Links to external sources may no longer work as intended. The content may not represent the latest thinking in this area or the Society’s current position on the topic.
Interdisciplinary approaches to dynamics in biology
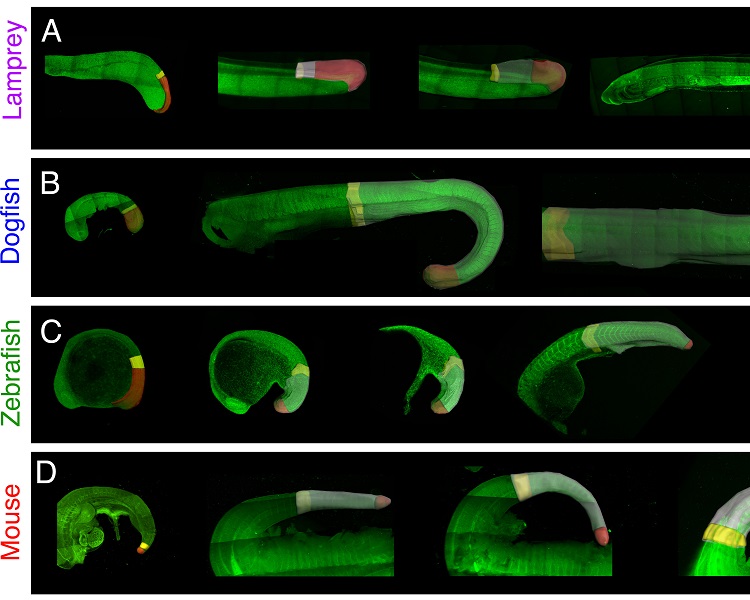
Theo Murphy international scientific meeting organised by Dr Ruben Perez-Carrasco and Dr Berta Verd.
This meeting brought together an interdisciplinary group of scientists interested in the timing of biological processes. Biology is dynamic at every scale, from transcription in a single cell to evolution. Increasingly, technological advances allow us to temporally quantify biological processes. The challenge we now face, which this meeting addressed, is to extract mechanistic understanding from the dynamics we measure.
Information on the speakers and programme is available below. Speaker abstracts are also available below. Recorded audio of the presentations is available below. Meeting papers will be published in a future issue of Interface Focus.
Attending this event
This meeting has taken place.
Enquiries: contact the Scientific Programmes team
Organisers
Schedule
09:20 - 09:55 |
Complex dynamics of ecological systems
Many analyses of ecological systems are still based on the idea that natural (or managed) systems are essentially close to stable equilibria of corresponding simple ecological models. Moving away from this paradigm is key for understanding both natural and managed systems and designing management strategies. The presence of strong density dependent interactions, multiple time scales including changing environmental conditions, spatial interactions, and high dimension all contribute to making the inclusion of the potential for complex dynamics key for understanding ecological systems. Here complex dynamics includes all the dynamical features, including chaotic dynamics, complex basin boundaries, consequences of bifurcations, stochasticity, transient dynamics, as well as other aspects. Recent progress in the kinds of analyses that can be used will be reviewed. The results will be applied to various specific examples from a variety of ecological systems. ![]() Professor Alan Hastings, University of California, Davis and Santa Fe Institute, USA
![]() Professor Alan Hastings, University of California, Davis and Santa Fe Institute, USADr Alan Hastings is a Distinguished Professor in the Department of Environmental Science and Policy at the University of California, Davis. He has published over 300 papers on aspects of theoretical ecology including many focusing socio-ecological systems and especially on the theory behind the design and function of marine protected areas. He is an elected member of the National Academy of Sciences, and a fellow of the American Academy of Arts and Sciences and the American Association for the Advancement of Science. He is also a fellow of the Ecological Society of America, the Society for Industrial and Applied Mathematics and the Society for Mathematical Biology. He is also the founding Editor-in-Chief of the journal Theoretical Ecology and co-edited the Encyclopedia of Theoretical Ecology. |
|
---|---|---|
09:55 - 10:30 |
Dynamical models and comparative biology: homology and analogy of processes
This talk explores the role of dynamical models of developmental processes in comparative biology. Dynamical models of processes such as insect segmentation and vertebrate somitogenesis can explain how these processes might plausibly work, or how they actually work, depending on their level of mechanistic detail. As a consequence, these models can inform phylogenetic hypotheses about homology, parallelism, and convergence by determining the probabilities of transformation between character states. An interesting question concerns how dynamical processes themselves should be described and classified in order to function this way. Should they be classified (1) independently of phylogenetic considerations eg, in terms of ahistorical properties such as phase portrait geometries or, (2) in terms of phylogeny? The first option may be useful at certain stages of research, especially when combined with mechanistic research approaches. But ignoring phylogeny threatens to resurrect some of the classic problems with the pre-Darwinian programs of “rational taxonomy” or “idealistic morphology.” Pursuing the second option gives occasion to re-examine the unresolved conceptual issue of “process homology” from the point of view of dynamical modeling, and to assess its relation to the classical criteria of homology, such as topology, complexity, and congruence. The central question concerns how to productively combine formal and historical approaches to understanding developmental processes. ![]() Dr James DiFrisco, Centre for Logic and Philosophy of Science, Leuven, Belgium
![]() Dr James DiFrisco, Centre for Logic and Philosophy of Science, Leuven, BelgiumJames DiFrisco is a philosopher of biology interested in the role of dynamical models in developmental biology, homology and character identity, the evolution of biological individuality, and the origin and nature of levels of organisation. He obtained a PhD in philosophy from Leuven, Belgium in 2015, and was a postdoctoral fellow at the Konrad Lorenz Institute for Evolution and Cognition Research, Austria from 2015 – 2018. Currently he is working on a research project at Leuven entitled “Hierarchical Evolution of Dynamical Systems”. During the spring 2020 semester he is a laboratory associate in the Department of Ecology & Evolutionary Biology at Yale University. |
|
10:30 - 11:00 | Coffee | |
11:00 - 11:35 |
Dynamical process in biology: on the role of mathematical modelling
Biology is fundamentally dynamic, and mathematical modelling plays an increasingly prominent role in understanding dynamical behaviours of living systems. What does a mathematical representation bring to the study of such systems? A quantitative description can enhance the clarity of assumptions made about the constitution of the system, and open up possibilities for more precise prediction. However, can the use of mathematical models do more than this? In addition to providing a precise deductive framework, mathematics also provides a language for abstract reasoning, with logical structures that do not necessarily correspond in any simple way to the physical elements of a system. Given the complexity of biological phenomena, tracing the route from system composition to system behaviour is challenging: the language of dynamical models provides a natural framework for exploring this dynamic process. ![]() Professor Nick Monk, University of Sheffield, UK
![]() Professor Nick Monk, University of Sheffield, UKNick Monk is Head of the School of Mathematics and Statistics at the University of Sheffield. Following an undergraduate degree in Mathematics and a PhD in Theoretical Physics, his research interests have been on the development and analysis of mathematical models of biological systems. His research has focused mainly on the mechanisms by which cells use signalling to coordinate their activities, particularly in the context of embryonic development. Working closely with experimental biologists, he has studied spatio-temporal patterning in a range of animal and plant systems. Complementary to his work in mathematical biology, he has an interest in exploring how a philosophical approach in which process and flow are primary can be used as a framework for understanding living systems. |
|
11:35 - 12:10 |
Dissecting transcriptional dynamics in development one burst at a time
Over the last few decades in vitro and in situ approaches have revealed the identity of the molecular players driving transcription in eukaryotes. Yet, these studies are virtually silent on the precise timing of the recruitment of each of these players to the promoter, and on how this recruitment determines output transcriptional dynamics in vivo. Here, we present a new method for simultaneously measuring local input transcription factor concentration at target loci and the resulting output transcriptional activity of these loci in single living cells. Specifically, we study how the Dorsal activator, a key transcription factor in the development of the fruit fly Drosophila melanogaster, is recruited to the promoter of its target gene snail in order to drive transcriptional bursting. We found that transient surges in Dorsal concentration coincide with, but do not precede, the onset of transcriptional bursts. Interestingly, these surges are not maintained throughout the duration of the bursts and subside before the promoter transitioned back into a transcriptionally inactive state. Instead, we discovered that the amplitude of the transient Dorsal concentration surges at the start of transcriptional burst, and not surge duration, dictates transcriptional burst duration. We speculate that Dorsal delivers a “package” of downstream players to the promoter (eg, a cluster of RNA polymerase molecules) that sustains the transcriptional burst until this package is exhausted. Thus, our tool sets the stage for uncovering the precise timing and ordering of the diverse molecular players that drive the transcriptional process. ![]() Dr Hernan Garcia, UC Berkeley, USA
![]() Dr Hernan Garcia, UC Berkeley, USAHernan G Garcia is an assistant professor in the Departments of Molecular & Cell Biology and of Physics at UC Berkeley. As a Physical Biologist, his research aims to uncover the quantitative and predictive principles dictating biological phenomena, with particular emphasis on embryonic development. To make this discourse between theory and experiment possible, Hernan has pioneered several technologies to measure and control transcription and translation in single cells of living fruit fly embryos. Hernan is a co-author of the textbook Physical Biology of the Cell and a co-director of the equally named course at the Marine Biological Laboratory in Woods Hole, MA. |
13:10 - 13:45 |
Dynamics of neural patterning in Drosophila
The genetic and molecular substrates of many developmental processes have been identified, yet how spatial patterns dynamically emerge, and the relative contribution of positional cues and self-organization, often remain unclear. Neural patterning in Drosophila is commonly described as a two-step process, where prepattern factors drive localized proneural gene expression, then Notch-mediated lateral inhibition singles out neural precursors. In the dorsal thorax of the adult fly, small sensory bristles are arranged in five rows. A combination of genome engineering, imaging and mathematical modelling revealed that this ordered layout is organized by Notch, which establishes a series of proneural stripes that resolve into regular rows of sensory organ precursor cells. Patterning is initiated by a gradient of Delta ligand, and progresses through inhibitory signalling between and within the stripes. A simple mathematical model captures this temporal sequence, and suggests that the interplay between cell-intrinsic dynamics and signalling is essential for the gradual refinement of the pattern. It is anticipated that a similar logic could be at play in other contexts, with different initial or boundary conditions producing different arrangements of neural precursors. This possibility is explored in ongoing work on patterning in the fly eye, where a regular lattice of R8 photoreceptor cells emerges in the wake of a travelling differentiation front. ![]() Dr Francis Corson, CNRS Ecole Normale Supérieure, France
![]() Dr Francis Corson, CNRS Ecole Normale Supérieure, FranceFrancis Corson is a theoretical biophysicist and CNRS research scientist at Ecole Normale Supérieure in Paris. Following a PhD in physics at ENS, devoted to plant morphogenesis, and a postdoc in the group of Eric Siggia at the Rockefeller University, where he developed new modelling approaches to fate specification, he has held CNRS positions at the Pasteur Institute then at ENS. Drawing on his background in nonlinear physics, and on close collaborations with experimentalists, his research aims to develop simple models to illuminate the dynamics of developmental patterning and morphogenesis, with a particular interest for self-organization, explored in such systems as the Drosophila peripheral nervous system and the early amniote embryo. |
|
---|---|---|
13:45 - 14:20 |
The dynamics of spinal cord development
The generation of the correct cell types at the appropriate position and time is the first step in the assembly of functional tissues. One well-studied example is the development of the vertebrate spinal cord. In this tissue, distinct neuronal subtypes are generated in a precise spatial order from progenitor cells arrayed along the dorsal-ventral axis of the neural tube. Underpinning this organization is a complex network of extrinsic and intrinsic factors. Particularly well understood is the mechanism that determines the generation of different neuronal subtypes in ventral regions of the spinal cord. In this region of the nervous system, the secreted protein Sonic Hedgehog (Shh) acts in graded fashion to organize the pattern of neurogenesis. This is a dynamic process in which exposure to Shh generates progenitors with successively more ventral identities. At the same time tissue growth alters the arrangement of cells and the proportions of cell types and contributes to the elaboration of pattern. A gene regulatory network composed of transcription factors regulated by Shh signalling play an essential role in determining the response of cells. The dynamics of this network controls the pattern, pace, precision and proportion of the forming neural tube. Thus, accurate development of the neural tube and the specification of neuronal subtype identity relies on the interplay of cellular and molecular processes. ![]() Dr James Briscoe FMedSci FRS, The Francis Crick Institute, UK
![]() Dr James Briscoe FMedSci FRS, The Francis Crick Institute, UKJames Briscoe is a senior group leader at the Francis Crick Institute. He obtained a BSc in Microbiology and Virology from the University of Warwick, UK. Following his PhD research in Ian Kerr's laboratory at the Imperial Cancer Research Fund, London, he undertook postdoctoral training at Columbia University, New York, USA, with Thomas Jessell, first as a Human Frontiers Science Program Fellow then as a Howard Hughes Medical Institute Fellow. In 2000 he moved to the Medical Research Council's National Institute for Medical Research to establish his own research group and in 2001 he was elected an EMBO Young Investigator. He was awarded the EMBO Gold Medal in 2008 and elected to EMBO in 2009. In 2018 he became Editor in Chief of Development, a journal published by the Company of Biologists, a not-for-profit scientific publisher. His current research interests include the molecular and cellular mechanisms of graded signalling by morphogens and the role of transcriptional networks in the specification of cell fate. To address these questions his lab uses a range of experimental and computational techniques with model systems that include mouse and chick embryos and embryonic stem cells.
|
|
14:20 - 14:55 |
Oscillations and noise: partners in neural cell state transitions in vivo
In recent years, our understanding of how cells make cell state transitions has been transformed by discovery of short-time scale dynamics in gene expression, which can be oscillatory or noisy. Ultradian oscillations are exemplified by the expression of HES/Her transcription factors in neural progenitors but it is not known whether oscillations occur in vivo what is the role of noise. This talk will describe a quantitative and dynamic single cell level imaging approach to analyse ultradian oscillations and noise during cell state transitions during mouse and zebrafish neural development. Using reporter protein fusion knock-ins, complex HES5 dynamics are revealed in mouse neural tissue, with both short and long term trends. Contrary to expectations, HES5 expression becomes more frequently periodic as cells transition to differentiation. Coupled with an overall decline in HES5 expression this creates a transient period of oscillations with higher fold expression change, which presumably increases the decoding capacity of HES5 oscillations. Mathematical modelling suggests that noise has an oscillatory “priming” function because the HES5 oscillator operates close to its bifurcation boundary where stochastic conversions between dynamics are enabled. CRISPR/Cas9 mutagenesis of a miR-9 binding site in a knock-in Her6 reporter in zebrafish shows that changes in Her6 dynamics, including an increased high frequency noise has the opposite effect of locking cells in a progenitor state which is normally transitory. These findings show how oscillations operate during cell state transitions at the tissue level and reveal that noise optimization by miR-9 is necessary for transitions to occur. ![]() Professor Nancy Papalopulu, University of Manchester, UK
![]() Professor Nancy Papalopulu, University of Manchester, UKNancy Papalopulu did her PhD at the National Institute for Medical Research, London with Dr Robb Krumlauf and a post-doc at the Salk Institute for Biomedical Research, US with Dr Chris Kintner. She was a Group Leader at the Gurdon Institute, Cambridge, UK and moved to the University of Manchester to take up a Professorship in Developmental Neuroscience in 2006. She is a Wellcome Trust Senior Research Fellow, an elected member of EMBO and a Fellow of the Academy of Medical Sciences. Nancy’s work combines experimentation, in particular quantitative and dynamic approaches, with theoretical modeling to understand how oscillatory transcription factor expression dynamics control fundamental cell state transitions during the development of the vertebrate central nervous system. |
|
14:55 - 15:25 | Tea | |
15:25 - 16:00 |
Unveiling the dynamics of commitment and fate choice in the developing nervous system
An ambitious goal of single-cell analyses is to describe dynamical biological processes and shed light on gene regulation. However, the destructive nature of transcriptomics measurements constitutes an immediate challenge to this purpose. “RNA velocity” is an analysis framework that helps to overcome this fundamental limit. It uses a single-cell transcriptomic snapshot measurement to estimate the time derivative of gene expression for a single cell. We applied the analysis framework to study different processes occurring during development, including differentiation, commitment, and cell cycle dynamics. In this talk, we will showcase the insights that the method is giving in the developing nervous system and the retina. In particular, our analysis captures details of differentiation dynamics in the Dentate Gyrus, cell cycle dynamics in retinal progenitors, and commitment biases. We discuss how to ensure that the estimation is not confounded by technical factors and on how to apply the framework to larger and more complex datasets. ![]() Dr Gioele La Manno, Swiss Federal Institute of Technology Lausanne (EPFL), Switzerland
![]() Dr Gioele La Manno, Swiss Federal Institute of Technology Lausanne (EPFL), SwitzerlandGioele La Manno was appointed in November 2018 as the first ELISIR Fellow at the School of Life Sciences of EPFL, giving him the privilege to start his own independent research group directly after defending my PhD. Dr La Manno earned his PhD at Karolinska Instituet and he is proud alumnus of the Linnarsson Lab. Dr La Manno’s original training is in Biotechnology (BSc @UNIPA) and Biomedicine (MSc @KI) but nowadays he will probably turn around if somebody is calling out for a Molecular Biologist, Computational Biologist or Bioinformatician. Dr La Manno’s research focuses on understanding brain development. He is particularly interested in describing the sequence of states that embryonic stem cells go through during their differentiation towards mature neurons. He has been tackling this question using machine-learning tools on the wealth of data provided by single cell RNA sequencing, a technique he has been working with and developing since its early times. |
09:00 - 09:35 |
Dynamic gene regulation at the single-cell level
Gene expression can be surprisingly dynamic and heterogeneous. This variability in gene expression, even in a clonal population of cells grown under the same condition, has been observed in diverse organisms, from mammalian stem cells to bacteria. It remains unclear how this variability is generated and what function it can serve. By using a combination of single cell time-lapse microscopy, mathematical modelling and synthetic biology techniques, the Locke group are attempting to understand how gene circuits generate dynamic gene expression, and how this dynamic information is transferred to downstream processes and other pathways. They work on simpler model systems such as cyanobacteria and B. subtilis, where it is possible to have precise single cell control of gene regulation, but have also extended this approach to the model plant Arabidopsis. In Arabidopsis, they are building on their work on simpler systems to examine the functional role of dynamic, and even stochastic, gene regulation in development. ![]() Dr James Locke, Sainsbury Laboratory, University of Cambridge, UK
![]() Dr James Locke, Sainsbury Laboratory, University of Cambridge, UKJames Locke is the Associate Director of the Sainsbury Laboratory, University of Cambridge. James graduated from the University of Warwick (2000) in Physics, before completing Maths Part III at Cambridge (2001). James then studied for a PhD at the University of Warwick (2006). He used an iterative process of experiment and theory to propose a new feedback loop in the plant circadian (24-hour) clock. He then conducted his postdoctoral work in the lab of Professor Michael Elowitz at the California Institute of Technology. He applied single cell time-lapse microscopy, modelling, and synthetic biology techniques to understand how cells amplify small molecule differences (noise) into alternative transcriptional states. Since 2012 he has been a group leader at the Sainsbury laboratory investigating gene expression dynamics in microbial and plant systems. He was awarded the Merrimack-CSB2 Prize in Systems Biology in 2013 and a Royal Society University Fellowship in 2015. |
|
---|---|---|
09:35 - 10:10 |
Our first choices: decoding signals during developmental transitions (lessons from quantitative biology)
During early development, extrinsic triggers prompt a collection of pluripotent cells in the blastocyst to begin the dramatic and long process of differentiation that gives rise to the tissues of the three germ layers (endoderm, mesoderm and ectoderm). Precise temporal control during these early fate-choices is paramount and impacts on the success of differentiation. Changes in morphology, gene expression signatures and epigenetic patterns and cell division cycles are believed to mark the point of no-return in fate choices. However, when and how cells irreversibly commit to differentiation is a fundamental, yet unanswered question. Poised to differentiate, embryonic stem (ES) cells are an invaluable model to address this question. Given appropriate differentiation cues, ES cells can recapitulate in vitro all the hallmark events that occur during differentiation and are our system of choice to understand fate decisions at the single cell level. During Silvia’s talk she will share two stories that illustrate how Silvia’s lab combines single cell imaging, genomic approaches and mathematical modeling to study how cells encode fate choices during early development. ![]() Dr Silvia Santos, The Francis Crick Institute, UK
![]() Dr Silvia Santos, The Francis Crick Institute, UKOriginally Portuguese, Silvia Santos left sunny Portugal to study Molecular and Cell Biology in the UK. Silvia did her PhD at the EMBL in Heidelberg where she worked as a Marie Curie E-Star fellow. She moved to Stanford University for her post-doctoral training with Jim Ferrell and Tobias Meyer where she worked initially as an EMBO fellow, and, later as HFSP fellow. Silvia started her independent research at the MRC-LMS in Imperial College London in 2014 as an MRC career development awardee (MRC-CDA). She is joining the Francis Crick Institute in December 2017 as a group leader to establish the Quantitative Cell Biology lab. Since her PhD Silvia combines experimental and theoretical approaches to understand decision-making during transitions. In this context, her lab studies cell division and cellular differentiation in early development, using human embryonic stem (hES) cells as a model system.
|
|
10:10 - 10:40 | Coffee | |
10:40 - 11:15 |
Comparative analysis of multi-cellular dynamics and pattern emergence in the vertebrate tailbud
A major question in developmental biology is how cell fate decisions are coordinated precisely in space and time to generate emergent patterns of gene expression. The timing of cell transitions is regulated at multiple length scales in a highly integrated manner. Within individual cells, gene regulatory networks (GRNs) drive cell state transitions with an inherent timing. These are in turn patterned across cell populations by extracellular signals that diffuse or are transported between cells. This talk focuses on the multi-tissue level and considers how multicellular ensembles shift relative to each other to influence the timing at which competent cells receive signals. Such ‘tissue tectonics’ provide a causal relationship between processes at higher levels of biological organization (ie the relative positioning of signalling and responding tissues) to the control of processes at lower levels (ie cell biology and the activity of GRNs). As such, it is an example of downward causation and provides a multi-scale feedback mechanism to enable the self-organisation of developmental processes. The vertebrate tailbud offers a unique system to explore the relationship between multi-tissue morphogenesis and cell fate coordination in vivo. This will be discussed together with recent work showing how the inhibition of convergence and extension within multi-cellular aggregates of embryonic cells disrupt the shaping of BMP and Wnt/beta-catenin signalling gradients. This leads to alterations in the anterior-posterior patterning of neural marker expression, demonstrating a role for tissue tectonics in pattern formation. ![]() Dr Ben Steventon, University of Cambridge, UK
![]() Dr Ben Steventon, University of Cambridge, UKBen began his studies in Developmental Biology as a PhD student with Roberto Mayor at UCL in 2004. After graduating in 2008, he moved to KCL to work with Andrea Streit. Subsequently he moved to the lab of Jean-Francois Nicolas and Estelle Hirsinger at the Institut Pasteur, Paris. There, he began to work with zebrafish embryos to further the understanding of the tissue deformations that lead to the elongation of the embryonic body axis. To develop imaging and analytical techniques to study this process at the cellular and molecular levels, he was awarded a Marie-Curie fellowship to work with Scott Fraser (University of California, USA) and Alfonso Martinez-Arias (University of Cambridge). With his Sir Henry Dale fellowship, he is now applying these techniques to investigate how cell fate decisions are orchestrated in space and time during axis patterning in zebrafish embryos. |
|
11:15 - 11:50 |
On clocks and timers in development
Some biological oscillators function throughout the life of an organism, for example the circadian clock, whereas others have a more restricted duration, particularly in embryogenesis. The “segmentation clock” is a multi-cellular patterning system of genetic oscillators thought to control the rhythmic and sequential formation of the vertebrate embryo's body segments. Individual oscillating cells are synchronized with their neighbours, forming a coherent wave pattern of gene expression. How these wave patterns arise and how they are regulated during embryogenesis is not clear. Dr Oates will describe recent progress in understanding the behaviour of individual cells from the zebrafish as they slow their oscillations and differentiate during segmentation, and discuss how this gives rise to the tissue-level wave patterns. Central to this understanding is the concept of a timer that regulates the duration of a clock. This perspective reveals what part of the oscillatory cycle is changing as the cells slow and stop. ![]() Dr Andy Oates, Swiss Federal Institute of Technology Lausanne, Switzerland
![]() Dr Andy Oates, Swiss Federal Institute of Technology Lausanne, SwitzerlandAndrew Oates received his PhD at the Ludwig Institute for Cancer Research and the University of Melbourne. His postdoctoral time was at Princeton University and the University of Chicago in the lab of Robert Ho, where his studies on the segmentation clock in zebrafish began in 1998. In 2003 he moved to Germany and started his group at the Max Planck Institute for Molecular Cell Biology and Genetics in Dresden. In 2012 he accepted a position at University College London as Professor of vertebrate developmental genetics and moved his group to the MRC-National Institute for Medical Research at Mill Hill in London. From April 2015, he became a member of the Francis Crick Institute in London. In September 2016, he joined École Polytechnique Fédéral de Lausanne (EPFL) in Switzerland as a Professor, where he is the head of the Timing, Oscillation, Patterns Laboratory and the Director of the Institute of Bioengineering. The Oates group is composed of biologists, engineers, and physicists using molecular genetics, quantitative imaging, and theoretical analysis to study a population of coupled genetic oscillators in the vertebrate embryo termed the segmentation clock. This system drives the rhythmic, sequential, and precise formation of embryonic body segments, exhibiting rich spatial and temporal phenomena spanning from molecular to tissue scales. |
|
11:50 - 12:25 |
Human time vs mouse time with stem cell differentiation
Different species have different tempos of embryonic development: larger animals tend to grow more slowly than smaller animals. Dr Ebisuya's group has been trying to understand the molecular basis of interspecies differences in developmental time by using in vitro segmentation clock as a model system. The segmentation clock is the oscillatory gene expressions that regulate the timing of body segment formation from presomitic mesoderm (PSM) during embryogenesis. The group has recently succeeded in inducing PSM from both human iPS cells and mouse ES cells, recapitulating the oscillation and travelling wave of segmentation clock in vitro. Interestingly, the oscillation period of human segmentation clock was 5-6 hours while that of mouse was 2-3 hours. Taking advantage of our in vitro system, the group measured several biochemical reaction parameters of the core gene of the oscillation mechanism, Hes7, finding out that the degradation and production processes of Hes7 are 2-3 times slower in human PSM cells compared to mouse cells. The mathematical model quantitatively explained how the slower biochemical reactions in human cells give rise to the longer oscillation period in the human segmentation clock. ![]() Dr Miki Ebisuya, EMBL Barcelona, Spain
![]() Dr Miki Ebisuya, EMBL Barcelona, SpainMiki Ebisuya did her undergraduate and PhD research at Kyoto University, Japan. After getting her PhD in 2008, she became a group leader at Kyoto University in 2009. Her lab moved to RIKEN in 2013, and then moved again to EMBL Barcelona, Spain, in 2018. The research interest of her group is reconstituting developmental mechanisms in cell culture. |
13:25 - 14:00 |
Dynamics of segment patterning in arthropods
Segmentation in arthropods is established during development, often concurrently with posterior elongation. Much about this patterning process is still poorly characterised, but recent years have seen considerable progress towards grasping its basic regulatory structure and logic. Many of the genes that affect segmentation appear to be linked into small networks that form dynamical components such as timers, oscillators, and switches. Additional cross-regulation between these components creates a dynamical interplay that is fundamental to producing the segmental pattern. Several of these dynamical components appear to be strikingly similar to ones involved in patterning the nervous system, suggesting perhaps that they evolved within a more ancient developmental context and were only later recruited to a role in body segmentation. Alternatively, body segmentation might have originated from an ancestral neuromuscular patterning process which involved these components from the start. Simple models that capture the essential dynamical properties of the segmentation network show how linking developmental processes that create traveling waves (velocities) with others that measure time is sufficient to generate segmental patterns, even in the absence of instructive initial conditions. In addition, through adjusting the relative timescales of these components, a given system can produce a range of different output patterns, demonstrating how different body forms might easily evolve. On the other hand, the example of Drosophila shows that instructive positional gradients can also be incorporated into such a system, replacing a robust but relatively slow self-organising capacity with finely-tuned top-down control. Even in this extreme case, however, it is clear that dynamical processes still form a fundamental, ancestral, core. ![]() Dr Erik Clark, Harvard Medical School, USA
![]() Dr Erik Clark, Harvard Medical School, USAErik Clark is a developmental systems biologist interested in patterning, gene regulation, and evolution. He is currently working in the DePace lab in the Department of Systems Biology at Harvard Medical School, on an EMBO postdoctoral fellowship. He is also a Junior Research Fellow at Trinity College, Cambridge. He did his PhD with Michael Akam at the University of Cambridge, graduating in 2017. He also has a BA in Biological Sciences from the University of Oxford and an MSc in Bioinformatics and Theoretical Systems Biology from Imperial College London. He studies anterior-posterior patterning processes in arthropod embryos, focusing in particular on the regulation and function of the “pair-rule” genes in the fruit fly Drosophila melanogaster. |
|
---|---|---|
14:00 - 14:35 |
Waves, flows and droplets: physical organisation of embryogenesis and regeneration
Embryonic development and tissue regeneration both require a high degree of spatiotemporal coordination. Dr Di Talia will describe my lab efforts to understand the synchronization of the cell cycle in early Drosophila embryos and the coordination of tissue growth in zebrafish bone regeneration. Dr Di Talia will show how synchronization of the cell cycle in Drosophila embryo is linked to precise nuclear positioning, which is in turn driven by cytoplasmic flows generated by actomyosin cortical contractions. Cortical contractions are regulated by oscillations in the activity of mitotic phosphatase PP1, thus forming a self-organized mechanism through which nuclei drive their positioning and synchronization. Dr Di Talia will also show how the cell cycle oscillator is interfaced with zygotic histone biogenesis by mediating the formation of the histone locus body by phase separation. Finally, Dr Di Talia will show that excitable waves of growth factor signalling drive cell and tissue growth in the osteoblast population controlling zebrafish scale regeneration, thus highlighting a novel principle of large-scale coordination for regeneration of large adult structures. ![]() Dr Stefano Di Talia, Duke University Medical Center, USA
![]() Dr Stefano Di Talia, Duke University Medical Center, USAStefano received his PhD from The Rockefeller University where he worked on the mechanisms of cell size control in budding yeast. As a postdoc in Princeton University, he initiated a new research line on the mechanisms of cell cycle regulation during Drosophila embryonic development. He established his lab in the Department of Cell Biology at Duke University, where he works on physical principles of spatiotemporal organisation in early Drosophila embryos. Recently, the lab has also initiated a new research direction to understand how tissue growth is controlled during zebrafish regeneration. |
|
14:35 - 15:10 |
Mapping space and time in developing model systems
During development, sequential cell fate decisions generate diversity by carefully orchestrating their organisation across space and time. Often the dynamics of cell fate decisions directly relate to spatial location, such as in the case of somitogenesis where decisions are organised along the embryonic anterior-posterior axis. Advances in single-cell transcriptomics have enabled the characterisation of numerous cell states to be identified with high-resolution, yet these methods typically require cellular dissociation which disrupts such spatial information. In silico techniques, such as pseudotime approaches, often aim to ‘reintroduce’ dynamics by using information inherent in the data but make several assumptions. Instead, the group sought to identify spatial information inherent in such single-cell datasets using a parallel spatially-defined bulk RNA-sequencing and complementary single-cell data. The group developed a pipeline to recover spatial information at single-cell resolution, which allows them to reconstruct cellular arrangements that closely reflect the original organisation of source tissues. This method has potential implications for transcriptomic assays, where preserving spatial information in high-resolution data could allow the identification of novel spatial and dynamic trends along embryonic axes. ![]() Dr Naomi Moris, University of Cambridge, UK
![]() Dr Naomi Moris, University of Cambridge, UKNaomi Moris is a Junior Research Fellow in the Department of Genetics at the University of Cambridge. She is particularly interested in understanding how cell fate decisions are coordinated during development. To explore this question, she makes use of three-dimensional in vitro model systems, such as ‘gastruloids’, to explore self-organisation principles using embryonic stem cells. In particular, she is interested in understanding how developmental programmes are organised in both space and time, to generate coordinated patterns as a result of underlying gene regulatory networks. |
|
15:10 - 15:40 | Tea | |
15:40 - 16:15 |
Using avian models to understand developmental tempo
Embryonic development is a process of orchestrated progressive steps, which lead to the generation of patterned structures. While the sequence of developmental events is highly conserved, the speed at which they occur or “tempo” (“embryonic tempo” as a term was defined by Ebisuya and Briscoe, 2018) is a unique feature of a species and is highly variable within chordata and even between closely related species. Embryonic tempo is a mostly unexplored field presenting an opportunity to investigate and develop a fundamental area of biology with far reaching implications for many fields, including stem cell research, developmental biology, life-long health, ecology and food production. Variability in tempo, suggests that changes can be effected through few pleiotropic changes and there is evidence that the control of tempo is genetic. Dr Davey will describe the preliminary steps we are taking to define embryonic tempo and understand the genetic control of tempo using commercial duck species and chicken breeds and other avian species which demonstrate highly regulated embryonic tempo. ![]() Dr Megan Davey, University of Edinburgh, UK
![]() Dr Megan Davey, University of Edinburgh, UKMegan Davey undertook her BSc Hons in Anatomy and Developmental Biology at UCL 1994 – 1997 and her PhD at The University of Dundee 2000 – 2003, specialising in understanding the development and patterning of the limb, using chicken developmental mutants. Starting as a postdoc at The Roslin Institute in 2004 and becoming a BBSRC Fellow there in 2007, Megan has continued to explore the function of causative mutations which lead to variations in phenotype using comparative anatomy, genomics and embryonic manipulation of avian species, including chickens, quails, ducks and emus. As well as practical applications for human developmental differences, Megan’s group are interested in understanding how differences in developmental tempo are controlled genetically and how these contribute to pattern formation in the embryo. Megan is also Academic Lead for Public Engagement at the Roslin Institute and Secretary of the British Society for Developmental Biology. |
|
16:00 - 17:00 | Closing remarks |