Links to external sources may no longer work as intended. The content may not represent the latest thinking in this area or the Society’s current position on the topic.
Soft interfacial materials: from fundamentals to formulation
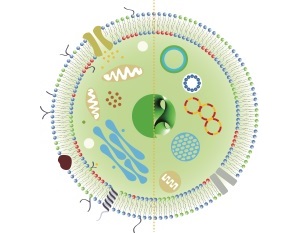
Scientific discussion meeting organised by Professor Michael Cates FRS, Professor John Seddon, Dr Nicholas Brooks, Dr Paul Clegg and Professor Alex Lips
The science of soft interfaces (lipid membranes, emulsions, particle‐stabilised droplets etc) is rapidly moving into an era of predictive capability that allows the design and development of advanced materials to be based on secure scientific knowledge. The meeting will not only address fundamental science, focussing on generic design principles for self‐organisation and interfacial structure, but also explore the resulting prospects for 'informed formulation' of new and improved industrial products.
You can download the draft programme (PDF), and biographies of the organisers and speakers are available below, together with speaker abstracts.
Attending this event
This meeting has already taken place. Recorded audio of the presentations can be found below, and papers from the meeting will be published in a future issue of Philosophical Transactions A.
Enquiries: Contact the events team
Organisers
Schedule
Chair
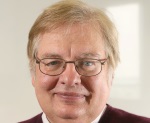
Professor Dominic Tildesley CBE FRSC, EPFL-CECAM, Switzerland
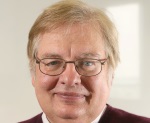
Professor Dominic Tildesley CBE FRSC, EPFL-CECAM, Switzerland
Dominic Tildesley received his chemistry degree from Southampton in 1973 and a DPhil from Oxford in 1976. He worked at the University of Southampton and Imperial College London until 1998. Subsequently, he joined Unilever and became Chief Scientist of the Home and Personal Care Division. In 2013 he moved to the EPFL Switzerland as director of CECAM (Centre Européen de Calcul Atomique et Moleculaire). He received the Marlow and Tilden Medals of the RSC and was awarded a CBE for services to science, technology and business in 2014. He is President of the Royal Society of Chemistry.
09:05 - 09:30 |
Self assembled structures to increase nutrient efficiency
A major challenge in the development of drug, nutrient or flavour delivery systems is to guarantee bioactive efficacy. The fast degradation and poor bioavailability are limitations, which need to be overtaken through the use of appropriate vehicles. Classical micro-encapsulates obtained by powder technology and encapsulation of the compounds into an amorphous matrix, represent the majority of encapsulation systems. Their ease of handling/dosing and their good stability in dried applications make them ideal for many products. However, these technologies are costly and usually not efficient in products with high water activity and liquids in general. In the present contribution, we will introduce another type of delivery systems, lipid self-assembled structures, and discuss their advantages and limitations. These delivery systems can be obtained by structuring oil present in products, often resulting in a price advantage. This presentation will focus on their specific features to deliver benefits such as increased yield of Maillard reaction products and controlled release. In particular, systems made with oil and lecithin show good performance in terms of sustained delivery of caffeine. In addition, they have the advantages to be easily processed and can be made from ingredients that are naturally present in many food products. ![]() Dr Laurent Sagalowicz, Nestlé Research Center, Switzerland
![]() Dr Laurent Sagalowicz, Nestlé Research Center, SwitzerlandLaurent Sagalowicz has 25 years of experience in research. He works in the fields of self-assembled structures, food encapsulation, vitamins and healthy lipids. He is also an expert in structure and characterisation with a special emphasis on the use of advanced electron microscopy. He has published about 80 peer-reviewed scientific articles and book chapters. His most recent publications concern lipid self –assembled structures, such as reversed bicontinuous cubic structures, micelles and their applications. He is also reviewer for a number of scientific journals. He is currently at Nestlé Research Center (Switzerland) in the micronutrient fortification group. |
|
---|---|---|
09:45 - 10:15 |
Predicting non-ionic surfactant phase diagrams
Agrochemical formulations are often optimised for biological performance. Surfactants are effective adjuvants for pesticide uptake into plants however they display complex phase behaviour and this can be a problem. Where they form hexagonal or cubic phases in water the associated viscosity is usually too high. A model which relates surfactant chemical structure to phase behaviour was developed. The model describes the shape of the surfactant and from this the phase structure under different conditions can be predicted. The model requires four parameters in order to estimate the shape of the surfactant. These parameters are simple counts of the number of carbon atoms or ethoxylate groups in the molecule. The model uses the shape, the temperature, and the concentration, to estimate which type of phase is most likely to be present. The model can be used to design or screen surfactants such that they are easy to build-in to formulations. A commercially important design is used as an example. ![]() Dr Gordon Bell, Syngenta, UK
![]() Dr Gordon Bell, Syngenta, UKDr Gordon Bell is a research scientist with Syngenta, based at the Jealotts Hill International Research Centre. He has over thirty years’ experience of formulation chemistry and product design. The products and technology he has developed in the areas of water dispersible granules, emulsion concentrates and microcapsules are supported by 30 patents. His areas of interest are formulation design, biological optimisation, product safety, solubility prediction, CAMD and modelling. He is an assistant editor of Pesticide Management Science. |
|
11:00 - 11:30 |
The physics of stratum corneum lipid bilayer membranes
The skin is a remarkable organism. The outer 40-100 microns constitute a protective layer, the stratum corneum, which is a composite material of keratin bodies (10-100 microns) embedded in a matrix of lipid bilayers. These bilayers are very different from the fluid phospholipid bilayers familiar from the plasma membrane that surrounds living cells, or that constitute organelles such as the Golgi apparatus and endoplasmic reticulum. I will discuss the physical properties of these fascinating materials which we have studied using extensive Molecular Dynamics simulations, and indicate how special features such as asymmetric lipids and extensive hydrogen bonding are essential for humidity control, flexibility, and biological function of skin, and help make skin the remarkable self-healing material that it is. ![]() Professor Peter Olmsted, Georgetown University, USA
![]() Professor Peter Olmsted, Georgetown University, USAPeter Olmsted received his PhD in Physics from the University of Illinois at Urbana-Champaign in 1991, where he studied the theory of liquid crystal hydrodynamics and non-equilibrium phase transitions. Following post-docs at Exxon, Cambridge, and the University of Michigan, where he worked on a variety of soft matter physics problems (membrane, polymers, biophysics, and liquid crystals), he moved to Leeds in 1996 for a University Research Fellowship. He became Professor in 2005, and led the Soft Matter Physics group in Leeds from 2008-2013. In 2014 he moved to Georgetown University in Washington DC, as Joseph Semmes Ives Chair in Physics, where he joined the new Institute for Soft Matter Synthesis and Metrology. His current research is mainly theory and computer simulation, and includes rheology, dynamics and instabilities in soft matter, polymers, lipid membranes and proteins. He works closely with experimentalists, and is always on the lookout for new problems! |
|
11:45 - 12:15 |
Model biomimetic lipid membranes based on quaternary lipid-water systems for protein encapsulation
Self-assembled lipidic bicontinuous cubic phases are attracting increasing interest as biocompatible carriers of large biomolecules including proteins, peptides, DNA and drugs. The lipid bilayer structure of these materials is particularly useful for the encapsulation of amphiphilic proteins and peptides as it mimics their native cell membrane environment. The lipidic cubic phase has been observed in nature, including in virally infected cells. As well as potentially retaining the protein in a functionally active form, the lipidic material can be biocompatible, and may protect the protein against degradation. However lipidic cubic phase systems formulated to date have been mainly based on a single lipid, or ternary systems comprising two lipids and water. Such materials are not representative of the native cell membrane which can contain up to one hundred different lipids. Herein we investigate the phase behaviour of more complex lipidic cubic phases comprised of three different lipids in excess water. High-throughput techniques have been exploited to screen the large compositional space associated with these systems. Lipidic cubic phases based around a more biomimetic bilayer is of potential use in a wide range of applications including pharmaceutical (drug delivery, gene therapy and medical imaging), materials science (biosensors), biology (long-term storage of fragile proteins, crystallisation) and chemistry/physics (fundamental protein – lipid interactions). ![]() Professor Calum Drummond, RMIT, Australia
![]() Professor Calum Drummond, RMIT, AustraliaProfessor Drummond’s research interests are in the area of advanced materials, including application to energy storage and biomedical products. He has been an author of over 200 publications. Professor Drummond is a graduate of The University of Melbourne (BScEd , BSc Hons, PhD and DSc). He has been an Australian Research Council (ARC) Queen Elizabeth II Fellow and an ARC Federation Fellow. Professor Drummond has a strong interest and passion for the commercialisation of research outcomes. He joined RMIT University, as Deputy Vice-Chancellor Research and Innovation, in 2014 from CSIRO where he was Group Executive for Manufacturing, Materials and Minerals. Immediately prior to this CSIRO Group Executive appointment, he was Chief of CSIRO Materials Science and Engineering (CMSE). Prior to becoming a Chief, Calum was seconded from CSIRO to be the inaugural Vice President Research at CAP-XX, an Intel portfolio company. CAP-XX develops supercapacitors for consumer electronic products. In 2006 CAP-XX listed on the London Stock Exchange. |
Chair
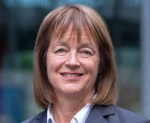
Professor Alice Gast, Imperial College London, UK
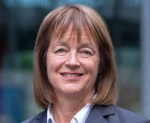
Professor Alice Gast, Imperial College London, UK
Professor Alice P Gast became President of Imperial College London on 1 September 2014.
Prior to her appointment at Imperial, Professor Gast was the 13th President of Lehigh University, Pennsylvania, USA, from August 2006 to August 2014. Other leadership roles include serving as the Vice-President for Research and Associate Provost and Robert T Haslam Chair in Chemical Engineering at the Massachusetts Institute of Technology from 2001 to 2006. She was a professor of chemical engineering at Stanford University from 1985 to 2001 and affiliated with the Stanford Synchrotron Radiation Laboratory. Professor Gast is a member of a number of international advisory committees and boards. In 2012, she was appointed to the board of directors of Chevron Corporation.
13:30 - 14:00 |
Cationic membrane-nucleic acid complexes used in gene delivery and gene silencing
Cationic liposomes (CLs) are studied worldwide as carriers of DNA and short interfering RNA for gene delivery and gene silencing, and related clinical trials are ongoing. Optimisation of transfection efficiency and silencing efficiency requires elucidation of the interactions of CL–nucleic acid nanoparticles (NPs) with cell membranes including events leading to release of active nucleic acids within the cytoplasm. As an introduction, synchrotron x-ray scattering data will be described, which reveals the distinct liquid crystalline phases of CL–nucleic acid complexes including the lamellar, inverse hexagonal, hexagonal, and gyroid cubic phases. This talk will describe experiments with surface-functionalised PEGylated CL–DNA nanoparticles (NPs) [R. N. Majzoub et al. Biomaterials 2014, 35, 4996-5005], including fluorescence microscopy co-localisation with members of the Rab GTPases, which has revealed CL–DNA pathways and interactions with cells [R. N. Majzoub et al. Biochim. Biophys. Acta – Biomembranes 2015, 1848, 1308-1318]. The functionalisation, achieved through custom synthesis, is intended to address and overcome cell targeting and endosomal escape barriers to nucleic acid delivery faced by NPs designed for in vivo applications. This work was done in collaboration with Ramsey N. Majzoub and Kai K. Ewert. Funded by the US National Institutes of Health. ![]() Professor Cyrus Safinya, University of California, Santa Barbara, USA
![]() Professor Cyrus Safinya, University of California, Santa Barbara, USACyrus R Safinya is a Professor in the Materials, Physics, and Molecular, Cellular, and Developmental Biology Departments at the University of California Santa Barbara (UCSB). He obtained a B.S. in Physics and Mathematics from Bates College (1975). His PhD thesis research was performed under the supervision of Professor Robert J Birgeneau (UC Berkeley Chancellor-Emeritus) at MIT (1981). He joined the Exxon Research and Engineering Company in 1981 and conducted research on structures and interactions in complex fluids and biological membranes. He joined UCSB in 1992. Current research in his group is centred on (1) the elucidation of key parameters, which control the interactions between proteins derived from the eukaryotic nerve cell cytoskeleton and lead to hierarchical structures, with the ultimate goal of relating structure to function, and (2) developing lipid-based carriers of functional DNA and short-interfering RNA for gene delivery and silencing applications. |
|
---|---|---|
14:15 - 14:45 |
Some physics mechanisms behind membrane shaping
Membrane proteins and lipids are internalised, externalised or transported within cells, not by bulk diffusion of single molecules, but embedded in the membrane of small vesicles or thin tubules. The formation of these "transport carriers" follows sequential events: membrane bending, fission from the donor compartment, transport and eventually fusion with the acceptor membrane. Similar sequences are involved during the internalisation of drug or gene carriers inside cells. These membrane-shaping events are generally mediated by different proteins binding to the membrane. The mechanisms behind these biological processes of membrane transformation are actively studied both in the cell biology and the biophysics contexts. Different physical methods have been developed in the past years to quantify and model the action of these proteins, in particular soft membrane nanotube with controlled curvature pulled from giant vesicles (GUVs). I will show in one example how simple soft matter principles can account for membrane deformation by proteins, and how it is possible to measure the intrinsic curvature of these proteins using membrane nanotubes combined with optical tweezers and confocal microscopy. Eventually, I will also present our current understanding of membrane scission, a necessary step for the detachment of the carrier from the parent membrane and its further trafficking inside the cell. ![]() Professor Patricia Bassereau, Institut Curie, France
![]() Professor Patricia Bassereau, Institut Curie, FrancePatricia Bassereau is currently CNRS Directrice de Recherche (equivalent to professor) at the Institut Curie in Paris where she is the leader of the group 'Membranes and cellular functions'. She started her carrier in Soft Matter in Montpellier (GDPC) and spent one year as a visiting scientist at the IBM Almaden Center (San Jose, USA). She moved to the Institut Curie in 1993 to work on questions related to 'Physics of the cell'. She develops a multidisciplinary approach, largely based on synthetic biology and on the development and study of biomimetic systems, as well as quantitative mechanical and microscopy methods to understand the role of biological membranes in cellular functions. Additionnally, she studies the mechanics of single filopodia in living cells, and in vitro the generation of cell protrusions. |
|
15:30 - 16:00 |
Delivery and controlled release from nanoparticles for drug delivery and imaging
Nanoparticles are becoming increasingly important in drug delivery and multimodal delivery and imaging. In some cases nanoparticles afford rapid release of very hydrophobic therapeutics, which is required for effective treatment. In other cases controlled/sustained release is required to maintain drug concentrations in vivo for appropriate times. And still in other cases it is desirable to retain the drug in the nanoparticle and to have some form of triggered release; for example, when targeting a cancer tumour. We will present an overview of nanoparticle formulations to achieve these goals. The process we have developed based on block-copolymer-directed, kinetically-controlled self-assembly, called Flash NanoPrecipitation (FNP), enables the production of 50-400 nm nanoparticles. Successful nanoparticle production involves controlling micromixing to effect supersaturations as high as 10,000 in 1.5 ms, and then controlling nucleation and growth rates to match block copolymer assembly rates. The rapid assembly enables the encapsulation of multiple drugs and imaging agents into the same nanoparticle, and the production of multivalent targeted nanoparticles. The flexibility of the assembly process enables the preparation of imaging nanoparticles based on fluorescence, PET, x-ray, and MRI. Finally, the drug release from nanoparticles poses some special problems. Most release data of hydrophobic drugs from nanoparticles erroneously report release rates that are dominated by mass transfer artefacts. Techniques to accurately assess release rates will be presented. ![]() Professor Robert K Prud'homme, Princeton University, USA
![]() Professor Robert K Prud'homme, Princeton University, USARobert K Prud'homme is a professor in the Department of Chemical and Biological Engineering at Princeton University and Director of the Engineering Biology Program at Princeton. He received his BS at Stanford University and his PhD from the University of Wisconsin at Madison under Professor Bob Bird. He has served on the executive committees of the American Institute of Chemical Engineers Materials Science Division and the U.S. Society of Rheology and was the President of the U.S. Society of Rheology. He served as the chair of the Technical Advisory Board for Material Science Research for Dow Chemical Company, which directs Dow’s materials research programs, and he was on the Board of Directors of Rheometric Scientific Inc., the leading manufacturer of rheological instrumentation. He also served on the Nanotechnology Scientific Advisory Committee for BASF, which provided guidance for future trends in nanotechnology for the company. His awards include the NSF Presidential Young Investigator Award, Princeton School of Engineering and Applied Science Outstanding Teaching Award, the Sydney Ross Lectureship at RPI, the Bird, Stewart and Lightfoot Lecturer at the University of Wisconsin, the Dinesh Shah lectureship at the University of Florida, and the Midland Macromolecular Institute Visiting Professor in Midland Michigan. He has been the organiser and Chair of the Gordon Conference on Ion Containing Polymers, and the Society of Petroleum Engineers Forum on Stimulation Fluid Rheology, in addition to organising numerous sessions at AIChE, ACS, and SOR meetings. He directed the Princeton-University of Minnesota-Iowa State NSF NIRT Center on nanoparticle formation. His research interests include rheology and self-assembly of complex fluids. Systems of interest are biopolymer solutions and gels, surfactant mesophases, and polymer/surfactant mixtures. The goals of the studies are to understand how weak molecular-level interactions can be used to tune macroscopic bulk properties and phase behaviour. Application of the work is directed at nanoparticle formation for drug delivery, controlled release, targeting, and imaging. |
Chair
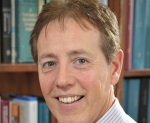
Professor Bernard Binks, University of Hull, UK
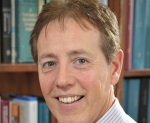
Professor Bernard Binks, University of Hull, UK
Professor Bernie Binks leads the Surfactant & Colloid Group in the Department of Chemistry, University of Hull. His research interests include surfactants, microemulsions, emulsions, foams, dispersions and the behaviour of colloidal particles at fluid interfaces. The latter involves particle-stabilised emulsions and foams and novel materials derived thereof, including dry water and powdered oil. He works in close collaboration with industry in the food, pharmaceutical, cosmetic and agrochemical sectors. He was awarded the RSC Faraday Division Surfaces and Interfaces Award in 2014 for his pioneering contributions in the field of particle-stabilised emulsions.
09:00 - 09:30 |
Inclusions in soft matter: opportunities for directed assembly
Colloidal particles can be manipulated using external fields to steer them into well-defined structures at given locations. We are developing alternative strategies based on fields that arise when a colloid is placed within soft matter to form an inclusion that generates a potential field. For example, a particle on a fluid interface can distort that interface to satisfy its wetting boundary conditions. The distortion has an associated energy field given by the product of its interfacial area and the surface tension. Fields generated by neighboring particles interact to drive assembly; preferred orientations for anisotropic objects emerge. The particle’s capillary energy depends on the local interface curvature. By molding the interface, we can define fields that drive microparticles along pre-determined paths to well defined locations. This example captures the emergent nature of the interactions, and their potential importance in schemes to make reconfigurable materials, since interfaces and their associated capillary energy landscapes can be readily reconfigured. There are important analogies in other soft matter systems. Particles in liquid crystals distort the director field and form defect structures that elicit an elastic energy response that can be used to define particle paths and sites for assembly. Particles adhered to lipid bilayer vesicles are another system in which such fields can be generated and exploited. These example systems have important analogies and pronounced differences which we seek to understand and exploit. ![]() Professor Kathleen Stebe, University of Pennsylvania, USA
![]() Professor Kathleen Stebe, University of Pennsylvania, USAKathleen J Stebe received a BA in Economics from the City College of New York, Magna cum Laude, and a PhD in Chemical Engineering at the Levich Institute, also at CCNY, under the guidance of Charles Maldarelli. Thereafter, she spent a post-doctoral year in Compiegne, France working with Dominique Barthès Biesel. Professor Stebe joined the Department of Chemical Engineering at Johns Hopkins University, where she rose through the ranks to become Professor and to serve as the department chair. In 2008, Professor Stebe joined the faculty at the University of Pennsylvania as the Richer and Elizabeth M Goodwin Professor of Engineering and Applied Science. From 2008-2012, Professor Stebe served as the department chair of Chemical and Biomolecular Engineering. In July 2012, she assumed the post of Deputy Dean for Research in the School of Engineering and Applied Science. Professor Stebe has been a Fellow at the Radcliffe Institute for Advanced Studies; she has received the Robert S Pond Excellence in Teaching Award at JHU, the Frenkiel Award from the Division of Fluid Dynamics of the American Physical Society, and was named a Fellow of the APS. Professor Stebe’s research focuses on capillary phenomena, including how anisotropic particles interact and assemble at interfaces, in complex fluids, including liquid crystals and lipid bilayers. She is an expert in interfacial flows, with particular emphasis on how surfactants can be used to direct interfacial flows, and how surfactants alter drop break up modes. Other aspects of her research address dynamic surface tension, rheology of protein laden interfaces, and the design of interfaces and bounding surfaces for biological and materials applications. |
|
---|---|---|
09:45 - 10:15 |
Arrested coalescence in microstructured emulsions
Arrested droplet coalescence has been shown to produce stable anisotropic shapes and to be a key mechanism for microstructure development in foods, petroleum, and pharmaceutical formulations. This work builds on a recent study of the dynamic arrest of binary droplet coalescence by internal elastic structures and examines the formation of multi-droplet connections in a microstructured oil-in-water emulsion. Arrested droplet connections are shown to be a strong function of interfacial and rheological variables and their history. New individual mechanisms of arrested droplet connectivity are demonstrated and used to construct a multi-droplet model of emulsion microstructure for applications in advanced formulated products. ![]() Professor Patrick Spicer, UNSW, Australia
![]() Professor Patrick Spicer, UNSW, AustraliaPat Spicer completed a PhD in Chemical Engineering at the University of Cincinnati where his research focused on design and synthesis of colloidal and nanoparticle materials. After defending his thesis, he went to work for the Procter & Gamble Company, leading their Crystallization group in the Corporate Engineering Division and supporting the scale-up and manufacture of complex fluid products for all of P&G's product areas. In 2006 Pat set up P&G's Microfluidics and Structured Fluids group, responsible for development, characterisation, scale-up, and modelling of microstructured fluids and processes. After 15 years with P&G, Pat began work as an Associate Professor of Chemical Engineering at UNSW Australia in Sydney. There his research focuses on the design and development of microstructured fluid materials by understanding their kinetic behaviour. |
|
11:00 - 11:30 |
Soft electrostatic repulsion in particle monolayers at liquid interfaces: surface pressure and effect of aggregation
![]() Professor Peter Kralchevsky, Sofia University, Bulgaria
![]() Professor Peter Kralchevsky, Sofia University, BulgariaPeter Kralchevsky is a full professor in the Department of Chemical Engineering in Sofia University (Sofia, Bulgaria) and Fellow of the Bulgarian Academy of Sciences. His research expertise is the field of physical chemistry and colloid science, with contributions in the investigation of surface forces acting in liquid films and between colloidal particles; capillary forces between particles attached to liquid interfaces and formation of two-dimensional particle arrays; thermodynamics and kinetics of surfactant and protein adsorption, micellisation, solubilisation, etc. So far, he has published 188 research and review papers, one book (Elsevier), with more than 6500 citations of his publications; h-index = 38. He has been leader and participant in many European and industrial projects: He was the Chair of COST Action D43 “Colloid and Interface Chemistry for Nanotechnology”, Vice Chair of COST Action CM1101 (2012–2016). In 2010, he was elected Secretary of the European Colloid and Interface Society (ECIS). |
|
11:45 - 12:15 |
Proteins as switchable Janus ellipsoids
Janus particles are micro- or nano-scale particles whose surfaces have two or more distinct physical properties. Such asymmetry results in interesting self-assembly properties, but homogeneous Janus particles can be difficult to synthesise. The protein BslA (Bacterial Surface Layer A) is a small (~4 nm) protein produced by the bacterium Bacillus subtilis that has a hydrophilic ‘body’ to which is appended a surface-exposed hydrophobic ‘cap’. These properties allow the ellipsoidal protein to partition to oil- and air-water interfaces where it self-assembles to form a robust, elastic, and highly hydrophobic film. We have investigated the behaviour of BslA using a combination of biophysical experiments and multiscale simulations. I will describe how BslA provides an intriguing example of a colloidal particle with switchable, environmentally-responsive physical features that have potential applications in nanoscale self-assembly. ![]() Professor Cait McPhee, Professor of Biological Physics, University of Edinburgh, Royal Society Dorothy Hodgkin Fellow 1999-2001
![]() Professor Cait McPhee, Professor of Biological Physics, University of Edinburgh, Royal Society Dorothy Hodgkin Fellow 1999-2001Cait MacPhee is a Professor of Biological Physics at the University of Edinburgh. She started her undergraduate life in Australia in the biosciences, gradually moving across to physics during the course of her career. Her postdoc was in the Oxford Centre for Molecular Sciences with Professor Chris Dobson, where she was awarded a Dorothy Hodgkin Fellowship by the Royal Society. Her first academic post was in the Cavendish Laboratory at the University of Cambridge, where she co-founded the Biological Physics Group with Tom Duke. She was awarded a University Research Fellowship by the Royal Society in 2002. In 2005 she moved to the School of Physics and Astronomy at the University of Edinburgh, where she remains to the present day. She has interests in protein structure-function relationships, protein evolution, nanobiotechnology and astrobiology. Cait tweets as @sciorama. |
Chair
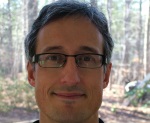
Dr Jeffrey Fowler, Syngenta, USA
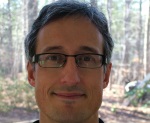
Dr Jeffrey Fowler, Syngenta, USA
Dr Jeffrey Fowler is a chemical engineer with degrees from Imperial College London, Washington University and Stanford University (PhD). Except for a few years in Switzerland he has worked in the USA for approximately equal periods on biopesticide formulation, conventional pesticide formulation, and formulation technology platforms. The latter has involved mainly aspects of colloid science and polymer processing, but he has a continuing interest in applying life science and biotechnology derived methods to physical chemistry problems. Dr Fowler was appointed a Syngenta Science and Technology Fellow in 2010, has been awarded the Sandoz and Syngenta science prizes and is an inventor on more than 15 patent families.
13:30 - 14:00 |
Self-assembly of small peptide amphiphiles, the structures formed and their applications
The self assembly of small peptides and other small molecules containing amino acid or peptide residues is receiving considerable attention in the literature because of their potential usefulness in a variety of fields. Potential applications include: substrates for tissue regeneration, controlled release media, and organic electronics to name a few. They are also of interest as novel structurants and surfactants that could replace existing molecules in home and personal care products, or add specific functionality to them. Indeed, possibly the only commercial product currently available is an amino acid based surfactant. The current apparent lack of application of these materials is in stark contrast to the great promise that they offer and has many causes, including material cost, consumer acceptability and regulatory issues. But one issue that is possibly not appreciated is that the very broad range of opportunities offered by small peptide self-assembly is in itself a barrier, in that if one can synthesise a peptide to form a particular structure or function, it is likely that many such molecules exist and that there is no clear means of exploring the structural space thus presented, or of optimising the structure for the material function. In this talk I will give a brief overview of the field and its applications, and discuss the efforts we have been pursuing to improve our understanding of the relationship between structure and function in this class of materials. ![]() Dr William Frith, Unilever, UK
![]() Dr William Frith, Unilever, UKDr Frith is a physical scientist specialising in foods and personal care product technology. He has been working in industrial R&D since 1986, after completing his PhD he joined ICI in Runcorn, UK and spent seven years there. In 1993 Frith joined Unilever R&D at Colworth, and has been here ever since. People call Frith a Rheologist, but he sees himself as more of a general physical scientist, and has experience across a broad range of topics including:
|
|
---|---|---|
14:15 - 14:45 |
Cellulose nano-crystals, rod-like particles for highly stable emulsions
It is now well established that surfactant-free emulsions can be stabilised by solid particles to form the so-called Pickering emulsions for which colloidal particles may be irreversibly anchored at the oil-water interface. They typically require an interfacial solid material that exhibits affinity for the two phases of the emulsion. Rod-like cellulose nanocrystals (CNC) were shown to stabilise highly stable oil-in-water emulsions bending along the interface, and preventing coalescence by steric effect. These CNC provide naturally a large range of particles from 200nm to several microns in length and around 10nm in width leading to variable aspect ratios and presenting a high level of organisation. They form stiff anisotropic versatile platforms with amphiphilic contrasted surfaces that develop hydrogen bonds, electrostatic and van der Waals interactions. Variation of their organisation at the drop interface has been investigated with variation of their surface chemistry. The properties of CNCs make them a good renewable and biocompatible candidate for petroleum derivative alternatives. This presentation aims to illustrate the potential of polysaccharidic nanoparticles at the oil-water interface to be used as building blocks for functional materials as surfactant-free Pickering emulsions, high internal phase emulsions that occur as a gel structure, or foams. ![]() Dr Isabelle Capron, INRA, Nantes, France
![]() Dr Isabelle Capron, INRA, Nantes, FranceDr Isabelle Capron’s research interests concern chemistry and physics of biopolymers. After completing her PhD in Chemistry-Physics of polymers at the University of Rouen (France), she worked as a researcher on various hydrocolloids at the University of Le Mans and at ESPCI in Paris. She spent 3 years in Holland in the starch research center of TNO, the Netherlands Organisation for Applied Scientific Research. Since 2005, she has been a senior researcher at INRA, the French National Institute for Agricultural Research in the field of biopolymers in Nantes (France). Her research in the Biopolymers, Interactions & Assemblies Unit concerns renewable materials, in particular the characterisation of isolated polysaccharides, or in interactions with other biomolecules building complex systems. Her work is now dedicated to colloidal bio-based particles, such as nanocellulose and nanochitin. These rod-like nanocrystals lead to anisotropic particles-stabilised interfaces. She develops such interface design in formulations for end-use property control of colloidal dispersions, Pickering emulsions and foams. |
|
15:30 - 16:00 |
Particles and capsules produced using cross-flow membrane emulsification
A key consideration for many applications that require functional particulates or microcapsules is the accurate control of size and size distribution. Cross-flow membrane emulsification (XME) is an excellent candidate for the production of very tightly size-controlled emulsions, at least in the micron to millimetre droplet size range [1]. Using a latex polymerisation as an example, we show how XME can be used to scale-up a laboratory preparation to multiple litres of product whilst retaining tight control on the final latex. In situ characterisation of the emulsions and resultant latex dispersions using an acoustic backscatter (ABS) system were used to gain insight into the process; the characterisation approach is novel and will be discussed here. Further developing the opportunities for the use of XME, we can also show that particle stabilised emulsions can be used as templates for a range of microcapsules [2]. The use of particulates as stabilisers is more complex than classical surfactants as a result of the widely different adsorption kinetics onto the growing liquid-liquid interface. Conditions necessary for the accurate control of emulsion stability and the efficient uptake of particulates have been examined. Examples of the types of capsules that can be prepared in this way will be discussed. [1] Q. Yuan, R. Hou, N. Aryanti, R. A. Williams, S. Biggs, S. Lawson, H. Silgram, M. Sarkar, R. Birch, Desalination, 224 (2008) 215. ![]() Professor Simon Biggs FREng, The University of Queensland, Australia
![]() Professor Simon Biggs FREng, The University of Queensland, AustraliaSimon Biggs is currently the Executive Dean for the Faculty of Engineering, Architecture and Information Technology at the University of Queensland. He has been the chief investigator on numerous research projects and he is an author of over 200 refereed publications. He received the 2005 Beilby Medal from the RSC/IMMM/SCI awarded for “a substantial contribution towards fundamental research of practical significance”. The main research interests of Professor Biggs’ group are in the field of colloid and interface engineering. He has a strong interest in the measurement, control, and manipulation of inter-particle forces to allow more efficient process engineering of particulate systems. He has pioneered the use of in-situ imaging with direct measurements of both adsorbed amounts and adsorption kinetics, using precision instrumentation such as light scattering, optical reflectometry and atomic force microscopy. More recently his group have begun to develop expertise in the bottom-up manufacture of functional particles, capsules and surface coatings which have application in a range of industrial products and processes. He is the founder of two spin-out companies from the University of Leeds. He was elected as a Fellow of the Royal Academy of Engineering in 2011. The citation for his fellowship states that he is ‘distinguished for achievements in the application of colloid and interface science to the development of new functional materials and innovations in the engineering of particulate systems in the chemical, minerals and nuclear sectors.’ |
|
16:15 - 17:00 |
Summary of discussions and closing remarks
![]() Professor Alex Lips, University of Edinburgh, UK
![]() Professor Alex Lips, University of Edinburgh, UKAlex Lips is currently an Honorary Professor in the Department of Physics and Astronomy at the University of Edinburgh. He also holds a Visiting Scientist position at Princeton University in the Department of Chemical and Bioengineering. He consults for several companies spanning food, chemical, agrochemical and pharmaceutical interests. Alex also is a scientific advisor for EPSRC projects. Prior to his current activities, Alex was for many years one of Unilever's leading physical scientists and he has wide technological experience in Foods and Home and Personal Care. With Unilever, he spent eleven years in the United States where as Director for Skin Materials Science he led a large research group in the materials science of skin care and cleansing products and in the biophysics of skin. Alex has an international reputation in colloid and surface chemistry and published in excess of 120 papers. He also is an inventor of more than 50 independent patent filings across Foods and Home and Personal Care technologies. ![]() Professor Wilson Poon, University of Edinburgh, UK
![]() Professor Wilson Poon, University of Edinburgh, UKWilson Poon has been professor of condensed matter physics at the University of Edinburgh since 1999. He is internationally known for his work using well-characterised model colloids to study fundamental questions in many-body physics, especially the glass transition and gelation, and to understand industrially relevant problems, especially the rheology of suspensions. An EPSRC Senior Research Fellowship and a subsequent ERC Advanced Grant is enabling him to become established in the physics of self-propelled colloids, including motile bacteria. |