Links to external sources may no longer work as intended. The content may not represent the latest thinking in this area or the Society’s current position on the topic.
Software solutions to the challenges of materials modelling
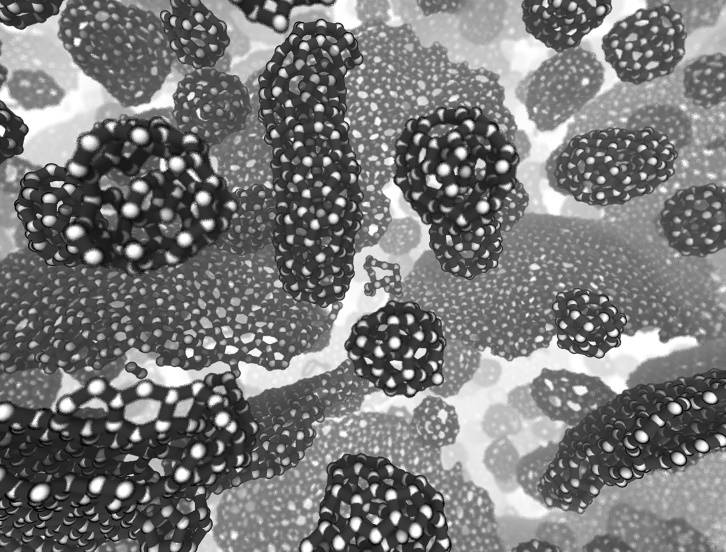
Satellite meeting organised by Professor Scott Woodley, Professor Sir Richard Catlow FRS, Professor Nora H de Leeuw and Professor Angelos Michaelides FRS.
In the Discussion meeting the aim was to discuss the challenges and achievements in materials modelling that will and have been enabled by High End Computer (HEC) resources. The Satellite meeting moved on to the software solutions recently developed and still required in order to effectively exploit HEC to address the scientific challenges identified during the Discussion meeting.
The schedule of talks and speaker biographies can be found below. Speaker abstracts can also be found below.
Attending this event
This meeting has taken place.
Enquiries: contact the Scientific Programmes team.
Organisers
Schedule
Chair
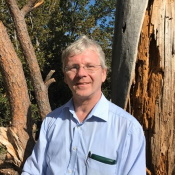
Professor J Christian Schoen, Max-Planck-Institute for Solid State Research, Germany
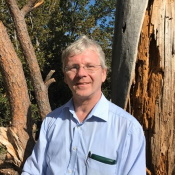
Professor J Christian Schoen, Max-Planck-Institute for Solid State Research, Germany
J Christian Schön studied physics at Bonn University and at MIT, where he received his PhD in theoretical solid state physics in 1988. Subsequently, he was Postdoc in mathematics at SDSU in San Diego, in physics at the Universities of Odense and Copenhagen, and in chemistry at Bonn University, where he became apl Professor in 2008. He is a Fellow of the Royal Society of Chemistry. Since 1999, he has been a Senior Scientist at the Max Planck Institute for Solid State Research. His main research areas are the theory of complex multi-minima-energy landscapes and their application in chemistry, such as prediction and modeling of molecular structures and clusters in vacuum and on surfaces, of metastable crystalline and amorphous materials in 1 - 3 dimensions, and the simulation and optimisation of their synthesis, employing techniques from the area of global optimisation, MC and MD simulations, optimal control and finite-time thermodynamics.
07:55 - 08:00 | Welcome |
---|---|
08:00 - 08:30 |
The NOMAD Artificial-Intelligence toolkit: turning materials-science data into knowledge and understanding
The talk describes recent glass-box (in contrast to black box) AI developments for learning rules for materials properties and functions by artificial intelligence and for identifying materials genes.1,2 Then Professor Scheffler will present the Novel-Materials-Discovery (NOMAD) Artificial-Intelligence (AI) Toolkit3, a web-based infrastructure for the interactive AI-based analysis of the material-science. The present implementation is linked to the NOMAD Archive, the largest database of computational materials-science data worldwide4, 5. However, the NOMAD Oasis, a local, standalone server can be used as well. Users of the NOMAD AI toolkit can modify the offered Jupyter notebooks towards their own research work. 1C Draxl and M Scheffler, Big-Data-Driven Materials Science and its FAIR Data Infrastructure. Plenary Chapter in Handbook of Materials Modeling (eds. S. Yip and W. Andreoni), Springer (2020). Reprint: doi.org/10.1007/978-3-319-44677-6_104 2M Boley and M Scheffler, Learning Rules for Materials Properties and Functions. Roadmap for Machine Learning in Electronic Structure Theory, ed. by S. Botti and M. Marques; Preprint: https://arxiv.org/abs/2104.01352 3The NOMAD AI Toolkit: https://nomad-lab.eu/aitoolkit 4C Draxl and M Scheffler, The NOMAD Laboratory: From Data Sharing to Artificial Intelligence. J. Phys. Mater. 2, 036001 (2019). Reprint DOI: 10.1088/2515-7639/ab13bb 5The NOMAD Data Base: https://nomad-lab.eu/prod/v1/staging/gui/about/information ![]() Professor Matthias Scheffler, The NOMAD Laboratory at the FHI of the Max Planck Society, Germany
![]() Professor Matthias Scheffler, The NOMAD Laboratory at the FHI of the Max Planck Society, GermanyMatthias Scheffler is the Director of the NOMAD (Novel Materials Discovery) Laboratory at the FHI of the Max Planck Society in Berlin (formerly, until the end of 2020, this was called the 'FHI Theory Department'). He works in condensed-matter theory, materials science, the chemical physics of solids, and artificial intelligence. A particular focus is on density-functional theory and many-electron quantum mechanics and on developments of multi-scale approaches. The latter is summarised by the appeal 'Get Real!', introducing environmental factors (eg partial pressures, deposition rates, and temperature) into ab initio calculations. In recent years, his work is increasingly concerned with data-centric scientific concepts and methods (the 4th paradigm of materials science) and the goal that materials-science data must become 'Findable and Artificial Intelligence Ready'. |
08:30 - 09:00 |
Protocols for fitting first principles force fields using machine learning
Abstract not available at time of publication. ![]() Professor Gábor Csányi, University of Cambridge, UK
![]() Professor Gábor Csányi, University of Cambridge, UKGábor Csányi obtained a degree from Cambridge in mathematics and a PhD in physics from MIT. After postdoctoral work at the Cavendish Laboratory, he joined the faculty of the Engineering Laboratory at the University of Cambridge where he is now Professor of Molecular Modelling. He is interested in first principles materials and molecular modelling, particularly in the application of machine learning methods, and also in molecular dynamics and statistical mechanics. |
09:00 - 09:30 |
Predicting material properties with the help of machine learning
A central goal of computational physics and chemistry is to predict material properties using first-principles methods based on the fundamental laws of quantum mechanics. However, the high computational costs of these methods typically prevent rigorous predictions of macroscopic quantities at finite temperatures, such as chemical potential, heat capacity and thermal conductivity. In this talk, Dr Cheng will first discuss how to enable such predictions by combining advanced statistical mechanics with data-driven machine learning interatomic potentials. As an example, the researchers computed the phase diagram of water from density functional theory at the hybrid level, accounting for thermal fluctuations, proton disordering and nuclear quantum effects. As applications in high-pressure physics, they simulated the high-pressure hydrogen system and found supercritical behaviour above the melting line and mapped the phase diagram of superionic water. Besides thermodynamic properties, Dr Cheng will talk about how to compute the heat conductivities of liquids just from equilibrium molecular dynamics trajectories. During the second part of the talk, Dr Cheng will rationalise why machine learning potentials work at all, and in particular, the locality argument. Dr Cheng will show that a machine learning potential trained on liquid water alone can predict the properties of diverse ice phases, because all the local environments characterising the ice phases are found in liquid water. ![]() Dr Bingqing Cheng, Institute of Science and Technology, Austria
![]() Dr Bingqing Cheng, Institute of Science and Technology, AustriaBingqing is an Assistant Professor at IST Austria. Prior to that, she obtained her PhD in Materials Science (Advisor: Michele Ceriotti) from EPFL in 2019. After graduation, she became a Junior Research Fellow at Trinity College, University of Cambridge, and then in 2020, a Departmental Early Career Fellow in the Department of Computer Science and Technology in Cambridge. She has won the Distinction Prize 8% for PhD thesis at EPFL, and the Volker Heine Young Investigator Award. Her research uses computer simulations to understand and predict material properties, with a particular focus on exploiting machine-learning methods to extend the scope of atomistic simulations. |
09:30 - 10:00 | Coffee |
10:00 - 10:20 |
Contributed talk: A universal and modern approach towards molecular modelling at first principles accuracy, Venkat Kapil, University of Cambridge, UK
Progress in the atomic-scale modelling of matter over the past decade has been tremendous, primarily due to improvements in techniques that compute or approximate the Born-Oppenheimer (BO) potential energy landscape (PES) and methods that describe the motion of atoms on the BO PES. Consequently, it is now possible to identify (meta)stable states, sample configurations consistent with the appropriate thermodynamic ensemble, and estimate the kinetics of reactions and phase transitions. All too often, however, progress is slowed down by the bottleneck associated with implementing new optimization algorithms and/or sampling techniques into the many existing electronic structure and empirical-potential codes. To address this problem, Venkat Kapil presents a new version of the i-PI software. This release extends the scope of the software, originally on path integral methods, towards a general and easily extensible framework that implements advanced algorithms for ‘moving’ atoms and ‘communicates’ with codes that compute the PES seamlessly through a server-client connection. The agnosticism of i-PI with the calculation of the PES allows a facile combination of advanced sampling methods with the latest developments in electronic structure methods and machine learning potentials. This leads to a universal and modern approach toward first-principles level materials modelling, as showcased by studies of complex systems such as porous materials for gas separation, pharmaceutically active molecular crystals, and monolayer water confined in graphene nanochannel. |
10:20 - 10:40 |
Contributed talk: Efficient computation of optical properties of large-scale heterogeneous systems, Joseph Prentice, University of Oxford, UK
The optical properties of large-scale (>1000 atoms) heterogeneous systems are of interest in several fields, from photovoltaics to biological systems. Computing such properties accurately from first principles, however, is challenging; even if only a small region is optically active, quantum mechanical environmental effects must often be included, and the cost of applying a quantitatively accurate level of theory is prohibitive. The work presented here demonstrates how such calculations can be performed efficiently from first principles via two methods: an extension of the spectral warping method of Ge et al, and a novel combination of quantum embedding (specifically embedded mean-field theory) and linear-scaling (time-dependent) density functional theory. The accuracy and utility of these methods is demonstrated by applying them to systems including the molecular crystal ROY, chromophores in solution, and pentacene-doped p-terphenyl. The results pave the way for quantitatively accurate calculations to be performed on previously inaccessible large-scale systems.The optical properties of large-scale (>1000 atoms) heterogeneous systems are of interest in several fields, from photovoltaics to biological systems. Computing such properties accurately from first principles, however, is challenging; even if only a small region is optically active, quantum mechanical environmental effects must often be included, and the cost of applying a quantitatively accurate level of theory is prohibitive. The work presented here demonstrates how such calculations can be performed efficiently from first principles via two methods: an extension of the spectral warping method of Ge et al, and a novel combination of quantum embedding (specifically embedded mean-field theory) and linear-scaling (time-dependent) density functional theory. The accuracy and utility of these methods is demonstrated by applying them to systems including the molecular crystal ROY, chromophores in solution, and pentacene-doped p-terphenyl. The results pave the way for quantitatively accurate calculations to be performed on previously inaccessible large-scale systems. |
10:40 - 11:30 | Discussion |
Chair
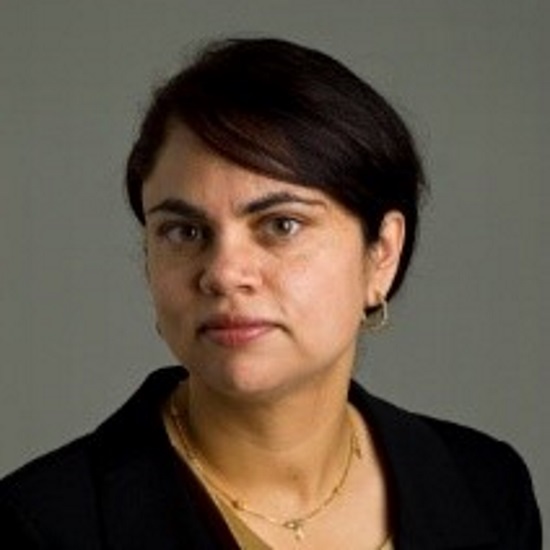
Dr Kalpana Katti, North Dakota State University, USA
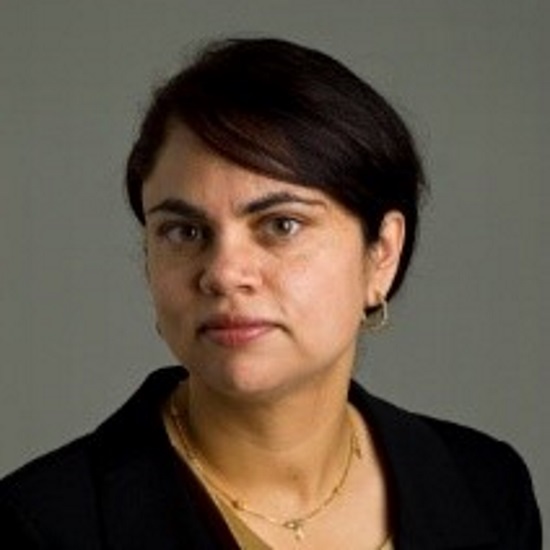
Dr Kalpana Katti, North Dakota State University, USA
Dr Kalpana Katti is a University Distinguished Professor at North Dakota State University. She leads the NDSU Center for Engineered Cancer Test-Beds (CECT) and also NSF EPSCoR Center for cellular Biointerfaces in Science and Engineering. She has published over 200 publications in Journals, conference proceedings and book chapters including 126 Journal articles. She is a NSF CAREER awardee, an elected Fellow of the American Institute for Medical and Biological Engineering (AIMBE) and recipient of the PhiKappaPhi lectureship. She has given over 70 invited, keynote and plenary lectures. Dr Katti’s primary area of research is in tissue engineering, cancer scaffolds and biomimetics. The Katti group has developed novel nanoclay based nanocomposites for biomedical applications by using nanoclays. She has also led multiscale modeling and experimental studies on biological nanocomposite systems such as seashells and bone. Her group has developed bone-mimetic scaffolds that create bone metastatic cancer testbeds. Her group has made important breakthroughs such as discovery of third-tier of hierarchy in collagen, and discovery of interlocking of platelets in nacre (in seashells). Dr Katti is actively involved in several professional societies and chairs many committees at MRS, TMS, Acers and ASCE-EMI.
12:30 - 13:00 |
Recent developments and applications of the quantum Monte Carlo method
The quantum Monte Carlo (QMC) technique is often referred to as a beyond density functional theory (DFT) method, because of its generally higher accuracy. It is becoming increasingly popular in computational material science, mainly thanks to the staggering increase and wide availablity of high performance computing, and to algorithmic developments. Because of its statistical foundations, the method is well suited to run effectively on massively parallel computers. Here Professor Alfè will briefly introduce the method, and outline recent developments that have improved its accuracy and efficiency. Professor Alfè will then present some selected systems that highlight the need for a post-DFT method, showing that QMC is indeed capable of providing the required additional accuracy. ![]() Professor Dario Alfè, University College London, UK and and University of Naples Federico II, Italy
![]() Professor Dario Alfè, University College London, UK and and University of Naples Federico II, ItalyProfessor Alfè's main interests include development of computer simulation techniques for condensed matter applications, with particular emphasis on new methods for high accuracy (eg quantum Monte Carlo), and on improving their efficiency on high performance computers. Some applications have included: 1) weak interacting systems molecular adsorption at surfaces, 2) Two dimensional systems, 3) High pressure-high temperature properties of Earth materials, 4) Structure, dynamics, melting and solid-solid phase transitions, transport properties. Professor Alfè Graduated in Physics (Laurea) at the University of Trieste in 1993, Italy, then obtained a PhD in condensed matter Physics from SISSA, Trieste in 1997, did a Post-doc University of Keele, then worked at UCL 1997-2000. Professor Alfè was Royal Society University Research fellow 2000-2006, European Young Investigator award 2006-1011, Reader in Physics 2003, Professor of Physics 2006 to date, UCL and Professor of Physics of Matter, University of Naples Federico II, Italy, 2018 to date. |
---|---|
13:00 - 13:30 |
Bringing quantum mechanics for electrons and nuclei to larger scales
Abstract not available at time of publication. ![]() Dr Mariana Rossi, Max Planck Institute for the Structure and Dynamics of Matter, Germany
![]() Dr Mariana Rossi, Max Planck Institute for the Structure and Dynamics of Matter, GermanyMariana was born Brazil and studied Physics (bachelor and masters) in the University of São Paulo. She then did her PhD in the Fritz Haber Institute of the Max Planck Society, Germany, under the supervision of Professor Volker Blum and Professor Matthias Scheffler. In her post-doc years, she worked at the University of Oxford with Professor David Manolopoulos and at the École Polytechnique Fédèrale de Lausanne with Professor Michele Ceriotti. From 2016 to 2020, she led the independent Otto Hahn Group 'Simulations from Ab Initio Approaches' in the Fritz Haber Institute, Berlin. From January 2020, she moved into a tenure-track position as head of a Lise Meitner Group in the Max Planck for Structure and Dynamics of Matter in Hamburg. Her research interests lie in the area of atomistic quantum-mechanical simulations of complex materials. |
13:30 - 14:00 |
Precise, accessible all-electron theory for real, complex materials: organic, inorganic, organic-inorganic hybrids
Abstract not available at time of publication. ![]() Dr Volker Blum, Duke University, USA
![]() Dr Volker Blum, Duke University, USAVolker Blum is an Associate Professor in the Department of Mechanical Engineering and Materials Science at Duke University, Durham, NC. He obtained his doctoral degree from University of Erlangen, Germany in 2001 and then pursued his post-doctoral research at National Renewable Energy Laboratory in Golden, CO, from 2002-2004. From 2004-2013, he was a scientist and group leader at the Fritz Haber Institute in Berlin, Germany. He develops computational methods and software for electronic structure simulations in materials science and in computational chemistry, leading the FHI-aims code project (over 150 contributors since 2004) and is involved in several other high-performance software projects in electronic structure theory and computational science. His current applied research focuses on novel semiconductor materials as well as molecular spectroscopy. |
14:00 - 14:30 | Tea |
14:30 - 15:00 | Billy McGregor, UKRI, UK |
15:00 - 15:20 |
Contributed talk: Quantifying the breakdown of electronic friction theory during molecular scattering of NO from Au(111), Connor Box, University of Warwick, UK
The Born-Oppenheimer approximation fails to capture the extent of multiquantum vibrational energy loss recorded during molecular scattering from metallic surfaces. Vibrational state-to-state scattering of NO on Au(111) has been one of the most studied examples in this regard, providing a testing ground for developing various nonadiabatic theories. The exact failings compared to experiment and their origin from theory are not established for any system, particularly since dynamic properties are affected by many compounding simulation errors, of which the quality of nonadiabatic treatment is just one. The authors use a high-dimensional machine learning representation of the energy and electronic friction tensor to minimise errors that arise from quantum chemistry. This allows us to perform a comprehensive quantitative analysis of the performance of molecular dynamics with electronic friction in describing state-to-state scattering. They find that electronic friction theory accurately predicts elastic and single-quantum energy loss, but underestimates multi-quantum energy loss and overestimates molecular trapping at high vibrational excitation. This analysis reveals potential remedies to these issues. |
15:20 - 16:00 | Discussion |
16:00 - 17:30 | Poster session |
Chair
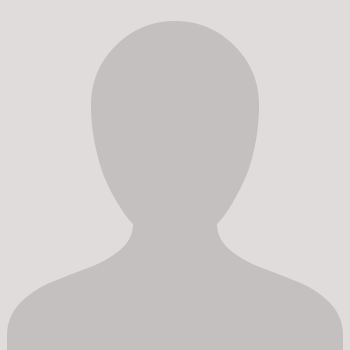
Professor Leeor Kronik, Weizmann Institute of Science, Israel
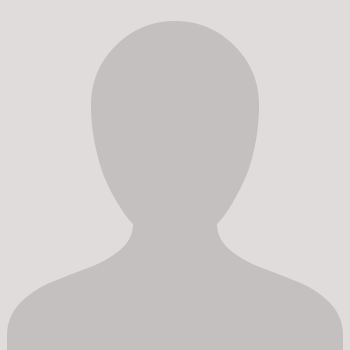
Professor Leeor Kronik, Weizmann Institute of Science, Israel
Professor Leeor Kronik directs the Beck Center for Advanced and Intelligent Materials at the Weizmann Institute of Science. He obtained his PhD at Tel Aviv University and was a Rothschild and Fulbright Post-doctoral Fellow at the University of Minnesota. His research interests are in developing density functional theory and in using it to understand and predict materials properties, with a current emphasis on organic and hybrid organic–inorganic solids and structures. He is a Fellow of the American Physical Society, and has recently received the Excellence in Research Award of the Israel Vacuum Society (2018), the Kimmel Award for Innovative Investigation (2021), and the Outstanding Scientist Award of the Israel Chemical Society (2021).
08:00 - 08:30 |
Modelling ultralong organic phosphorescence in molecular crystals of carbazole and derivatives
Ultralong Organic Phosphorescence (UOP) has generated significant interest because of its applications in several areas such as photovoltaic cells, bioimaging and anticounterfeiting.1 The molecule of carbazole (Cz) is commonly used as a building block in organic materials for optoelectronic applications, acting as a light-absorbing, electron donor and emitting moiety. Cz and its derivatives display UOP at room temperature. While the processes behind UOP have been associated with the stabilisation of H-aggregates, recent experimental studies indicate the presence of impurities drives the mechanism keeping the excited states alive for a long time.2 In this talk, Dr Crespo Otero will discuss the mechanism behind light-induced processes in crystalline and impure Cz and some derivatives using embedding methods developed in our group.3,4 Dr Crespo Otero will revisit the role of aggregation and isomeric impurities on the excited state pathways and analyse the mechanisms for exciton, Dexter energy transfer and electron transport considering Marcus and Marcus–Levich–Jortner theories.5 The researchers' excited state mechanisms provide a plausible interpretation of the experimental results and support the formation of charge-separated states at the defect/host interface. They believe these results contribute to a better understanding of the factors that enhance the excited-state lifetimes in organic materials and the role of doping with organic molecules. References 1 Kenry, C. Chen and B. Liu, Nat. Commun., 2019, 10, 2111. 2 C. Chen, Z. Chi, K. C. Chong, A. S. Batsanov, Z. Yang, Z. Mao, Z. Yang and B. Liu, Nat. Mater., 2021, 20, 175–180. 3 M. Rivera, M. Dommett, A. Sidat, W. Rahim and R. Crespo‐Otero, J. Comput. Chem., 2020, 41, 1045–1058. 4 M. Rivera, M. Dommett and R. Crespo-Otero, J. Chem. Theory Comput., 2019, 15, 2504–2516. 5 F. J. Hernández and R. Crespo-Otero, J. Mater. Chem. C, 2021, 9, 11882–11892. ![]() Dr Rachel Crespo Otero, Queen Mary, University of London, UK
![]() Dr Rachel Crespo Otero, Queen Mary, University of London, UKRachel was born and grew up in Havana, Cuba. She did her PhD in Chemistry within a collaborative program between the University of Havana and the Autonomous University of Madrid. Following two postdoctoral positions at MPI-KOFO (Germany) and the University of Bath (UK), she joined the Chemistry department at Queen Mary University of London as a Lecturer in January 2015 and was promoted to Senior Lecturer in 2019. Her research focuses on the development and use of computational techniques to understand excited state processes in organic materials with applications in OLEDs and solid-state lasers. She contributes to the development of Newton-X and fromage. |
---|---|
08:30 - 09:00 |
QM/MM simulations of materials chemistry with ChemShell
ChemShell is a scriptable computational chemistry environment with an emphasis on multiscale simulation of complex systems using combined quantum mechanical and molecular mechanical (QM/MM) methods. The use of QM/MM embedded cluster calculations for studying materials systems will be highlighted with recent results on copper-containing zeolites, where selective catalytic reduction with ammonia can reduce the emission of environmentally harmful nitrogen oxides. Insights from QM/MM calculations on the influence of solvent on the SCR mechanism will be discussed. The recent major redevelopment of ChemShell as an open source, python-based package will also be presented, including implementation of new QM/MM schemes for materials modelling and the use of multi-level parallelisation frameworks on high performance computing platforms. ![]() Dr Thomas Keal, STFC Daresbury Laboratory, UK
![]() Dr Thomas Keal, STFC Daresbury Laboratory, UKThomas Keal is a Principal Scientist in the Computational Chemistry Group at STFC Daresbury Laboratory, where he leads the team developing the QM/MM environment ChemShell and DL-FIND geometry optimisation library. His research interests include the development of new exchange-correlation functionals for density functional theory, methods for excited state optimisation and dynamics, and QM/MM modelling of biomolecules and materials, and he has contributed code to several electronic structure packages. Recently he led the project to redevelop ChemShell as a python-based, open source multiscale modelling code. His recent work focusses on the development of new embedding methods with application to transition metal controlled nitrogen chemistry in zeolites and proteins. |
09:00 - 09:30 |
Next-generation kinetic Monte Carlo approaches for understanding catalytic kinetics at unprecedented spatial and temporal scales
Kinetic Monte-Carlo (KMC) simulations have been instrumental in multiscale catalysis studies, enabling the elucidation of the complex dynamics of heterogeneous catalysts and the prediction of macroscopic performance metrics, such as activity and selectivity. However, the accessible length- and time-scales are still limited, and handling lattices containing millions of sites with 'traditional' sequential KMC implementations becomes prohibitive due to large memory requirements and long simulation times. Domain decomposition approaches could address these limitations, but they are challenging to implement in KMC simulation due to the inherently sequential nature thereof, by which one reactive event is causally linked to future (and past) events. These causal relations and the random time advancements of KMC steps, necessitate sophisticated protocols for conflict resolution at the boundaries between subdomains. Jefferson’s Time-Warp algorithm overcomes these challenges using local operations: sending and receiving messages/anti-messages, saving simulation state snapshots, and rolling-back in time to reinstate a previous state. Thus, any causality violations, arising transiently during simulation, are corrected, and the exact dynamics of the underlying stochastic model (the master equation) are finally reproduced. In this work, the researchers have coupled the Time-Warp algorithm with the Graph-Theoretical KMC framework enabling the handling of complex adsorbate lateral interactions and reaction events within large lattices. This approach has been implemented in Zacros, their general-purpose KMC software, and has been validated and benchmarked for efficiency in model systems as well as realistic chemistries. This work makes Zacros the first-of-its-kind general-purpose KMC code with distributed parallelisation capability to study heterogeneous catalysts. ![]() Dr Michail Stamatakis, University College London, UK
![]() Dr Michail Stamatakis, University College London, UKDr Michail Stamatakis obtained a Diploma in Chemical Engineering from the National Technical University of Athens (Greece) in 2004, and joined Rice University (USA) for his Doctoral studies, which he completed in 2009. He subsequently carried out post-doctoral research at the University of Delaware (USA) and joined the Department of Chemical Engineering at University College London (UK) as a Lecturer in 2012. His research revolves around the development and application of multiscale modelling methods for understanding complex phenomena in catalysis and materials science. Within this context, he has studied catalytic materials based on transition metals and alloys, but also dichalcogenides and carbides as 'niche' formulations, for reactions encountered in biomass conversion to fuels and chemicals, gas (methane) upgrade to synthesis gas and liquid fuels, as well as emissions control. A key enabling factor of these studies has been the kinetic Monte Carlo software Zacros that he has established for the simulation of surface phenomena and chemical reactions on heterogeneous catalysts. The software has been commercialized by UCL Business and is now licensed to more than 600 users worldwide. |
09:30 - 10:00 | Coffee |
10:00 - 10:30 |
Advanced software, machine learning, powerful computers: enough for materials modelling?
Modeling has two main objectives, the rationalisation of existing results and the prediction of new systems and new phenomena. The field has made tremendous progress, and the availability of powerful computers, sophisticated software solutions, and machine learning algorithms are contributing to the discovery of new materials. However, the widespread use of modeling tools can also lead to inaccurate or useless predictions with potentially negative effects on the credibility of the field. In this talk Professor Pacchioni will briefly illustrate this emerging problem using materials for catalysis as an example. A large number of variables determine the performance of a heterogeneous catalyst, posing a challenge for quantum chemical modeling. The complexity of the problem has been significantly reduced with the advent of single atom catalysts (SACs) and, in particular, graphene-based SACs. In this context we are witnessing an increasing tendency to screen large number of materials based on density functional theory (DFT) and to propose universal descriptors to provide a guide for the synthesis of new catalysts. Professor Pacchioni will critically analyse some of the current problems associated with this activity: accuracy of calculations, neglect of important contributions in the models used, physical meaning of the proposed descriptors, imprecise data sets used to train machine learning algorithms, not to mention a few serious data reproducibility problems. The final message is that in an era where computing power has grown enormously, perhaps the time has come to shift the focus from the quantity to the quality of the data produced, to be of real help to the experimentalist in the design of new catalytic 1 1G. Di Liberto, Luis A. Cipriano, G. Pacchioni, “Universal principles for the rational design of single atom electrocatalysts? Handle with care”, ACS Catalysis, 12, 5846-5856 (2022). ![]() Professor Gianfranco Pacchioni, Università di Milano-Bicocca, Italy
![]() Professor Gianfranco Pacchioni, Università di Milano-Bicocca, ItalyGianfranco Pacchioni received his PhD at the Freie Universität Berlin in 1984. He is active in the field of modeling of heterogeneous catalysis and oxide materials. He worked at the IBM Almaden Research Center, the Technical University of Munich, the Fritz-Haber Institute (Berlin), etc. He is Full Professor at the University of Milano-Bicocca where he has been Vice Rector for Research (2013-2019) and Director of the Department of Materials Science (2003-2009). Editor-in-chief of the Journal of Physics: Condensed Matter (Institute of Physics, UK); co-author of about 550 papers (>30000 citations; h-index WoS 89; Google Scholar 100); he has given about 500 invited talks. He received several awards (Humboldt Award, Pascal Medal, etc.); Fellow of the Accademia Nazionale dei Lincei (2014), the Academia Europaea (2012), the European Academy of Sciences (2009). |
10:30 - 10:50 |
Contributed talk: Using cluster expansions to predict the structure and properties of PdZn bimetallic catalysts, Lara Kabalan, Cardiff University, UK
Metals alloys are known to improve the performance of heterogenous catalysts for many different reactions, such as CO2 hydrogenation1,2. Predicting an effective and novel catalyst, by computing combinations of elements and compositions using Density Functional Theory (DFT), is expensive and highly time-consuming. To accelerate the discovery process, one can couple DFT with cluster expansion (CE), which the authors demonstrate here in the identification of an effective and novel catalyst for CO2 hydrogenation. The authors focus on the PdZn (101) and (110) facets, which are most stable for binary alloys, and CE models for both of facets were tested in the concentration range where the body-centred tetragonal (BCT) phase exists, which is from 40 to 50% Zn concentration. These results show that higher Pd concentrations are predicted for both facets in the sub-surface while the top layers remain chemically ordered in a 1:1 alloy; the behaviour changes outside the stable BCT range, where segregation of Zn on the top surface is predicted for Zn concentrations above 50%. The outcomes highlight the critical importance of CE approach for determining structure−property relationships and accelerating the discovery and design of surfaces and nanostructured materials. 1GA Olah, Angew. Chem., Int. Ed. (2005), 44, 2636. |
10:50 - 11:30 | Discussion |
Chair
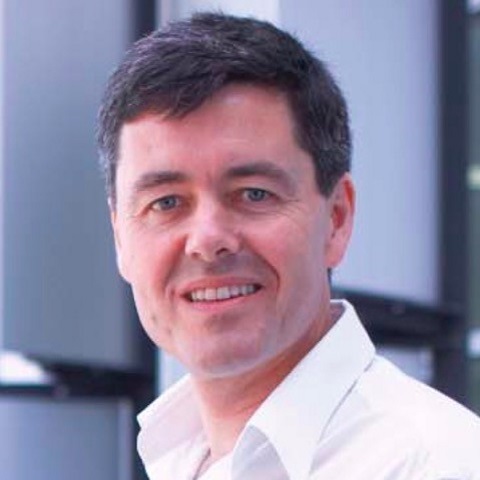
Professor Julian Gale, Curtin University, Australia
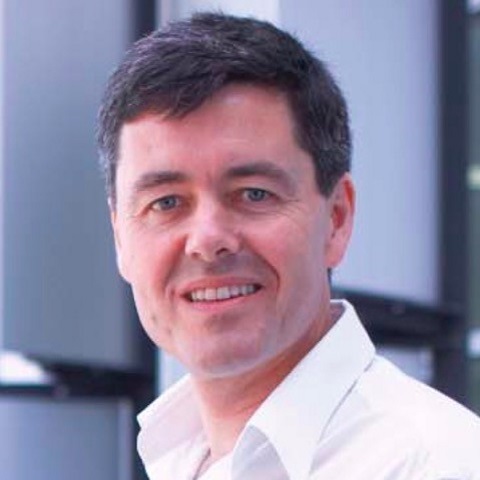
Professor Julian Gale, Curtin University, Australia
Professor Julian Gale obtained his first degree from the University of Oxford in Natural Sciences (Chemistry), where he continued on to study for a DPhil in the Department of Chemical Crystallography. After a postdoctoral position at the Royal Institution of Great Britain he moved to Imperial College London as a Royal Society University Research Fellow and subsequently Reader in Theoretical and Computational Chemistry. In 2003, he moved to his current location, Curtin University, as a Premier’s Research Fellow and now holds the position of John Curtin Distinguished Professor of Computational Chemistry. Recently he was awarded an Australian Research Council Laureate Fellowship and is a Fellow of the Australian Academy of Science. Research interests include the development and application of computational techniques to problems in areas including materials chemistry, crystallisation, geochemistry and mineralogy.
12:30 - 13:00 |
Molecular crystal structure prediction for guiding materials discovery
Abstract not available at time of publication ![]() Professor Graeme Day
![]() Professor Graeme DayProfessor Graeme Day joined the University of Southampton in 2012. As a member of the Computational Systems Chemistry research group, his research develops computational methods for materials discovery and prediction of the crystal structures. Graeme Day received his BSc in Chemistry, Mathematics and Computing Science from Saint Mary's University, Halifax, Canada, after which he studied for an MSc in Theoretical Chemistry from the University of Oxford and obtained his PhD in 2003 from University College London. He then moved to the University of Cambridge for postdoctoral work and was awarded a Royal Society University Research Fellowship in 2005, which he held in Cambridge until 2012. In 2012 he moved to the University of Southampton as a Reader and was promoted to Professor of Chemical Modelling in 2014. His research concerns the development of computational methods for modelling the organic molecular solid state. A key focus of this work is the prediction of crystal structures from first principles; his research group applies these methods in a range of applications, including pharmaceutical solid form screening, NMR crystallography and computer-guided discovery of functional materials. |
---|---|
13:00 - 13:20 |
Contributed talk: DMC-ICE13: ambient and high pressure polymorphs of ice from Diffusion Monte Carlo and Density Functional Theory, F Della Pia, University of Cambridge, UK
Ice is one of the most interesting molecular crystals for a wide range of scientific fields, including physics, chemistry, biology, as well as space and material science. Its electronic properties are determined by complex topologies of hydrogen bonds as well as non-local dispersion interactions, providing a stern set to test electronic structure theory methods and get general insights both on molecular crystals and liquid water, where high accuracy simulations are hindered by prohibitive computational cost. Moreover, the recent discoveries of new ice phases have renewed the interest and increased the already extreme complexity of the water phase diagram, calling for a new analysis on the performance of electronic structure methods on the stability of the known polymorphs. The DMC-ICE13 dataset was constructed by computing reference lattice energies of thirteen ice polymorphs with sub-chemical accuracy using Diffusion Monte Carlo and conducting an extensive benchmark of several Density Functional Theory functionals. The obtained results suggest that a single functional achieving reliable performances for all phases is still missing, and that care is needed in the selection of the most appropriate functional for the desired application. |
13:20 - 13:40 |
Contributed talk: Crumbling crystals, Niamh O'Neill, University of Cambridge, UK
Dissolution is a ubiquitous phenomenon, and important biological and physical processes rely on dissolution of ionic salts in water. Simulations lend themselves conveniently to studying dissolution, providing the spatio-temporal resolution that can be difficult or impossible to obtain experimentally. Nevertheless, it's a complex process, requiring ab initio level of treatment and long time simulations, typically hindered by computational expense. The authors use advances in machine learning potential methodology to resolve the entire dissolution mechanism of NaCl in water under multiple conditions with an ab initio equivalent accuracy. A general overall mechanism pervades whereby a steady ion-wise unwrapping of the crystal precedes its rapid disintegration. This disintegration is characterised by the crystal reaching a point of maximum instability, where destabilising ion-water interactions dominate over the cohesive ion-ion interactions of the crystal. From high to low concentrations, dissolution times can be categorised as highly stochastic to almost completely deterministic respectively. They show the stochastic extent of the overall mechanism can be reasoned by simple arguments with respect to microscopic sub-events during the dissolution. This study provides important insight into a long-standing fundamental scientific problem. Moreover, this has important implications on our general understanding of dissolution in other crystals, as well as crystal nucleation. |
13:40 - 14:10 | Tea |
14:10 - 15:00 | Panel discussion/Overview (future directions) |