Links to external sources may no longer work as intended. The content may not represent the latest thinking in this area or the Society’s current position on the topic.
Anhydrobiosis – cheating death and telling the tale
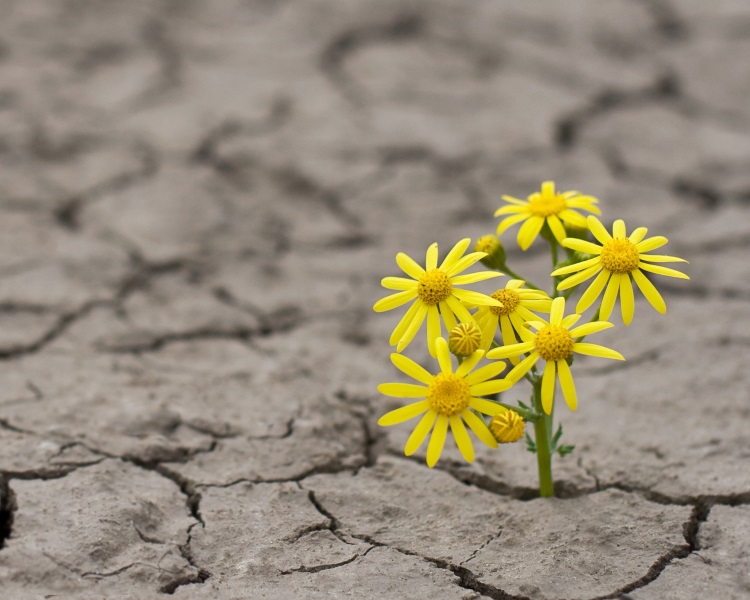
Theo Murphy meeting organised by Professor Roy Quinlan and Professor Takekazu Kunieda.
Anhydrobiosis was first described by Van Leeuwenhoek in 1702. Both plants and animals have anhydrobiotic responses and understanding the mechanisms that support such an extreme stress response will prove invaluable for future food security and for new therapeutics to treat for example proteinopathy-based diseases. Interdisciplinary scientists at the plant-animal interface scoped the application of anhydrobiotic mechanisms in future technologies.
Click watch on YouTube to view the full video playlist.
Organisers
Schedule
Chair
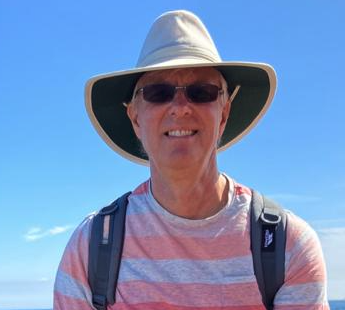
Professor Roy Quinlan, University of Durham, UK
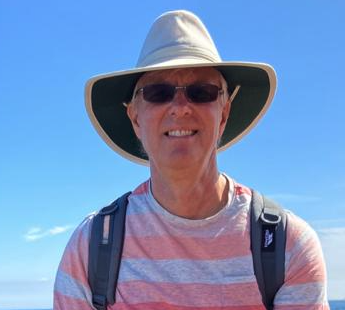
Professor Roy Quinlan, University of Durham, UK
Roy Quinlan is emeritus professor of biomedical sciences at the University of Durham (UK). His interests in the cytoskeleton, protein chaperones, the eye lens and cataractogenesis were the starting point to organising this conference. Liquid Liquid Phase Separation (LLPS) of the crystallins and their association with the cytoskeleton underpins transparency and optical function in the eye. The lens is also particularly sensitive to ionising radiation. The fact that some animals, such as the tardigrade Ramazzottius varieornatus, is very radioresistant and had an interesting cytoskeleton-chaperone complement was a watershed moment for me (pun intended) and where my research career was always heading had I but realised it sooner! This remarkable system demanded my attention. An inter-disciplinary, multi-system conference on plant and animal anhydrobiosis was the only way to encourage and promote this most remarkable of life-preserving events.
12:00 - 12:05 |
Session 1 - Animal anhydrobiosis, the proteins and their assemblies.
Anhydrobiosis was first described by Antonie Van Leeuwenhoek in 1702 in a lecture to the Royal Society. Seeing life emerge before your very eyes from a desiccated animal or plant is one of the most incredible biological events to fascinate, bewilder and motivate scientists. The future of our planet depends on tapping into such life-preserving responses as we face man-made extremes in climate change. Whilst all life can elicit a stress response, anhydrobiosis is the ultimate. From the study of “resurrection” plants trehalose was discovered. Both plants and animals also use proteins rich in Intrinsically Disordered Domains (IDDs) to preserve life when water is removed. Liquid-Liquid phase separation is a key to both protection strategies. Comparing the different anhydrobiotic responses of plants and animals will deliver benefits for both food security and disease prevention/treatment. There has never been a more important time to build collaborative links across disciplines and across continents to understand and exploit the biology, chemistry and physics of anhydrobiosis. ![]() Professor Roy Quinlan, University of Durham, UK
![]() Professor Roy Quinlan, University of Durham, UKRoy Quinlan is emeritus professor of biomedical sciences at the University of Durham (UK). His interests in the cytoskeleton, protein chaperones, the eye lens and cataractogenesis were the starting point to organising this conference. Liquid Liquid Phase Separation (LLPS) of the crystallins and their association with the cytoskeleton underpins transparency and optical function in the eye. The lens is also particularly sensitive to ionising radiation. The fact that some animals, such as the tardigrade Ramazzottius varieornatus, is very radioresistant and had an interesting cytoskeleton-chaperone complement was a watershed moment for me (pun intended) and where my research career was always heading had I but realised it sooner! This remarkable system demanded my attention. An inter-disciplinary, multi-system conference on plant and animal anhydrobiosis was the only way to encourage and promote this most remarkable of life-preserving events. |
---|---|
12:05 - 12:30 |
Unique toolbox in tardigrade anhydrobiosis
Tardigrades are known for their anhydrobiotic ability and the resilience against various extreme stresses. The recent efforts to find the tolerance-related factors identified multiple tardigrade-unique protein families. For example, tardigrade DNA protection protein dubbed Dsup is scarcely conserved in other organisms but can protect DNA from hazardous irradiation and oxidative stress and enhances the radio resistance of human cultured cells. These findings placed tardigrade-unique proteins as important clues supporting tolerant ability of tardigrades, which are even functional in other organisms. Many of tardigrade tolerance proteins including Dsup were found to be soluble even after boiling possibly through their hydrophilic nature with resulting unstructured flexibility. Recently, the relationship between stress response and the condensation of unstructured proteins attracts attention and many tardigrade proteins have been identified through the screening of stress-dependent condensing proteins. Some of them are revealed to form cytoskeleton-like filamentous network in animal cells in response to hyperosmosis and these transitions are demonstrated to stiffen the cells and enable the cells to counteract deformation stress and to retain cell integrity during dehydration process. Stress-dependent change of the physical property of cells can be achieved through reversible protein condensation and it contributes for the resistance against stress. ![]() Dr Takekazu Kunieda, University of Tokyo, Japan
![]() Dr Takekazu Kunieda, University of Tokyo, JapanTakekazu Kunieda is the Associate Professor at Graduate School of Science, University of Tokyo since 2018. He received his PhD in Pharmaceutical Sciences from University of Tokyo on the regeneration of fly appendages. After he worked on fly developmental biology as a postdoc at University of Basel, Switzerland, he backed to University of Tokyo and were engaged in the research on the organ regeneration of frog and the social behaviors of honeybee. He started the study on the anhydrobiosis and the extremotolerance of tardigrades since 2004. His research team has conducted genome, transcriptome, biochemical, cell biological and biophysical study to elucidate the molecular mechanisms and the principle underlying tardigrade anhydrobiosis and extremotolerance. His team also aims to confer the resilient ability to other organisms, already with a partial success. Takekazu Kunieda is the Associate Professor at Graduate School of Science, University of Tokyo since 2018. He received his PhD in Pharmaceutical Sciences from University of Tokyo on the regeneration of fly appendages. After he worked on fly developmental biology as a postdoc at University of Basel, Switzerland, he backed to University of Tokyo and were engaged in the research on the organ regeneration of frog and the social behaviours of honeybees. He started the study on the anhydrobiosis and the extremotolerance of tardigrades since 2004. His research team has conducted genome, transcriptome, biochemical, cell biological and biophysical study to elucidate the molecular mechanisms and the principle underlying tardigrade anhydrobiosis and extremotolerance. His team also aims to confer the resilient ability to other organisms, already with a partial success. |
12:30 - 12:55 |
Anhydrobiotic midge and its dry-preservable cultured cell line
Anhydrobiosis represents an extreme example of tolerance adaptation to water loss. Polypedilum vanderplanki is the unique insect known to be capable of anhydrobiosis. Trehalose, which accumulates in the larvae up to 20% of the dry body mass, is thought to replace the water in its tissues. Similarly, highly hydrophilic proteins called the late embryogenesis abundant (LEA) proteins are expressed in huge quantities and act as a protectant for biological molecules against aggregation and denaturation. However, transduction of trehalose and LEA proteins in desiccation-sensitive cells did not improve viability after long-term storage of the dried cells. These findings suggest other factors must be involved in induction of anhydrobiosis. The genome of the anhydrobiotic midge specifically contains clusters of multi-copy genes with products, which act as desiccation protectants. A cell line derived from the midge, Pv11, showing ability of complete desiccation tolerance was established. Since gene knock-down and knock-out systems for Pv11 cells have been developed, this cell line can be used as a tool to investigate the anhydrobiosis at the molecular level. Here current knowledge of molecular mechanisms underlying the anhydrobiosis in P. vanderplanki will be discussed.Anhydrobiosis represents an extreme example of tolerance adaptation to water loss. Polypedilum vanderplanki is the unique insect known to be capable of anhydrobiosis. Trehalose, which accumulates in the larvae up to 20% of the dry body mass, is thought to replace the water in its tissues. Similarly, highly hydrophilic proteins called the late embryogenesis abundant (LEA) proteins are expressed in huge quantities and act as a protectant for biological molecules against aggregation and denaturation. However, transduction of trehalose and LEA proteins in desiccation-sensitive cells did not improve viability after long-term storage of the dried cells. These findings suggest other factors must be involved in induction of anhydrobiosis. The genome of the anhydrobiotic midge specifically contains clusters of multi-copy genes with products, which act as desiccation protectants. A cell line derived from the midge, Pv11, showing ability of complete desiccation tolerance was established. Since gene knock-down and knock-out systems for Pv11 cells have been developed, this cell line can be used as a tool to investigate the anhydrobiosis at the molecular level. Here current knowledge of molecular mechanisms underlying the anhydrobiosis in P. vanderplanki will be discussed. ![]() Dr Takahiro Kikawada, University of Tokyo - Graduate School of Frontier Sciences, Japan
![]() Dr Takahiro Kikawada, University of Tokyo - Graduate School of Frontier Sciences, JapanSince 2000, Dr Takahiro Kikawada has been studying the molecular mechanism underlying anhydrobiosis in Polypedilum vanderplanki, a unique insect with the capability to survive severe drought. In the progress of the research, he identified important genes and metabolites involved in the desiccation tolerance. Currently, in collaboration with many research institutes in Japan and overseas, he is trying to get closer to the secret of the desiccation tolerance by combining cell engineering techniques such as genomics and genome editing technology using the dry-preservable cultured cell line (Pv11 cells) derived from the anhydrobiotic midge. Meanwhile, he is developing a technology for dry preservation of proteins and cells at room temperature by applying the molecular mechanisms underlying the desiccation tolerance. |
12:55 - 13:20 | Poster session |
13:20 - 13:25 | Break |
13:25 - 13:50 |
A novel tertiary structure model for ab-initio interpretation of BioSaxs data
Small angle x-ray scattering is one of the most flexible and readily available experimental methods for obtaining information on the structure of proteins in solution. In the advent of powerful predictive methods such as the alphaFold and rossettaFold algorithms, this information has become increasingly in demand, owing to the need to characterise the more flexible and varying components of proteins which resist characterisation by these and more standard experimental techniques. To deal with structures about little of which is known a parsimonious method of representing the tertiary fold of a protein backbone as a discrete curve has been developed. It represents the fundamental Ramachandran constraints through a pair of parameters and is able to generate millions of potentially realistic portent geometries from only a sequence in a short space of time. The method for using this model to interpret BioSaxs data will be introduced. A particular focus will be given to how the model can additionally be linked to established protein modelling techniques and additional data sources of ab-intio protein structure, in order to boost the methods predictive efficacy. ![]() Dr Christopher Prior, Durham University, UK
![]() Dr Christopher Prior, Durham University, UKChristopher obtained his PhD from university college London, studying and developing the topological measures to characterise entangled curves, and the applications of these methods in solar physics and biophysics. After a post-doctoral position modelling biopolymer at Oxford university, he obtained a fellowship at Durham university developing various long running projects which have formed the basis for his long-term interests. He is now an associate professor in the department of mathematical sciences at Durham university. He is interested in systems which can be described by entangled bundles of curves and how this entanglement plays a fundamental role in their development and organisation. This includes protein structure identification and characterisation, and the effect on solar weather by the changing entanglement of complex entangled magnetic fieldline structures. |
13:50 - 14:15 |
Biophysical principles of molecular chaperone self- and co-assembly
We have been developing and applying quantitive mass-measurement approaches to interrogate directly the structure and dynamics of proteins. Here I will focus on the insights this has enabled in studying the evolution of specificity in assembly of molecular chaperone proteins, and the implications this has with respect to their key role in protecting the cell under times of acute and chronic stress. ![]() Dr Justin Benesch, University of Oxford, UK
![]() Dr Justin Benesch, University of Oxford, UKJustin’s research has garnered an international reputation for innovative biophysical chemistry approaches based on combining mass measurement with other experimental methods, simulations, and quantitative thermodynamic and kinetic analyses. This has allowed him and his group to change our thinking as to how proteins assemble, interact, and even evolve. After a degree in Chemistry at the University of Oxford, Justin obtained his PhD from the University of Cambridge for the development and application of novel mass spectrometry approaches working with Professor Dame Carol Robinson FRS. He was awarded fellowships from the Medical Research Council and Royal Society, and appointed to faculty at the University of Oxford in 2012, where he is now Professor in Biophysical Chemistry, and Tutorial Fellow in Physical Chemistry at University College. Justin has been recognised by the Cell Stress Society International with the Alfred Tissières Award, the Howard Prize Lecture from the Biophysical Sciences Institute at Durham, and the Norman Heatley Award from the Royal Society of Chemistry in 2019. His group’s research impacts broadly the interface between chemistry and the life sciences. Their insights have been important to understanding molecular chaperone (mal)function in humans, and the stress tolerance of plants; and their innovations in mass measurement approaches have provided new means for researchers to quantify biomolecules and their interactions. He currently leads two major initiatives in developing a next generation of proteomics approaches. |
14:15 - 14:40 |
Phase separation as a stress survival strategy
Biomolecular condensates formed by phase separation are membraneless compartments in the cytoplasm and nucleoplasm of cells, which have major roles in cellular organization and physiology. RNP granules are a specific type of condensate that assemble from RNA-binding proteins and RNA. In this talk, I will discuss how the concept of biomolecular condensates has expanded our view of RNP granules and their link to the cellular stress response. I will introduce in vitro reconstitution systems based on the concept of phase separation that now allow us to reconstruct RNP granules in the test tube. Using these reconstitution systems as well as cell biological and genetic approaches, we have gained important insights into the molecular rules of RNP granule assembly, such as the driving forces and amino acids that govern condensation, the conformational changes underlying assembly and molecular mechanisms of condensate regulation and control. I will further discuss how the concept of phase separation has allowed us to dissect the functions of RNP granules, and I will demonstrate how condensate formation can be used by cells to sense and respond to changes in the environment and regulate fundamental cellular processes such as protein synthesis. ![]() Professor Simon Alberti, Technische Universität Dresden, Germany
![]() Professor Simon Alberti, Technische Universität Dresden, GermanySimon Alberti is Professor of Cellular Biochemistry at the Technical University Dresden, Biotechnology Center (BIOTEC), Center for Molecular and Cellular Bioengineering (CMCB). He received his PhD in 2004 in Biology from the University of Bonn, Germany. In 2005 he joined the lab of Susan Lindquist at the Whitehead Institute for Biomedical Research (Cambridge, USA), where he worked on prions and amyloids. From 2010 to 2019 he worked as a group leader at the Max Planck Institute of Molecular Cell Biology and Genetics in Dresden, Germany, where he studied proteostasis and the molecular principles underlying cytoplasmic organization. |
14:40 - 15:05 |
Liquid-Liquid Phase Separation of Anhydrobiosis-Related Proteins from Artemia franciscana
The brine shrimp Artemia franciscana expresses Late Embryogenesis Abundant proteins from three groups in their anhydrobiotic life-history stage and is the only animal known to express LEA proteins belonging to groups 1 (LEA_5) and 6 (SMP) in addition to group 3 (LEA_4) proteins. The reason for the more extensive LEA repertoire in brine shrimp compared to other anhydrobiotic members in this kingdom of life is unknown. The proteins AfrLEA6 and AfLEA1 are mostly intrinsically disordered in the hydrated state, and both proteins form biomolecular condensates driven by the consensus sequence motifs attributed to the LEA_5 and SMP families. Interestingly, RNA promotes LLPS only for AfLEA1 while AfrLEA6 droplets incorporate target proteins based on their surface charge. The role of proteinaceous LLPS in cellular stress responses has been firmly established in several models and includes heat shock and osmotic stress, but its role in animal anhydrobiosis is less well defined. Based on these findings, it is still premature to conclude that most proteins from the LEA_5 and SMP families will form biomolecular condensates during desiccation stress. Nevertheless, forming target-specific protective compartments via LLPS constitutes an additional feature in the growing protection repertoire of this exciting family of proteins. ![]() Michael A. Menze, Professor and Associate Dean for Research and Innovation, University of Louisville, Louisville, KY, USA
![]() Michael A. Menze, Professor and Associate Dean for Research and Innovation, University of Louisville, Louisville, KY, USADr Michael A. Menze is a Professor in the Biology Department at the University of Louisville. He earned his doctoral degree under the guidance of Dr Grieshaber from the University of Duesseldorf in collaboration with Dr Decker’s group at the Institute for Molecular Biophysics at the University of Mainz. His graduate work elucidated the role of allosteric regulation of respiratory pigments in the hypoxia tolerance of crustaceans. His postdoctoral work with Dr Steven Hand at Louisiana State University and Mehmet Toner at Harvard Medical School focused on the mechanisms of water-stress tolerance in invertebrates. Intrigued by the exceptional stress resistance of the brine shrimp Artemia franciscana, he investigated the role of compatible organic osmolytes and proteaceous protectants in biostabilization. Dr Menze continues to investigate the molecular basis of anhydrobiosis in animals and develops biomimetics approaches to improve the long-term preservation of biologics. |
15:05 - 15:10 | Break |
15:10 - 15:25 |
Discussion
![]() Professor Roy Quinlan, University of Durham, UK
![]() Professor Roy Quinlan, University of Durham, UKRoy Quinlan is emeritus professor of biomedical sciences at the University of Durham (UK). His interests in the cytoskeleton, protein chaperones, the eye lens and cataractogenesis were the starting point to organising this conference. Liquid Liquid Phase Separation (LLPS) of the crystallins and their association with the cytoskeleton underpins transparency and optical function in the eye. The lens is also particularly sensitive to ionising radiation. The fact that some animals, such as the tardigrade Ramazzottius varieornatus, is very radioresistant and had an interesting cytoskeleton-chaperone complement was a watershed moment for me (pun intended) and where my research career was always heading had I but realised it sooner! This remarkable system demanded my attention. An inter-disciplinary, multi-system conference on plant and animal anhydrobiosis was the only way to encourage and promote this most remarkable of life-preserving events. |
Chair
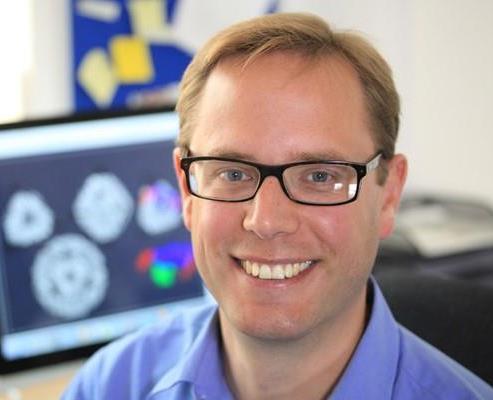
Dr Justin Benesch, University of Oxford, UK
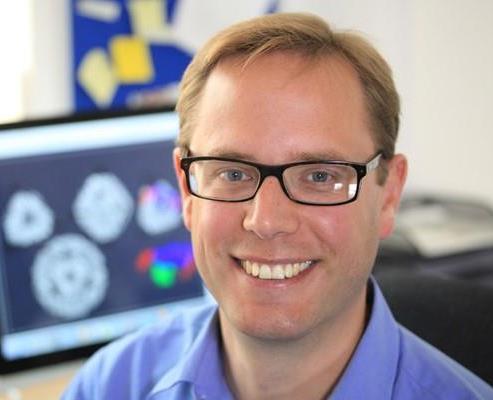
Dr Justin Benesch, University of Oxford, UK
Justin’s research has garnered an international reputation for innovative biophysical chemistry approaches based on combining mass measurement with other experimental methods, simulations, and quantitative thermodynamic and kinetic analyses. This has allowed him and his group to change our thinking as to how proteins assemble, interact, and even evolve.
After a degree in Chemistry at the University of Oxford, Justin obtained his PhD from the University of Cambridge for the development and application of novel mass spectrometry approaches working with Professor Dame Carol Robinson FRS. He was awarded fellowships from the Medical Research Council and Royal Society, and appointed to faculty at the University of Oxford in 2012, where he is now Professor in Biophysical Chemistry, and Tutorial Fellow in Physical Chemistry at University College. Justin has been recognised by the Cell Stress Society International with the Alfred Tissières Award, the Howard Prize Lecture from the Biophysical Sciences Institute at Durham, and the Norman Heatley Award from the Royal Society of Chemistry in 2019.
His group’s research impacts broadly the interface between chemistry and the life sciences. Their insights have been important to understanding molecular chaperone (mal)function in humans, and the stress tolerance of plants; and their innovations in mass measurement approaches have provided new means for researchers to quantify biomolecules and their interactions. He currently leads two major initiatives in developing a next generation of proteomics approaches.
12:00 - 12:25 |
Calcium signalling in response to stress in plants
Abiotic and biotic environmental stimuli are sensed and transduced by signalling networks in plants leading to an appropriate pattern of protective gene expression. My lab is interested in how calcium, involved in response to so many different primary signals, can encode specific information to elicit the correct downstream responses. The calcium signature hypothesis states that different external stimuli elicit unique spatiotemporal patterns of elevations in cellular calcium concentration and thus encode stimulus-specific information that is “read” by plant cells. Through a combination of experimental and mathematical approaches, we have determined how calcium signatures are “decoded” by specific transcription factors to lead to appropriate specific gene expression responses. Our most recent work has found that unique calcium signatures occur when different stresses are applied simultaneously or sequentially, or when a single stress is applied under different environmental conditions. These signatures represent integrated information obtained from different environmental cues together, and are decoded to produce unique gene expression “decisions”. ![]() Professor Marc Knight, Durham University, UK
![]() Professor Marc Knight, Durham University, UKMarc Knight is currently the Chair in Plant Cell Signalling at the Department of Biosciences, Durham University, England, UK, since March 2006, when he moved from the University of Oxford. He earned his BSc in Botany with honours from the University of Durham (1986) and then PhD in plant biochemistry from the University of Glasgow (1990). He then undertook postdoctoral studies at the University of Edinburgh in the lab of Prof Tony Trewavas (1989- 1993) which established his research interest in calcium signalling. In 1993 he was awarded a University Research Fellowship from the Royal Society to establish his own research group at the Department of Plant Sciences at the University of Oxford, where he developed his interest in cold hardiness. Marc has published 89 peer-reviewed research papers, accounting for over 18,000 citations. His current research interests are the role of calcium signalling in conveying information to regulate stress gene expression, and the molecular/mechanistic basis of plant freezing tolerance. |
---|---|
12:25 - 12:50 |
How do angiosperm plants survive desiccation of vegetative tissues using Craterostigma plantagineum as a model plant?
The objective is to understand how angiosperm plants can survive long periods without water. Resurrection plants are a small group of angiosperm plants which tolerate severe water loss, and adjust their water content with the relative humidity in the environment. The plants can remain in the desiccated state for several months. When rainfall occurs, the plants recover and assume full physiological activity. The studies are focused on the desiccation tolerant resurrection plants Craterostigma plantagineum and Lindernia brevidens. Genome and transcriptome sequences are available and are compared with the sequences from the desiccation sensitive close relative Lindernia subrasemosa. This comparative approach demonstrates that expression levels of genes encoding protective molecules including proteins and carbohydrate metabolites are differentially regulated in desiccation tolerant and non-tolerant plants. These genes are excellent candidates to identify essential molecular components of desiccation tolerance in angiosperm plants. It is suggested that desiccation tolerance has evolved through expressing components of seed desiccation pathways in vegetative tissues.The objective is to understand how angiosperm plants can survive long periods without water. Resurrection plants are a small group of angiosperm plants which tolerate severe water loss, and adjust their water content with the relative humidity in the environment. The plants can remain in the desiccated state for several months. When rainfall occurs, the plants recover and assume full physiological activity. The studies are focused on the desiccation tolerant resurrection plants Craterostigma plantagineum and Lindernia brevidens. Genome and transcriptome sequences are available and are compared with the sequences from the desiccation sensitive close relative Lindernia subrasemosa. This comparative approach demonstrates that expression levels of genes encoding protective molecules including proteins and carbohydrate metabolites are differentially regulated in desiccation tolerant and non-tolerant plants. These genes are excellent candidates to identify essential molecular components of desiccation tolerance in angiosperm plants. It is suggested that desiccation tolerance has evolved through expressing components of seed desiccation pathways in vegetative tissues. ![]() Professor Dorothea Bartels, University of Bonn, Germany
![]() Professor Dorothea Bartels, University of Bonn, GermanyDorothea Bartels is a professor at the University of Bonn. She studied biology and chemistry at the University of Hannover, Germany. She obtained her doctoral degree in Botany from the University of Hannover, Germany. She worked for several years as a postdoc at the Plant Breeding Institute, Cambridge, UK. Then she became a group leader at the Max Planck Institute for Plant Breeding in Cologne, Germany when she started to work on stress in plants. This was followed by a professorship at the University of Bonn which was succeeded by a professorship at the Vrije Universiteit, Amsterdam, NL and finally she returned to a professorship for physiology and biochemistry of plants at the University of the main research interests are molecular stress physiology in higher plants and particularly anhydrobiosis and how plants adapt to environments with water deficit. She is editor in chief of the international journal Planta, she is an EMBO member and a member of the Academy of Mainz.Bonn. |
12:50 - 13:20 |
Identification of genes important for drought tolerance in non-model species
Exploitation of comparative genomics in an evolutionary framework has allowed us to understand the modes of genome and gene family evolution to identify novel stress response genes across the plant kingdom. We have shown that the evolutionary history of plants was shaped by two bursts of unprecedented genomic novelty linked to multicellularity and terrestrialisation, and that gene expansion and co-option are the more common mechanisms of biological innovation in the evolution of features enabling water uptake and transport. Specifically, by incorporating trait evolution (drought adaptation) into a comparative genomics framework, we identified different patterns of gene family evolution in species with opposing stress phenotypes. For example, the C3 desert species, Rhazya stricta (R. stricta) maintains high photosynthetic rates despite extreme environmental conditions. Gene families specific to R. stricta, such as photosynthesis and respiration associated genes responded to diurnal temperature changes and water limitation. By combining transcriptomics and evolutionary genomics, we showed that specific genes have diverged from their homologs in other species. Furthermore, we quantified gene loss in drought sensitive compared to closely related drought tolerant species, and together these analyses may provide useful targets for improving tolerance to extreme environments. ![]() Professor Ulrika Bechtold, University of Essex, UK
![]() Professor Ulrika Bechtold, University of Essex, UKProfessor Ulrike Bechtold studied biology at the University of Giessen in Germany, and in 1998 moved to the UK to study for a PhD at the John Innes Centre in Norwich. She started her research career characterising a family of enzymes that reverse a specific post-translational modification, in Arabidopsis. After 7 years as a postdoc, she started her academic career as a Lecturer in 2011 at the University of Essex, and since 2019, was a Senior Lecturer and Director of Recruitment at the University of Essex. She recently moved to the Durham University to take up a position as Associate Professor of Plant Molecular Biology in March 2022. Her main research focuses on abiotic stress, specifically the integration of plant growth and stress signalling pathways in response to drought stress. In recent years she has also become interested in the evolution and comparative genomics of stress tolerance traits. Ulrike studied biology at the University of Giessen in Germany, and in 1998 moved to the UK to study for a PhD at the John Innes Centre in Norwich. She started her research career characterising a family of enzymes that reverse a specific post-translational modification, in Arabidopsis. After 7 years as a postdoc, she started her academic career as a Lecturer in 2011 at the University of Essex, and since 2019, was a Senior Lecturer and Director of Recruitment at the University of Essex. She recently moved to the Durham University to take up a position as Associate Professor of Plant Molecular Biology in March 2022. Her main research focuses on abiotic stress, specifically the integration of plant growth and stress signalling pathways in response to drought stress. In recent years she has also become interested in the evolution and comparative genomics of stress tolerance traits. |
13:20 - 13:45 | Poster session |
13:45 - 13:50 | Break |
13:50 - 14:15 |
Phase behaviours of unfolded proteins reveal novel twists to the unfolded protein response
Protein homeostasis involves regulation of the concentrations of unfolded states of globular proteins. Dysregulation can cause phase separation leading to protein-rich deposits. Mutations within a model protein are used in cellular studies to understand the connection between protein stability and phase separation. Protein unfolding is necessary but insufficient to drive phase separation. Instead, only those unfolded states that possess a requisite sequence grammar can drive both protein destabilization, to increase the concentration of unfolded states and enable cohesive interactions among unfolded proteins. The formation of unfolded protein deposits (UPODs) allows for specificity in targeting by molecular chaperones such as Hsp40 and Hsp70. These chaperones destabilize UPODs by binding preferentially to and processing unfolded proteins in the dilute phase. Proteomic analysis of UPODs reveal surprises that can be explained based on physico-chemical principles but are confounding from a biological perspective. ![]() Professor Rohit Pappu, Washington University in St. Louis, USA
![]() Professor Rohit Pappu, Washington University in St. Louis, USARohit Pappu is the Gene K. Beare Distinguished Professor of Engineering at Washington University in St. Louis (WashU). He has been at WashU since 2001. Pappu received his PhD in Biological Physics from Tufts University and completed two postdocs, one at WashU in Computational Biophysics, and the second at Johns Hopkins in Biophysics. Pappu’s research interests are focused on the form, function, evolution, and phase behaviour of proteins defined by heterogeneity. These include systems such as intrinsically disordered proteins and multivalent macromolecules. Research in the Pappu lab is driven by adaptations of polymer physics theories, multiscale simulations, in vitro experiments, and collaborations with cell biologists. |
14:15 - 14:40 |
Ionic strength influences interactions between intrinsically disordered proteins and curved membrane surfaces
Intrinsically disordered proteins (IDPs) are a class of proteins that lack substantial regions of tertiary structure and have dynamic behaviour that can be well characterized by polymer theory. In addition, IDPs often contain high degrees of net charge, giving rise to polyampholytic properties as well as electrostatic interactions with anionic membranes. Zeno et al. uses quantitative fluorescence approaches to quantify intramolecular and intermolecular interactions between IDPs and charged membrane surfaces. By modulating the ionic strength of the solution and to control the level of electrostatic screening, they discover that IDPs are potent sensors of membrane curvature. These findings are applicable to a wide range of proteins, especially those involved in membrane trafficking and remodelling. ![]() Dr Wade Zeno, The University of Southern California, USA
![]() Dr Wade Zeno, The University of Southern California, USADr Wade Zeno received his B.S. in Chemical Engineering at the University of Nevada, Reno in 2010, where he also minored in mathematics and chemistry. He then earned a Ph.D. in Chemical Engineering with a Designated Emphasis in Biotechnology from the University of California, Davis in 2016. His doctoral work involved the characterization of biomaterials containing protein-lipid nanodiscs as well as understanding and controlling lipid phase behaviour in the presence of highly concentrated proteins. During this time, he also completed an industrial internship at Bayer Pharmaceuticals where he worked on protein purification and isolation. After completing his Ph.D. work, Dr Zeno did his postdoctoral work in Biomedical Engineering at the University of Texas at Austin, where he studied the role of protein-lipid interactions in membrane traffic. Dr Zeno is now an Assistant Professor of Chemical Engineering and Materials Science at the University of Southern California. |
14:40 - 15:05 |
Phase Transitions and Biomedical Applications
Proteostasis imbalances lead to cell dysfunction and disease. The main source of misfolded proteins in cells are defective ribosomal products (DRiPs), resulting from premature translation termination, damaged mRNAs, DNA mutations. DRiPs are recognized by protein quality control (PQC) machineries, including the chaperones HSP70s, small HSPs and VCP. These chaperones bind to DRiPs and promote their proteasomal degradation. DRiPs can accumulate inside biomolecular condensates called stress granules (SGs) and promote their conversion from a liquid-like state into a solid-like aggregated state. DRiPs also rapidly accumulate in nucleoli and PML bodies, which are biomolecular condensates similar to SGs. By targeting misfolded proteins and DRiPs for degradation, the PQC prevents the conversion of SGs, PML and nucleoli into an irreversibly aggregated state. Thus, targeting aggregation-prone proteins to condensates upon stress is emerging as a general mechanism to prevent irreversible protein aggregation. Our data have important pathological implications, since alteration of SG dynamics and nucleolar stress are implicated in the pathogenesis of Amyotrophic Lateral Sclerosis. Moreover, mutations of chaperones that alter their ability to undergo dynamic phase transitions and chaperone activity are linked to neuromuscular diseases. We propose that boosting chaperone targeting to condensates may maintain phase transition dynamics, offering new therapeutic opportunities. ![]() Dr Serena Carra, University of Modena and Reggio Emilia, Italy
![]() Dr Serena Carra, University of Modena and Reggio Emilia, ItalyDr Carra obtained in Italy a master degree in Pharmaceutical and Chemical Sciences in 1998 and a PhD degree in Neurobiology in 2004. She worked until 2007 as post-doc in Prof. Landry lab (Canada), and from 2007-2009 as Senior Scientist in Prof. Kampinga lab (UMCG, The Netherlands). During this period, Dr Carra discovered the HSPB8-BAG3-HSP70 complex and its role in targeting misfolded proteins to autophagy for clearance. In 2009, Dr Carra was promoted as Assistant Professor at UMCG and pursued her work on the implication of small HSPs in age-related neurodegenerative diseases. In 2011, Dr Carra was awarded the Rita Levi Montalcini Prize and she moved to the University of Modena and Reggio Emilia (Italy), where she is now working as Associate Professor. Dr Carra studies the role of chaperones in the maintenance of proteostasis, as well as their implication in neurodegenerative and neuromuscular diseases. |
15:05 - 15:10 | Break |
15:10 - 15:25 |
Discussion
![]() Dr Justin Benesch, University of Oxford, UK
![]() Dr Justin Benesch, University of Oxford, UKJustin’s research has garnered an international reputation for innovative biophysical chemistry approaches based on combining mass measurement with other experimental methods, simulations, and quantitative thermodynamic and kinetic analyses. This has allowed him and his group to change our thinking as to how proteins assemble, interact, and even evolve. After a degree in Chemistry at the University of Oxford, Justin obtained his PhD from the University of Cambridge for the development and application of novel mass spectrometry approaches working with Professor Dame Carol Robinson FRS. He was awarded fellowships from the Medical Research Council and Royal Society, and appointed to faculty at the University of Oxford in 2012, where he is now Professor in Biophysical Chemistry, and Tutorial Fellow in Physical Chemistry at University College. Justin has been recognised by the Cell Stress Society International with the Alfred Tissières Award, the Howard Prize Lecture from the Biophysical Sciences Institute at Durham, and the Norman Heatley Award from the Royal Society of Chemistry in 2019. His group’s research impacts broadly the interface between chemistry and the life sciences. Their insights have been important to understanding molecular chaperone (mal)function in humans, and the stress tolerance of plants; and their innovations in mass measurement approaches have provided new means for researchers to quantify biomolecules and their interactions. He currently leads two major initiatives in developing a next generation of proteomics approaches. |
Chair
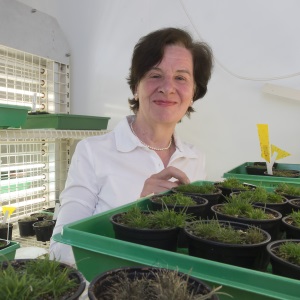
Professor Dorothea Bartels, University of Bonn, Germany
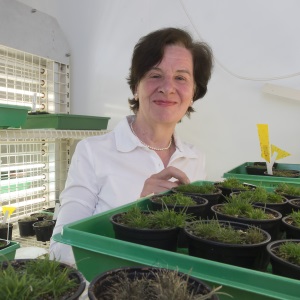
Professor Dorothea Bartels, University of Bonn, Germany
Dorothea Bartels is a professor at the University of Bonn. She studied biology and chemistry at the University of Hannover, Germany. She obtained her doctoral degree in Botany from the University of Hannover, Germany. She worked for several years as a postdoc at the Plant Breeding Institute, Cambridge, UK. Then she became a group leader at the Max Planck Institute for Plant Breeding in Cologne, Germany when she started to work on stress in plants. This was followed by a professorship at the University of Bonn which was succeeded by a professorship at the Vrije Universiteit, Amsterdam, NL and finally she returned to a professorship for physiology and biochemistry of plants at the University of the main research interests are molecular stress physiology in higher plants and particularly anhydrobiosis and how plants adapt to environments with water deficit. She is editor in chief of the international journal Planta, she is an EMBO member and a member of the Academy of Mainz.Bonn.
12:00 - 12:25 |
Evolution of stress resistance in metazoans
Water is essential for life, and a significant reduction of body water usually leads to physiological malfunctioning and eventually death. Nonetheless, some organisms have evolved strategies to survive at least a limited lack of water, and a few taxa have evolved the ability to survive complete dehydration. In particular, microscopic invertebrates like tardigrades, rotifers and nematodes seem to have discovered and adopted a range of strategies to survive stressful conditions which would be lethal for other animals. Despite the importance of understanding the basic principles of how these organisms can cope with stress associated with lack of water and their potential biotechnological applications, the exact mechanisms (behavioural, physiological, cellular and molecular) are still poorly understood, but recent advances in genomics are allowing comparative analyses of both common and unique mechanisms which different taxa employ to survive challenging conditions. This comparative survey of how taxonomically different organisms cope with lack of water highlights similarities and differences and can help informing further studies to understand principles and help developing practical applications. ![]() Dr Chiara Boschetti, School of Biological and Marine Sciences, University of Plymouth, UK
![]() Dr Chiara Boschetti, School of Biological and Marine Sciences, University of Plymouth, UKDr Chiara Boschetti completed her PhD in Animal Biology at the University of Milan (Italy), during which she started studying the “evolutionary scandalous” bdelloid rotifers, small invertebrates which reproduce exclusively asexually and can survive complete desiccation. After a visiting scholarship at Imperial College London (UK) she moved to the University of Cambridge (UK) where she studied the effects of lack of water at the molecular and cellular level in both desiccation-resistant and desiccation-sensitive organisms. Dr Boschetti contributed to the first studies of comparative genomics in bdelloid rotifers and discovered that the high levels of expressed foreign genes (incorporated by horizontal gene transfer) in bdelloids’ genomes can further improve their stress resistance. She then joined the University of Plymouth (UK) as a Lecturer in Biological Sciences, where she is studying rotifers and tardigrades as well as other stress-resistant organisms to further understand how they can survive extreme environmental conditions. |
---|---|
12:25 - 12:50 |
Resurrection plants as models for climate smart agriculture
Drought is the greatest threat to world agriculture and due to global warming, increased aridification is predicted in most current food producing areas. This is particularly significant for Africa, where 95% of agriculture is rain fed. Current crops are intolerant of even moderate water loss and while improved resistance to water loss has been achieved, such mechanisms fail under severe and prolonged drought. There are some 240 Angiosperm species (resurrection plants) that display vegetative desiccation tolerance; a phenomenon which if fully understood, could ultimately be used in the production of crops with increased tolerance to water deficit stress. My group has systematically, using a multidisciplinary approach, investigated mechanisms whereby several different resurrection plants, each as a model for a crop to be transformed, tolerate these extreme conditions. Recent studies include investigations of resurrection plant associated root microbiomes and their potential roles in plant drought tolerance. In this presentation an overview of molecular physiological processes associated with DT in a range of resurrection plants will be given and current and future potential applied outputs discussed. ![]() Professor Jill M Farrant, University of Cape Town, South Africa
![]() Professor Jill M Farrant, University of Cape Town, South AfricaProfessor Jill Farrant holds a South African Research Chair in “Systems Biology Studies on Plant Desiccation Tolerance for Food Security” in the Department of Molecular and Cell Biology, University of Cape Town, South Afric. She utilizes a multidisciplinary approach to understand protection mechanisms associated with vegetative desiccation tolerance, and its regulation, with the ultimate aim of producing drought tolerant crops for food security. She has received considerable recognition for her research, having achieved 10 national and international awards, and her work has been the subject of several International documentaries, which can be found on her website http://www.mcb.uct.ac.za/mcb/people/staff/academic/farrant. Farrant has 151 peer reviewed publications, 16 book chapters, over 200 conference proceedings, has graduated 36 MSc students and 30 PhD students during the 27 years she has been an academic. She is a Fellow of the AAS, RSSAf, TWAS and UCT, and a Member of ASSAf and TWOS. |
12:50 - 13:20 | Poster session |
13:20 - 13:25 | Break |
13:25 - 13:50 |
Sorghum’s Solutions to Drought
Sorghum is a crop adapted for growth in arid climates characterised by water scarcity and extreme heat. Its productivity remains undiminished under harsh drought conditions where other cereals fail. A recent discovery is that the superior stress tolerance of sorghum also exists at the single cell level in primary stem cells prior to differentiation and tissue formation. This provides a useful experimental system for identifying protein/gene networks underpinning sorghum drought stress tolerance. A remarkable finding is that signals secreted by sorghum cells into the extracellular matrix can be used to stimulate Arabidopsis cells to behave as if they were sorghum cells – they become extremely osmotic stress-tolerant to similar levels displayed by sorghum cells. This aligns with the global hypothesis in the Chivasa lab, that extracellular matrix signals are key drivers of collective “decision-making” to synchronise adaptive responses and optimise energy distribution between stress metabolism and productive growth. How nature has solved the drought problem in sorghum is being investigated using this hypothesis and the latest results revealing some of the key players will be presented. ![]() Dr Stephen Chivasa, Durham University , UK
![]() Dr Stephen Chivasa, Durham University , UKOn completing his PhD from the University of Cambridge, Steve Chivasa joined Durham University as a Post-Doctoral Research Associate in the group of Prof Toni Slabas. He left Durham to work in the corporate world and returned to Durham to take up a fellowship in the department of Biosciences. He moved to a tenure-track position in 2018. The fundamental question his research seeks to understand is - how do cells in tissues communicate with their neighbours? The underpinning hypothesis is that cells operate an ingenious collective “decision-making” process where a quorum must be reached for response activation in a decentralized system. The implication of this is that we can target specific tissue compartments for analysis using Proteomics and Metabolomics to unravel key signals driving stress adaptation. This in being applied in research directed at ongoing efforts to develop agritech innovations for protection against damage from drought stress damage. |
13:50 - 14:15 |
Leveraging evolutionary innovations to improve plant resilience
Drought is the most pervasive issue we face in agriculture today and there is considerable interest to produce new crop varieties that can thrive on marginal soils and use less water. Work in the VanBuren Lab focuses on understanding natural adaptations to overcome extreme drought such as the desiccation tolerance found in resurrection plants. To answer fundamental and applied research questions, the lab employs systems-level approaches across diverse model and crop species. Much of this work focuses on wild grasses and orphan cereals in the Chloridoideae subfamily of C4 grasses, which have superior heat, drought, and salinity tolerance compared to other grasses. Comparative genomics, systems biology, physiology, and modelling-based approaches are leveraged to understand the genetic basis of stress tolerance in these unique grasses. The long-term goal of this work is to use natural adaptations to engineer improved stress tolerance and resilience into crop plants. ![]() Dr Robert VanBuren, Michigan State University, USA
![]() Dr Robert VanBuren, Michigan State University, USAThe broad goals of the VanBuren lab are to leverage systems-level approaches to understand how plants respond to complex environmental stresses and how these responses evolved across diverse land plant lineages. The VanBuren lab integrates computational, systems biology, and evolutionary approaches in diverse crop, model, and wild plant systems. Current projects centres on the evolution of desiccation tolerance in resurrection plants and variation in water stress responses in cereals. The lab leverage comparative systems of closely related species with contrasting phenotypes to help dissect the molecular, evolutionary, and network dynamics underlying complex biological processes. The long-term goal of this work is to use these evolutionary innovations to engineer improved stress tolerance and resilience into crop plants. |
14:15 - 14:40 |
Desiccation as an alternative to cold-storage of biologic pharmaceuticals
The past two decades have seen an explosion in the development and use of ‘biologics,’ drugs derived from or containing components of living organisms. This includes protein and nucleic acid-based pharmaceuticals, anti-venoms, allergens, blood, blood components, and cells. While biologics have proven both effective and efficient, they have one major drawback, many are inherently unstable. Currently, the most widespread method of biologic stabilization is cold storage. Cold storage can be effective, but in remote or developing parts of the world, under austere and crisis conditions, or during prolonged space missions, the cold storage represents an enormous economic and logistic burden. To unlock the full potential of biologics, economic and effective means of stabilization must be developed for these life-saving therapeutics. Building on the past contributions of researchers in the desiccation tolerance field, and our own fundamental research on anhydrobiosis, we are developing and testing innovations for storing diverse biologics in a dry, unrefrigerated state. We seek to understand the detrimental effects desiccation imposes on diverse biomolecules, what types of natural protectants are most efficient at preventing these effects, and how we can use genetic, protein-, and chemical-engineering to tune protection for specific biologic targets. ![]() Dr Thomas Boothby, Department of Molecular Biology, University of Wyoming, USA
![]() Dr Thomas Boothby, Department of Molecular Biology, University of Wyoming, USADr Thomas Boothby is an assistant professor of molecular biology at the University of Wyoming. Dr Boothby received his Ph.D. from the University of Maryland where he studied desiccation tolerance in fern spores. He completed his postdoctoral work at the University of North Carolina in the departments of biology and chemistry studying stress tolerance in tardigrades. His research at the University of Wyoming centres around gaining a fundamental understanding of how organisms survive extreme stresses, such as desiccation, and how we can harness this fundamental knowledge to solve ‘real world problems.’ |
14:40 - 14:45 | Break |
14:45 - 15:45 |
Closing Discussion
![]() Professor Dorothea Bartels, University of Bonn, Germany
![]() Professor Dorothea Bartels, University of Bonn, GermanyDorothea Bartels is a professor at the University of Bonn. She studied biology and chemistry at the University of Hannover, Germany. She obtained her doctoral degree in Botany from the University of Hannover, Germany. She worked for several years as a postdoc at the Plant Breeding Institute, Cambridge, UK. Then she became a group leader at the Max Planck Institute for Plant Breeding in Cologne, Germany when she started to work on stress in plants. This was followed by a professorship at the University of Bonn which was succeeded by a professorship at the Vrije Universiteit, Amsterdam, NL and finally she returned to a professorship for physiology and biochemistry of plants at the University of the main research interests are molecular stress physiology in higher plants and particularly anhydrobiosis and how plants adapt to environments with water deficit. She is editor in chief of the international journal Planta, she is an EMBO member and a member of the Academy of Mainz.Bonn. |