation measurements of a tractable model system, where we show that these can signal the presence of quantum coherence. Deviations from the counting statistics of independent emitters constitute a direct fingerprint of quantum coherence in the steady state. In contrast to typical approaches of two-dimensional spectroscopy, which rely in the use of fast coherent pulses, the proposed method can be employed in stationary setups in which the system is driven by thermal light, enabling measurements under the natural conditions of solar light-harvesting. Furthermore, we show that, even when coherence is washed out in the stationary state, coherent dynamics can still be evidenced by frequency-resolved counting statistics. ![]() Dr Frank Schlawin, Department of Physics, University of Oxford, UK
![]() Dr Frank Schlawin, Department of Physics, University of Oxford, UKI completed my PhD at the University of California, Irvine, under the supervision of Prof. Shaul Mukamel, and at the University of Freiburg, Germany, under the supervision of Prof. Andreas Buchleitner, from which I received my degree. In 2015, I joined the group of Prof. Dieter Jaksch at the University of Oxford. My research interests span current research areas concerning the interaction of quantum light with complex quantum systems. Since the time of my PhD, I work on the theory of nonlinear spectroscopy with quantum light. I am particularly interested in the role of photonic quantum correlations in absorption processes, and how these can be employed for spectroscopic applications. Besides, I am also interested in how quantum light can be employed to manipulate material properties when these are strongly coupled to optical cavities. |
|
10:15 - 10:30 | Discussion | |
10:30 - 11:00 | Coffee break | |
11:00 - 11:30 |
Nonlinear multi wave mixing spectroscopy with quantum light
The progress in quantum optics utilizes a unique photon state configuration for engineering of the ultimate light-matter interactions with relatively simple material systems (qubits). It results in a broad range of applications including radiation sources, quantum communication, information, computing and nanotechnology. The development of the ultrafast multidimensional nonlinear spectroscopy that has been enabled by progress in ultrafast optical technology provides a unique tool for probing complex molecules, semiconductors, nanomaterials by classical light fields. In particular squeezed light generated in four-wave mixing experiments provide to be an exciting multiphoton analogue of the single photon sources that have robust quantum correlations. In this talk I reviewed our recent results in the field of nonlinear spectroscopy with quantum light. In particular, I show how quantum squeezing generation in the four-wave mixing can be used as a multidimensional spectroscopy tool for probing Brillouin and Raman scattering. We further show how various interferometers such as cascading and SU(1,1) commonly used in quantum information theory provide an additional degree of control of spectroscopic signals with enhanced resolution. Our theory, which contains both microscopic treatment of the squeezing generation as well as noise treatment, is in a good agreement with the recent experiments in hot rubidium vapor. ![]() Professor Konstantin Dorfman, State Key Laboratory of Precision Spectroscopy, East China Normal University, China
![]() Professor Konstantin Dorfman, State Key Laboratory of Precision Spectroscopy, East China Normal University, ChinaKonstantin E Dorfman was born in Russia. He completed his BS degree in Physics from Nizhny Novgorod State University, Russia, in 2006. In 2009 he completed his PhD degree at Texas A&M University. From 2009 to 2015 he worked as a postdoctoral scholar at Princeton University, Texas A&M University, University of California, Irvine, and a staff scientist in Singapore Agency for Science, Technology and Research (A*STAR). Dr Dorfman is currently a professor of physics at State Key Laboratory of Precision Spectroscopy, East China Normal University, Shanghai. His scientific interests include atomic, molecular, optical, and chemical physics, quantum and nonlinear optics and photonics, X-ray optics, ultrafast phenomena, and quantum thermodynamics. |
|
11:30 - 11:45 | Discussion | |
11:45 - 12:15 |
Understanding the quantum efficiency of light harvesting photosynthetic systems one photon at a time
Photosynthetic light harvesting of plants and bacteria in vivo is characterized by the remarkable capability of producing electron-hole pairs from absorbed photons with near unit quantum efficiency under weak illumination conditions. This quantum efficiency is usually measured from bulk kinetic studies. While ultrafast spectroscopic studies have revealed much insight into the microscopic dynamics of excitonic energy transport that follows the initial process of photoexcitation, the microscopic dynamics and energetics of the initial absorption of photons from the sun’s radiation field are still poorly understood. Yet these are essential components for a complete and consistent microscopic understanding of the dynamical processes underlying the quantum efficiency for conversion of the energy of a single photon to an electron-hole pair. I shall present a theoretical analysis of scattering/absorption of states of light with exactly one photon from a realistic light harvesting system. The analysis leads to calculation of transmission, fluorescence, and absorption probabilities for comparison with experiments using quantum light sources. Our approach yields the relations between output and input photon fluxes, in addition to probabilities of excitation and excitonic energy transport in the light harvesting complex, including the effects of phonons in the latter, i.e., the vibrations of the chromophores and their protein environment. While the basic theory employs a density matrix approach, producing average excitation probabilities for different excitonic states as well as photon fluxes for transmission and fluorescence, I shall also present a theory for calculation of individual quantum trajectories based on the detection and counting of fluorescent photons. ![]() Professor Birgitta Whaley, Department of Chemistry, University of California Berkely, USA
![]() Professor Birgitta Whaley, Department of Chemistry, University of California Berkely, USABirgitta Whaley is Professor of Chemistry at the University of California, Berkeley and co-Director of the Berkeley Quantum Information and Computation Center. Whaley holds a PhD from the University of Chicago and was a Postdoctoral Fellow at Hebrew University Jerusalem and Tel Aviv University. Whaley's research lies at the interface between quantum physics and chemistry and biology. Her work is broadly focused on quantum information and quantum computation, quantum control and simulation of complex quantum systems, with particular focus on implementation of quantum information processing, and on quantum effects in biological systems. Professor Whaley is a member of the American Academy of Arts and Sciences, and of the International Academy of Molecular Quantum Science. She is a Fellow of the American Physical Society, as well as recipient of awards from Bergmann, Sloan, and Alexander von Humboldt Foundations, and senior Fellowships at the Miller Institute (Berkeley) and the Institute for Advanced Studies in Berlin. |
|
12:15 - 12:30 | Discussion |
Chair
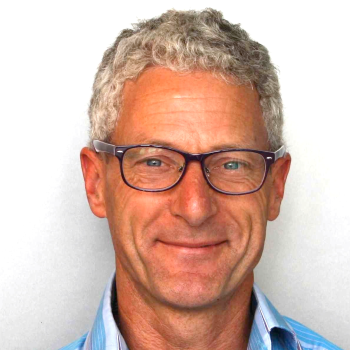
Professor Bill Barnes, University of Exeter, UK and University of Twente, the Netherlands
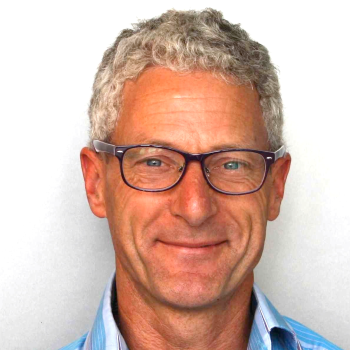
Professor Bill Barnes, University of Exeter, UK and University of Twente, the Netherlands
Professor Bill Barnes has been fascinated by the interaction between molecules and light since his PhD (1983-86). Much of his work has concerned changing the way molecules absorb, emit and transfer energy in the form of light. His research is primarily experimental in nature, involving the fabrication and study of molecules confined to well-defined nanostructures. Now he is particularly concerned with looking at how light may be used to link molecules together in new ways, especially because such an approach enables a radical modification of molecular properties. His interests extend widely, he has been involved in projects spanning physical geography, manuscript studies and astrophysics.
13:30 - 14:00 |
Cavity-engineering of molecular and materials properties from first principles QEDFT
Computer simulations that predict the light-induced change in the physical and chemical properties of complex systems usually ignore the quantum nature of light. Here we will show the effects of quantum-photons can be included in the newly developed quantum electrodynamics density-functional formalism (QEDFT). We provide an overview of how well-established concepts in the fields of quantum chemistry and material sciences have to be adapted when the quantum nature of light becomes important and present the novel framework we have developed of quantum electrodynamics density-functional formalism (QEDFT). We illustrate the method to molecular complexes identifying fundamental changes in the Born-Oppenheimer surfaces, conical intersections (chemical reactivity and energy transfer and spectroscopy. We also show how periodic driving of many-body interacting 2D systems allow to design Floquet states of matter with tunable electronic properties on ultrafast time scales (and cavity induced-topology). This work paves the road for the development of what can be coined as QED-materials and QED-chemistry. ![]() Professor Angel Rubio, Max Plank Institute for Structure and Dynamics of Matter at Hamburg, Germany
![]() Professor Angel Rubio, Max Plank Institute for Structure and Dynamics of Matter at Hamburg, GermanyAngel Rubio, born on 27 September 1965 in Oviedo, Spain, is the Director of the Theory Department of the Max Planck Institute for the Structure and Dynamics of Matter. He is also Distinguished Research Scientist at the Simons Foundation Flatiron Institute (NY, USA) and Professor/Chair for condensed matter physics at the University of the Basque Country in Donostia-San Sebastián, Spain. He received his PhD in Physics in 1991 from the University of Valladolid. He worked as post-doctoral researcher at University of California at Berkeley (1992-94). He is External Scientific Member of the Fritz Haber Institute in Berlin and Full Professor at the University of Hamburg (2016). He is acknowledged as a pioneer and leader in the area of computational materials physics, one of the founders of modern 'theoretical spectroscopy', and the originator of the widely used ab initio open-source project Octopus. Recently his research has focussed on the prediction and characterization of new non-equilibrium states of matter. His work has been recognized by several awards, including the 2018 Max Born medal and prize, 2016 Medal of the Spanish Royal Physical Society, the 2014 Premio Rey Jaime I for basic research, the 2006 DuPont Prize in nanotechnology, the 2005 Friedrich Wilhelm Bessel Research Award of the Humboldt Foundation, and two European Research Council advanced grants (2011 and 2016). Rubio is Fellow of the American Physical Society and the American Association for the Advancement of Science, member of the Academia Europaea, and a foreign associate member of the National Academy of Sciences. |
|
---|---|---|
14:00 - 14:15 | Discussion | |
14:15 - 14:45 |
Interacting a handful of molecules and photons: nonlinear optics and polaritonic states
The interaction of light and matter at the nanometer scale lies at the heart of quantum optics because it concerns elementary processes such as absorption or emission of a photon by an atom. Over the past decade, we have shown that direct coupling of a photon to a single two-level atom should be possible via tight focusing. However, because transitions in quantum emitters are typically not closed, laboratory demonstrations of this idea fall short of the theoretical prediction. I shall report on recent achievements, where the branching ratio of a single organic molecule is improved by a substantial Purcell effect when coupled to a microcavity. Furthermore, we will discuss extension of the coherent linear and nonlinear experiments that become accessible in this regime to molecules coupled to subwavelength waveguides. Indeed, many interesting proposals in quantum optics and light-matter interaction rely on having multiple quantum emitters well-coupled to a single mode of light. We show that one-dimensional (1D) photonic channels including microresonator elements on a chip are ideal for coupling a well-defined number of identical emitters and indistinguishable photons. These developments make organic molecules viable candidates for the realization of novel superposition states of light and matter as well as integration in chip-based quantum optical circuits. ![]() Professor Vahid Sandoghdar, Max Planck Institute for the Science of Light, Germany
![]() Professor Vahid Sandoghdar, Max Planck Institute for the Science of Light, GermanyVahid Sandoghdar is one of the pioneers of the field of Nano-Optics, which merges various methods and research areas to investigate fundamental issues in the interaction between light and matter at the nanometer scale. His current research ranges from quantum optics, plasmonics and ultrahigh resolution microscopy to nanobiophysics. Sandoghdar obtained his B.S. in physics from the University of California at Davis in 1987 and Ph.D. in physics from Yale University in 1993. After a postdoctoral stay at the Ecole Normale Supérieure in Paris he moved to the University of Konstanz in Germany, where he started a new line of research to combine single molecule spectroscopy, scanning probe microscopy and quantum optics. In 2001, he accepted a chair at the Laboratory of Physical Chemistry at ETH in Zurich, Switzerland. In 2011, he became director at the newly established Max Planck Institute for the Science of Light in Erlangen and Alexander von Humboldt Professor at the University of Erlangen-Nuremberg in Germany. Vahid Sandoghdar is the founder of the new Max-Planck-Zentrum für Physik und Medizin, a joint research center that aims to address questions in fundamental medical research with physical and mathematical methods. |
|
14:45 - 15:00 | Discussion | |
15:00 - 15:30 | Tea break | |
15:30 - 16:00 |
The interface between chemistry and QED: cavity control of chemical transformations
At the interface of chemistry and quantum optics, recent interest in strong light-matter coupling has led to a rapid convergence of these two historically very different fields. Various experiments have explored the new regime of polaritonic chemistry, where matter and the electromagnetic degrees of freedom become equally important. In chemistry, the idea of altering molecular processes by strongly coupling to the vacuum fluctuations of the electromagnetic field has been suggested and demonstrated. Yet, the theoretical fundamentals of how the chemical landscape and induced dynamical processes are changed under strong light-matter coupling has remained elusive. In this context, I will discuss a new formalism at the intersection of cavity quantum-electrodynamics and electronic structure methods, quantum-electrodynamical density functional theory (QEDFT), to treat electrons, photons and phonons on the same quantized footing. So far, QEDFT studies have usually considered lossless cavities. Realistic cavities, however, exhibit finite photon lifetimes that lead to incoherent phenomena that may dominate the dynamics of the light-matter excitations. Therefore we have extended the QEDFT description to explicitly consider lossy (and very lossy) cavity modes. This allows us to perform first-principles studies of light-matter interactions ranging from the weak-coupling regime to the strong-coupling regime where hybrid light-matter polariton states are formed. We show that by correctly tuning the coupling it is possible to achieve cavity-mediated energy transfer between electronic excited states of the molecules. Further, I will introduce a new method to calculate polaritonic excited-state potential-energy surfaces for strongly coupled light-matter systems, we show how this coupling can be exploited to alter photochemical reaction pathways by influencing avoided crossings. Finally, using this ab initio understanding and new theoretical and computational methods, I will discuss a general methodology for optical control of chemical dynamics. ![]() Professor Prineha Narang, John A Paulson School of Engineering and Applied Sciences, Harvard University, USA
![]() Professor Prineha Narang, John A Paulson School of Engineering and Applied Sciences, Harvard University, USAPrineha Narang is a Professor at the John A Paulson School of Engineering and Applied Sciences at Harvard University. Prineha’s work has been recognized by many awards and special designations, including being named a Moore Inventor Fellow by the Gordon and Betty Moore Foundation for innovations in quantum science and technology, CIFAR Azrieli Global Scholar by the Canadian Institute for Advanced Research, a Top Innovator by MIT Tech Review (MIT TR35), and a Young Scientist by the World Economic Forum in 2018. In 2017, she was named by Forbes Magazine on their '30 under 30' list for her work in atom-by-atom quantum engineering. Prineha designs materials at the smallest scale, using single atoms, to enable the leap to quantum technologies. She has pioneered work in classical and quantum computing to approach problems in physics and chemistry with a new toolkit. At SEAS she designed and teaches ES 170, a popular undergraduate class on quantum engineering. Outside of science, she is an avid triathlete and runner. |
|
16:00 - 16:15 | Discussion | |
16:15 - 16:45 |
Spectroscopy at the atomic scale by using x-ray spontaneous parametric down conversion
Nonlinear interactions between x-rays and long wavelength radiation can be used as a powerful atomic scale probe for light-matter interactions and for properties of valence electrons [1-3]. The x-rays provide the high resolution whereas the optical/UV photons interact strongly with the valence electrons and provide the spectroscopic information. This probe can provide novel microscopic data in solids that are inaccessible by any existing method, hence to advance the understanding of many phenomena. Spontaneous parametric down conversion is a second order nonlinear optical effect, which is driven by the vacuum fluctuations. As such, the process requires only one input x-ray beam and it can be used as a probe at wavelengths where the material is opaque. The simple setup, the broad spectral range it spans, and the ability to reveal spectral information combined with structural information makes the effect of spontaneous parametric down conversion from x-ray into long wavelength radiation very unique.In my talk I will describe the recent progress in the study of x-ray into long wavelength radiation spontaneous parametric down conversion. I will discuss our new insight on the effect, the challenges, and the expected future developments. I will elaborate on our recent observation of the very strong nonlinearity in non-centrosymmetric crystals [4] and discuss the implications of this observation.
1. T. E. Glover et al. Nature 488, 603 (2012) 2. K. Tamasaku et al. Nat. Phys. 7, 705 (2011) 3. A . Schori et al. Phys. Rev. Lett. 119, 253902 (2017) 4. S. Sofer et al. arXiv:1904.13146 ![]() Professor Sharon Shwartz, Bar Ilan University, Israel
![]() Professor Sharon Shwartz, Bar Ilan University, IsraelSharon Shwartz received his BA and PhD from the Technion, Israel, in 2001 and 2008, respectively. He was a postdoctoral fellow at Stanford University from 2008 until 2012. In 2012 Sharon Shwartz returned to Israel and joined the Physics department and the Institute of Nanotechnology and Advanced Materials at Bar Ilan University as a faculty member. In 2018 he was appointed as Associate Professor. His research focuses on nonlinear, ultrafast, and quantum phenomena with x-rays and on their applications for imaging and spectroscopy. Shwartz has led and participated in several pioneering experiments in the fields of nonlinear and quantum optics with x-rays using x-ray sources ranging from x-ray tubes to x-ray free-electron lasers. |
|
16:45 - 17:00 | Discussion | |
17:00 - 17:15 | Final remarks |