Links to external sources may no longer work as intended. The content may not represent the latest thinking in this area or the Society’s current position on the topic.
Particle, condensed matter and quantum physics: links via Maxwell's equations
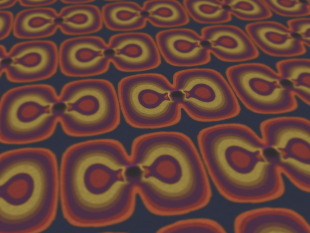
Satellite meeting organised by Professor Anatoly Zayats, Professor John Ellis CBE FRS and Professor Roy Pike FRS
Event details
Maxwell’s equations unify electricity and magnetism and link research in all modern physics. This meeting will provide in‐depth discussions of the state of the art and inter‐relations between the fields of high-energy physics, experimental and theoretical condensed matter and quantum physics so as to explore common themes and analogies between them, exposing young researchers to connections between current developments in these fields.
Abstracts will be made available shortly.
A draft programme is available to download and recorded audio of the presentations will be available on this page after the event.
Attending this event
This is a invitation only residential conference, which allows for increased discussion and networking. It is free to attend, however participants need to cover their accommodation and catering costs if required.
Enquiries: Contact the events team
Participants are also encouraged to attend the related scientific discussion meeting which immediately precedes this event.
Organisers
Schedule
Chair
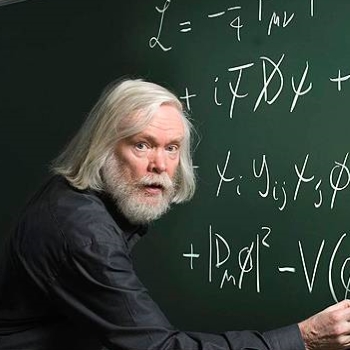
Professor John Ellis CBE FRS, Kings College London, UK
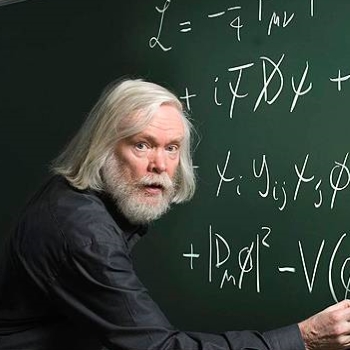
Professor John Ellis CBE FRS, Kings College London, UK
Jonathan Ellis has made numerous contributions to the theory of elementary particles and especially unified gauge theories. Particularly notable were the first proposal of three jet structure (subsequently seen experimentally) as a gluon signal, and the first precise application of the renormalization group to grand unified theories, with the first precise estimate of sin2 θ W. Works on the theory of CP violation, on the phenomenology of the Higgs boson, and on the implications of grand unified theories for the generation of baryon number in the early Universe, have also attracted much attention. His gift for communication (his summer school lectures and reviews are models of their kind), and his special concern with the experimental implications of topical theories, make him a key figure in the discussion of new high-energy physics projects.
09:05 - 09:30 |
Isolated photons, dressed electrons and jets: defining the final state in high energy collisions
At particle colliders, the energy is such that to a good approximation the products of a collision can often be treated as bullet-like particles, and Feynman diagrams treated as probabilities, rather than amplitudes. But clear definitions of observables remain vital to reduce model dependence and achieve the best precision - as well as being desirable in principle. I will discuss some of the issues around this - some of which are resolved, and some of which are the subject of active debate. ![]() Professor Jonathan Butterworth, UCL, UK
![]() Professor Jonathan Butterworth, UCL, UKJon grew up in Manchester and did his degrees at Oxford University. He worked at the DESY laboratory in Hamburg, first employed by Penn State University (1992-1995), then by UCL. Since 2000 he has worked on the ATLAS experiment at the CERN Large Hadron Collider, first helping build it, and then analysing the data, especially on hadronic jets. He is currently head of Physics & Astronomy at UCL. He writes regularly for the Guardian, and recently published a book, Smashing Physics, about his experiences working on the discovery of the Higgs Boson. |
|
---|---|---|
09:30 - 10:00 |
The MOeDAL experiment at LHC: broadening the LHC horizons
In the talk I will review the current physics programme of the MoEDAL experiment at Point 8 of the Large Hadron Collider (LHC) ring at CERN (Switzerland). This experiment, which has resumed full operation recently, after LHC started its second run after the long shut down, is the seventh and newest LHC experiment. The experiment is dedicated to the search for highly ionizing particle avatars of physics beyond the Standard Model, extending signi ficantly the discovery horizon of the LHC. Slowly moving, highly ionising particles that could signal new physics at the LHC-run-II energies of up to 14 TeV, include, among others, long-lived charged supersymmetry partners of ordinary matter that are predicted to exist in supersymmetric extensions of the standard model, new types of quarks, termed ``quirks'', which are bound together in hadronic structures but through a new kind of strong forces, and of course monopole-like magnetically charged particles, of masses in the range 4-7 TeV/c^2 = (7 - 16) x 10^{-24} Kg (termed ``electroweak monopoles'') , that have been predicted to exist in some modifications of the standard model. In view of the nature of this meeting, I will put the emphasis of my review on a brief description of the theory behind such monopole-like configurations, their production mechanisms at LHC energies and the corresponding MoEDAL searches, which are largely complementary to the corresponding programs of the large multipurpose LHC detectors ATLAS and CMS. ![]() Professor Nick Mavromatos, King's College London, UK
![]() Professor Nick Mavromatos, King's College London, UKNick E. Mavromatos is a Professor of Theoretical particle Physics at King's College London, Physics Department, a post he holds since September 2006. He graduated (with a Bachelor degree in Physics (BSc) with First class honours in 1984) from the University of Athens (Greece), and obtained a Doctorate degree (D Phil) in theoretical particle physics from Oxford University (UK) in 1987. He has then been a junior research fellow at Hertford College Oxford University (1987-1990), a research fellow at CERN (Switzerland) (1990-1992), a Marie Curie Fellow at Annecy LAPP (1993-1995) and an STFC Advanced Fellow in Oxford (1995- 1999) before moving as a lecturer to the Physics Department of King's College London (1999) and then (2003-2006) a reader. He is a scientific associate at CERN since 1995. His research interests lie on quantum gravity and string theory phenomenology and astro-particle physics. Twice he has been a recipient of the first Award of the Gravity Research Foundation (USA) Essay competition (1999 and 2005). Since 2011 he is the Physics coordinator of the MoEDAL/LHC Experiment at CERN. He is the author of more than 300 research publications and refereed conference proceedings. He is a Fellow of the Institute of Physics (UK) (2004) and an elected ordinary member of the London Physical Society (2003). He has also been several times a visiting professor in the University of Valencia (Spain) where he taught graduate courses on General Relativity and Astroparticle Physics. |
|
11:00 - 11:30 |
Maxwell in one equation, at home in space-time
The subject of this talk is the link between Maxwell's equations and Clifford Algebra. The latter entered mainstream physics via the Pauli and Dirac algebras in the 1920s, but it is less commonly known that the Maxwell equations themselves can benefit from being written in a Clifford Algebra form. The simplest form of the equations is achieved in a more recent version of Clifford Algebra called Geometric Algebra (GA), which was pioneered by David Hestenes in the 1960s. GA provides a unified mathematical language spanning complex numbers, quaternions, spinors, and the tensors of general relativity, and providing for each a home in the geometric algebra of spacetime. Applied to the Maxwell equations, it allows them to be written in a single compact equation, and in a form which displays clearly its relation with other wave equations of relativistic physics, such as the neutrino and Dirac equations. The benefits of the new form are not just cosmetic, but suggest new solution approaches, and the example of radiation from a moving charge will be discussed, where the GA approach provides one of the most compact (and intuitively useful) expressions for the radiated fields so far found. ![]() Professor Anthony Lasenby, University of Cambridge, UK
![]() Professor Anthony Lasenby, University of Cambridge, UKAnthony Lasenby is Professor of Astrophysics and Cosmology at the University of Cambridge. After undergraduate and postgraduate work at Cambridge and Queen Mary College, his Ph.D. work was carried out at the Jodrell Bank Radio Observatory specialising in the Cosmic Microwave Background, which has been a major subject of his research ever since. After a brief period at the National Radio Astronomy Observatory in America, he moved from Manchester to Cambridge in 1984, and has been at the Cavendish Laboratory Cambridge since then. He was Head of the Astrophysics Group and the Mullard Radio Astronomy Observatory in the Cavendish Laboratory from 2001-2007, a Deputy Head of the Laboratory 2003-2005, and is currently Deputy Head of the Kavli Institute for Cosmology in Cambridge. A recent major project in which he has been working is the Planck Satellite project, for which he is a Co-Investigator and member of the Planck Editorial Board. His other main interests include theoretical physics and cosmology, statistical techniques in data analysis and the application of new geometric techniques in physics. |
|
11:30 - 11:45 |
Finite-energy electroweak monopoles at the LHC
Monopole configurations in the Standard Model have infinite energy and are therefore not physical. One possibility to obtain a finite mass monopole is to embed the Standard Model SU(2)xU(1) into a semi-simple group. Alternatively the Standard Model may be modified by a non-minimal Higgs coupling whose form is chosen to regularize the infinite energy. A finite-energy electroweak monopole compatible with Higgs measurements is constructed. ![]() Dr Tevong You, University of Cambridge, UK
![]() Dr Tevong You, University of Cambridge, UKTevong You studied at Imperial College London and ETH Zurich before obtaining his PhD at King’s College London under Professor John Ellis FRS. He is currently a Junior Research Fellow at Gonville & Caius College, Cambridge. His research interests in theoretical particle physics lies in the phenomenological connection between abstract mathematical structures and concrete experimental results. |
|
11:45 - 12:00 |
Magnetic current and new modifications of Maxwell's equations
Maxwell’s equations are the main foundation of current communication technology; however, given certain ambiguities and unknown parameters, they are still incomplete. Magnetic current is apparently the main crucial missing component of these equations. In this talk, we will resolve to revise these equations as well as the conventional definitions of the terms and parameters from the beginning, basing ourselves merely on logical theory to justify all measurements made so far. These revisions will be initiated by modifying Bohr’s Model and the physical differentiations of magnetic and electrical charges and fluxes in order to justify all electromagnetic phenomena under a consistent umbrella. Consequently, we can theoretically present a rational illustration of magnetic current and amend the contradictions and inconsistencies in the current models and theory of electromagnetic waves. Furthermore, a question is put forward to determine whether we can go beyond Maxwell’s equations. In order to answer this question some rational justifications, confirmed with measurements, will be presented which demonstrate that we can propagate the wave inside a metallic waveguide merely by using a magnetic field without generating an electrical field. Finally, a general question is put forward asking the gravity can be linked to electromagnetic forces or not. Criticizing the Standard Model and String theory, a different hypothetical model is presented to justify the gravity based on the proposal theory. ![]() Mr Mehdi Nosrati, University of Alberta, Canada
![]() Mr Mehdi Nosrati, University of Alberta, CanadaMehdi Nosrati received the MS degree in electrical engineering from Tarbiat Modares University, Tehran, Iran, in 2006. He was lecturer for 4 years in the university. He started his PhD program from Sept. 2011 in the University of Alberta. His current research interest includes microwave and millimeter-wave components. Integrating MEMS actuator with waveguide structures to design tunable microwave components is the main part of his research as the PhD student. Moreover, his personal research interests include the nature of electromagnetic waves and Maxwell’s equations. |
|
12:30 - 13:00 |
Higgs Physics at the Run2 LHC
A way to search for new physics is to look for deviations in Higgs and electroweak production. This talk explains how to do this in detail in the context of an Effective Theory approach. It will be shown how a global fit of LEP and LHC Run1 data has constrained new physics participating in the electroweak sector to a level that is competitive and complementary to direct searches for new states and move onto the prospects for discovery at Run2 LHC. ![]() Professor Veronica Sanz, University of Sussex, UK
![]() Professor Veronica Sanz, University of Sussex, UKBorn in Spain, Veronica Sanz held postdoctoral positions at Durham and Boston. Sanz was a visiting scholar at Harvard and got a Marie Curie at Yale. She obtained a professorship at York (Canada) in 2008, was an Associate at CERN in 2012 and moved to the UK in 2013. |
Chair
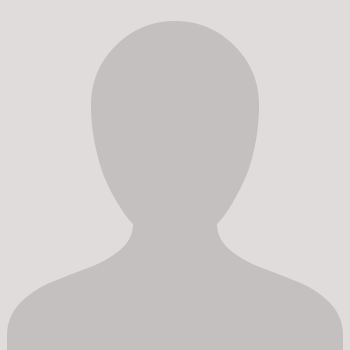
Dr Joe Bhaseen, King’s College London, UK
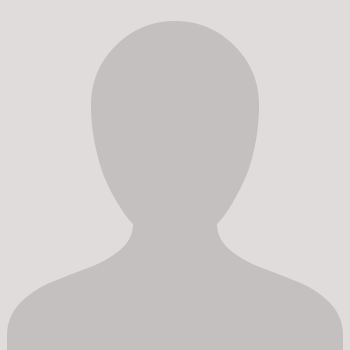
Dr Joe Bhaseen, King’s College London, UK
Joe Bhaseen has been a lecturer in the Department of Physics at King's College London since 2012. His research in theoretical condensed matter physics is in the area of strongly correlated systems. His research interests include field theory approaches to quantum many body systems, cold atomic gases and matter-light systems. He is currently working on the dynamics of strongly correlated systems out of equilibrium, including condensed matter applications of gauge-gravity duality.
14:15 - 14:30 |
Emergent coulomb physics in Heisenberg spin liquids
Condensed matter systems exhibit a richness of possible ground states, with some carrying excitations with unique properties otherwise not shared by the elementary particles in nature. A particular class of geometrically frustrated spin models is known to exhibit excitations that much alike mimics the behavior of Coulomb charges. At low temperatures these systems are described by an emergent gauge field, with spin configurations mapped to configurations statistically described by a field governed by a magnetostatic action. These so-called classical Coulomb spin liquids were up to now known to exist only in models whose concomintant gauge-charged excitations live on a bipartite lattice. The situation where the charges occupy the sites of a non-bipartite lattice was hitherto unknown, and in this talk a model with emergent charges on a triangular lattice will be presented. This has interesting new consequences for observable physical phenomena, and goes along with a novel mapping to a gauge field. Dilution of this system produces disorder nucleated 'vector' charges that are fractionalized objects carrying 1/3 of the moment of the original spins in the model, making this perhaps the first instance of a classical spin system exhibiting fractionalization into 3 objects. A zero temperature effective theory for these fractionalized, Coulomb vector charges, will be presented, which represents a new kind of Coulomb spin model presenting a glassy phase. ![]() Dr Jorge Rehn, Max Planck Institute for the Physics of Complex Systems, Germany
![]() Dr Jorge Rehn, Max Planck Institute for the Physics of Complex Systems, GermanyJorge Rehn received his BSc and MSc in Physics at Universidade Federal de Pernambuco, Recife-Brasil. He is currently a PhD student at the MPIPKS, Dresden, and his main research interest lies on the non-trivial effects of disorder in various physical systems, as for example in the emergence of a many-body localized phase on quantum spin chains, or what new phenomena are expected by introduction of disorder in the emerging topological phase of highly frustrated magnets. |
|
---|---|---|
14:30 - 14:45 |
A study of the effect of curvature on near-field radiative heat transfer
Electromagnetic wave-effects become important in radiative heat exchange between two bodies when they are separated by gaps less than wavelength of light. When the two bodies support surface modes which can be thermally excited, the enhanced near-field radiative transfer due to photon tunnelling can exceed the classical blackbody limit by several orders of magnitude. While the dependence of near-field radiative transfer on the gap between two planar objects is well understood, the effect of curvature on near-field radiative transfer is unclear. In particular, the relevance of an approximate method (called proximity approximation) to predict the near-field interaction between curved bodies is disputed. Hence, the exact computation of near-field radiative transfer between curved bodies, such as between two spherical bodies, become important. This computation is based on a first-principles derivation using vector spherical wave expansion method which is commonly employed to analyse electromagnetic scattering problems in spherical coordinates. In this talk, drawbacks in current methods to compute the radiative transfer between the spherical bodies are discussed, and a simplified form of the coefficients of vector translation theorem is shown which enables computation of radiative heat transfer for nanoscale gaps. Based on the analysis of results, a modified form of proximity approximation method is also proposed to resolve the discrepancy between theoretical predictions and experimental observations. ![]() Dr Karthik Sasihithlu, Imperial College London, UK
![]() Dr Karthik Sasihithlu, Imperial College London, UKDr. Karthik Sasihithlu is a Research Associate at Blackett Laboratory, Imperial College London working under Professor Sir John Pendry exploring different mechanisms of near-field heat transfer. He completed his Ph.D. at Columbia University, New York, where he analyzed curvature effects in near-field radiative heat transfer. Before starting his position at Imperial College, he was a postdoctoral researcher at Institut d’Optique, France, where he worked under Professor Jean-Jacques Greffet to model scattering from a layer of particles placed close to a substrate. |
|
14:45 - 15:00 |
Graphene, plasmons and transformation optics
The optical, and consequently, plasmonic properties of graphene (an atomic-thick honeycomb lattice of carbon atoms) can be controlled by external means such as a gate voltage, and for this reason graphene has attracted a lot of attention as a highly versatile plasmonic material. Plasmons in graphene feature a deep subwavelength confinement together with a strong enhancement of the electromagnetic fields, which can be employed to increase the low optical absorption of this 2D material having a great potentiality for graphene-based optoelectronics. In this contribution Dr Huidobro uses transformation optics to study plasmons in graphene excited with the help of subwavelength dielectric gratings. The potential of transformation optics, which has successfully provided analytical solutions to various problems in plasmonics, resides in its ability to relate highly symmetric structures to more complex ones. Here they consider gratings formed by a periodic modulation of graphene’s conductivity, as well as this together with a subwavelength dielectric grating of the same periodicity placed close to the graphene sheet. In both cases, the shape of the periodic profiles derive from a conformal transformation that maps a Cartesian mesh into a wavy and periodic one, and they can be accurately approximated to a sinusoidal shape. It shows coupling to highly confined graphene surface plasmons that provide very large field enhancements, thus increasing the low optical absorption of graphene. For the case of periodic modulation of the conductivity they discuss the optimal conditions for coupling into the surface plasmons. ![]() Dr Paloma Huidobro, Imperial College London, UK
![]() Dr Paloma Huidobro, Imperial College London, UKDr Huidobro is a research associate in the nanoplasmonics group at the Department of Physics, Imperial College London, where she works in collaboration with Prof. Stefan Maier and Prof. Sir John Pendry. She graduated and received her PhD in Condensed Matter Physics and Nanotechnology from Autonomous University in Madrid. Under the supervision Prof. García-Vidal she presented a PhD thesis that included the topics of spoof surface plasmons, transformation optics, quantum plasmonics and nano-optical trapping. Her current research interests are in the area of nano-photonics, plasmonics, metamaterials and transformation optics. She develops theoretical research in this field, and holds expertise in both analytical techniques and simulation tools for solving Maxwell’s equations. |
|
16:00 - 16:30 |
From Einstein-Maxwell to quantum transport
Recently there has been a great deal of interest in the possible applications of gauge-gravity duality to condensed matter problems. This talk will discuss applications of these holographic techniques to strongly correlated systems. The methods provide unifying links between different domains of physics, including gauge theories, gravity, fluid dynamics and quantum mechanics. ![]() Dr Joe Bhaseen, King’s College London, UK
![]() Dr Joe Bhaseen, King’s College London, UKJoe Bhaseen has been a lecturer in the Department of Physics at King's College London since 2012. His research in theoretical condensed matter physics is in the area of strongly correlated systems. His research interests include field theory approaches to quantum many body systems, cold atomic gases and matter-light systems. He is currently working on the dynamics of strongly correlated systems out of equilibrium, including condensed matter applications of gauge-gravity duality. |
|
16:45 - 17:15 |
The history of Maxwell’s equations
Maxwell’s equations have been called the Mona Lisa of physics. Simple, elegant and powerful, they encapsulate the laws of electromagnetism, which, 150 years ago, opened the door to hitherto undreamt of regions of scientific knowledge. As Albert Einstein put it, these laws marked the end of one scientific epoch and the start of another (our own). Richard Feynman was in no doubt that future historians will judge Maxwell’s discovery to be the most significant event of the nineteenth century. Basil Mahon will show that the emergence of these wonderful equations owes as much to the experimental genius of Michael Faraday as to the theoretical genius of Maxwell. By creating the entirely new concept of the electromagnetic field, the two men made it possible for scientists to break free from the strictly mechanical view of the physical world which had prevailed since Newton’s time. Maxwell’s theory was so different from anything that had gone before that none of his contemporaries really understood it, and several decades passed before the theory gained acceptance. Basil Mahon will discuss the factors that brought this acceptance about, including the contribution of Oliver Heaviside. ![]() Basil Mahon
![]() Basil MahonBasil Mahon has had careers as an officer in the Royal Electrical and Mechanical Engineers, and in the Government Statistical Service, where one of his jobs was to run the 1991 census in England and Wales. He has since followed up a lifelong interest in the physical sciences and their history by writing books and articles. His book ‘The Man Who Changed Everything: the Life of James Clerk Maxwell’ was published in 2003. ‘Oliver Heaviside: Maverick Mastermind of Electricity’ followed in 2009. His latest book, co-written with Nancy Forbes, is ‘Faraday, Maxwell, and the Electromagnetic Field: How Two Men Revolutionized Physics’, published in 2014. |
Chair
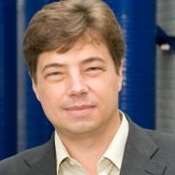
Professor Anatoly Zayats, King's College London, UK
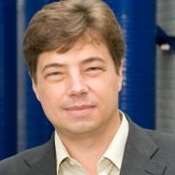
Professor Anatoly Zayats, King's College London, UK
Professor Anatoly V. Zayats is the head of the Experimental Biophysics and Nanotechnology Group at the Department of Physics, King’s College London, where he also leads Nano-optics and Near-field Spectroscopy Laboratory. He graduated and received PhD in Physics from Moscow Institute of Physics and Technology. His current research interests are in the areas of nano-optics, scanning probe microscopy, nanophotonics and plasmonics, metamaterials, nonlinear optics and spectroscopy, surface plasmons and polaritons, and optical properties of surfaces, thin films, semiconductors and low-dimensional structures. He is a holder of the Royal Society Wolfson Research Merit Award, a Fellow of the Institute of Physics, the Optical Society of America, SPIE and the Royal Society of Chemistry.
09:00 - 09:30 |
Electromagnetic doughnuts: localised and propagating toroidal excitations
Recent progress in toroidal electrodynamics that has been possible with artificial metamaterials will be reviewed. The toroidal dipole is a localised electromagnetic excitation independent from the familiar magnetic and electric dipoles. While the electric dipole can be understood as separated opposite charges and the magnetic dipole as a current loop, the toroidal dipole introduced by Y. B. Zaldovich in 1958 it corresponds to currents flowing on the surface of a torus. Resonant interactions of induced toroidal dipoles with electromagnetic waves have recently been observed in metamaterial structures at microwave, terahertz and optical frequencies. They provide distinct and physically significant contributions to the basic characteristics of matter including absorption, dispersion, and optical activity, the origin of which cannot be comprehensively interpreted in the context of standard multipoles alone. Interference of radiating induced toroidal and electric dipoles leads to transparency windows in artificial materials as a manifestation of the dynamic anapole. Toroidal excitations also exist in free-space as spatially and temporally localised electromagnetic pulses propagating at the speed of light and interacting with matter. ![]() Professor Nikolay Zheludev, University of Southampton, UK
![]() Professor Nikolay Zheludev, University of Southampton, UKProfessor Nikolay Zheludev’s research interests are in nanophotonics and metamaterials. He directs the Centre for Photonic Metamaterials at Southampton University, UK and Centre for Disruptive Photonic Technologies at Nanyang Technological University, Singapore. He is also founding co-Director of the Photonics Institute at NTU, Singapore and Deputy Director of the Optoelectronics Research Centre at Southampton. His personal awards include the Royal Society Wolfson Merit Award and Senior Research Professorships of the EPSRC and the Leverhulme Trust. In 2015 he was awarded the IOP Thomas Young medal “For global leadership and pioneering, seminal work in optical metamaterials and nanophotonics”. Prof Zheludev is Editor-in-Chief of Journal of Optics. |
|
---|---|---|
09:30 - 09:45 |
Near-field terahertz spectroscopy: studying terahertz resonators on a micro-scale
Terahertz radiation allows for non-destructive detection of objects and processes ’invisible’ at optical and microwave frequencies. Modern terahertz science promises break-through security, medical, and quality control techniques, as well as access to crucial astronomical observation and environmental monitoring. However, the emerging terahertz technology is held back by the scarcity of functional materials and devices required for manipulation of terahertz radiation. This talk demonstrates opportunities and advantages of the near-field terahertz time-domain spectroscopy for direct studies of terahertz electromagnetic resonances occurring on a micrometre scale. As examples of micro-resonators, it considers conductive micro-fibres and dielectric micro-spheres. Micro-resonators are at the heart of numerous promising terahertz solutions, including the metamaterial approach – creating functional materials from artificial pre-designed resonant micrometre-sized ‘meta-atoms’. Experimental studies of micrometre-scale terahertz resonances are essential, yet inaccessible to common far-field spectroscopic techniques due to extreme sensitivity requirements. This non-contact technique maps the field patterns of terahertz resonant modes excited in individual conductive or insulating micro-objects, and gives access to essential parameters of micro-resonators, including their resonance frequency, local field enhancement and quality factors. Depending on the underlying physics of observed terahertz resonances, it allows for material and structural characterisation of micro-objects. This work uses the examples of carbon micro-fibres and titanium dioxide micro-spheres to show the advantages of near-field terahertz time-domain spectroscopy for non-contact terahertz conductivity probing and anisotropic material characterisation; and direct observation of versatile resonant modes, including surface-plasmon resonances in conductive dipoles, and magnetic dipole resonances in dielectric subwavelength terahertz resonators. ![]() Dr Irinia Khromova, King's College London, UK
![]() Dr Irinia Khromova, King's College London, UKDr Irina Khromova received a Ph.D. degree in physical and mathematical sciences from Saratov State University, Russia, in 2008, and a Ph.D. degree in telecommunications engineering from the Public University of Navarra, Spain, in 2011. She began her research career at the Nonlinear Phenomena Group, Saratov State University in 2003. In 2005, she joined the Chair of Laser and Computer Physics, Saratov State University. In 2007, she joined the Antenna Group, Public University of Navarra. She is currently an Assistant Professor at the Public University of Navarra, and a Research Associate at King’s College London, UK. She was a Visiting Researcher at the Macquarie University, Australia (2009), the Technical University of Eindhoven, the Netherlands (2010), Saratov State Technical University, Russia (2011), Technical University of Denmark (2013) and University College London (2014-2015). Her current scientific interests include terahertz near-field spectroscopy and microscopy, properties and applications of carbon nanostructures, all-dielectric and plasmonic terahertz and optical metamaterials. |
|
09:45 - 10:00 |
Transformation optics applied to electron-loss problems in plasmonics
Transformation optics is a relatively new subfield in electromagnetic research. Yet, it has been at the heart of many of the most promising advancements in electromagnetism in recent years. Originally developed to aid numerical simulations in cylindrical geometries, it has since been applied as a design tool for such exotic devices as invisibility cloaks or negative refractive index lenses. Within the last 5 years, transformation optics has entered the field of plasmonics. It has proved valuable as a design tool for devices such as beam shifters, surface cloaks or light harvesters. Moreover, it has also proven itself as an analytical tool in the study of interacting plasmonic nano-particles or van der Waals forces. This talk would like to add an entry to the already long list of fields where transformation optics can make a difference. The study of electron energy loss spectroscopy of plasmonic nano-particles. ![]() Mr Matthias Kraft, Imperial College London, UK
![]() Mr Matthias Kraft, Imperial College London, UKMatthias Kraft received his undergraduate degree in Physics from Imperial College London in 2013. His studies focussed on theoretical physics and I completed his masters project on noise assisted transport in ultracold atoms. Since then he has joined John Pendry's research group and is currently pursuing a PhD in the field of Transformation optics and Plasmonics. |
|
11:00 - 11:30 |
From spinning fields to chiral optical forces
Direct manipulation of particles through light-induced forces has led to formidable progress, which has been impacting research in many areas ranging from ultracold-matter physics to biology. The rise of nano-optics has offered the experimentalists new types of optical excitations associated with inhomogeneous fields and complex beam topologies that lead to a great variety of dynamical effects. It was pointed out recently that light radiation pressure is determined by the sole orbital part of the Poynting vector, with no contribution from its spin part. This has important consequences that can be clearly illustrated in the context of surface plasmon optics. The intrinsic spinning character of a plasmonic field brings indeed a clear dynamical distinction between orbital and spin energy flows that can be readily discussed in terms of induced optical forces and torques. Simultaneously, the interest focuses on exploiting the connections between spin-orbit interaction and the concept of chirality at the level of the plasmonic near field. These connections have direct fundamental implications with new possibilities opened in the context of chirality. In particular, new types of optical forces have been recently unveiled when a chiral object is illuminated by a chiral light field that can lead to new chiral separation and discrimination schemes. Such new effects and schemes will be presented and discussed when aiming at manipulating chiral nano-objects using tailored chiral optical fields. ![]() Dr Cyriaque Genet, ISIS - University of Strasbourg and CNRS, France
![]() Dr Cyriaque Genet, ISIS - University of Strasbourg and CNRS, FranceCyriaque Genet received his PhD in 2002 from University Pierre-et-Marie-Curie in Paris. His PhD focused on quantum vacuum fluctuations and Casimir forces. He then worked as a postdoctoral researcher in the group of Prof Woerdman at the University of Leiden, Netherlands. In 2004, he joined the group of Prof. Ebbesen in Strasbourg as a permanent CNRS researcher. His research interests are in the areas of surface plasmon optics, strongly coupled systems, optical trapping and plasmonic forces. His most recent work focused on spin-orbit interactions in nano-optics. In this context, he is currently studying the importance of chirality in the field of optical forces where new types of chiroptical discrimination protocols, pulling forces and left-handed torques can be described both from far and near-field perspectives. |
|
11:30 - 11:45 |
Spin Hall effects in photonics
Scalar waves can be uniquely described by their amplitude and phase distributions through space; while the amplitude determines the position, the phase distribution determines the propagation direction (wave-vector) of the waves. In addition to those spatial degrees of freedom, electromagnetic waves, being described by vector fields, also posses polarization degrees of freedom, determined by the directions in which the electric and magnetic fields oscillate with time. Spin-orbit interactions of light describe how, under certain circumstances, the polarization of light can affect its propagation direction. This coupling between the spatial and polarization degrees of freedom is completely described by Maxwell's equations applied to the appropriate geometry, and can be analogous to spin-orbit interactions of relativistic quantum particles and electrons in solids. After mentioning some general examples, this talk will focus on a novel kind of spin-orbit interaction that arises on near fields, which are associated with an elliptical or circular polarization in the electric and/or magnetic fields, and can be exploited for an extremely robust polarization-controlled nano-routing of electromagnetic waves in a wide range of scenarios. Experimental examples in different platforms will be described, as well as the reciprocal scenario, in which light propagation can be used to synthesize polarizations. ![]() Dr Francisco Rodríguez-Fortuño, King's College London, UK
![]() Dr Francisco Rodríguez-Fortuño, King's College London, UKFrancisco José Rodríguez-Fortuño is a researcher at King's College London on the topics of plasmonic devices, nano-optics, optical forces, metamaterials and novel electromagnetic phenomena. After obtaining a degree in Telecommunications Engineering in 2008 at the Universitat Politècnica de València (UPV), Spain, he carried out his Masters' and PhD studies at UPV in the topic of plasmonic metamaterials. During his PhD, Francisco was a visiting researcher at the University of Pennsylvania, USA, 2011 and at King's College London, UK, 2012, where he later worked as a Research Assistant in 2014. Francisco has authored or co-authored 25 research papers, contributed 38 works at international conferences and authored a popular article in the spanish edition of Scientific American. He has received several academic performance distinctions and two prizes for his PhD work. Francisco is a member of the editorial board of Scientific Reports, Nature Publishing Group. |
|
11:45 - 12:00 |
Multipoles, spherical t-designs and polarization state reconstruction
Measurement of the polarisation state of light is a common problem in many branches of fundamental and applied science. Accurate and robust measurements are essential in all such applications. Recently, interest has grown in determining higher order polarisation properties, as these can play a key role in nonlinear and quantum processes. Whilst polarisation dependent optical nonlinear processes can provide important insights into crystal and molecular structure, higher order properties described through a multipolar expansion of the polarisation matrix can contain “hidden” polarisation correlations, which are of interest both in a quantum and in a classical context. Optimisation of linear polarisation measurements is well studied, however, the problem of optimally reconstructing higher order polarisation properties has to date remained unsolved. The authors present their recent work in which they derive an analytic solution to this problem using an arbitrary number of measurements. Their analysis hence generalises existing results in the linear domain which have been predominately confined to minimal measurement sets, however, critically the authors present optimal measurement strategies for higher order problems. The presented method employs the elegant mathematical framework of spherical t-designs, thereby the derived optimal measurement sets constitute a powerful generalisation of the concept of mutually unbiased bases. ![]() Dr Matthew Foreman, Max Planck Institute for the Science of Light, Germany
![]() Dr Matthew Foreman, Max Planck Institute for the Science of Light, GermanyMatthew R. Foreman received his Ph.D. in Physics from Imperial College London, UK, in 2010. Thereafter he was awarded an Engineering and Physical Sciences Research Council (EPSRC) Ph.D. Plus fellowship for the continuation of his work on single molecule polarization microscopy. In 2010 he also held a visiting lecturer position at the National Yang Ming University in Taiwan working within the Modern Optics Laboratory. Subsequently, he was based at the UK National Physical Laboratory within the Engineering Measurement Department, where his work focused on the calibration and correction of optical instruments in surface metrology. In 2012 he took up a Max Planck Postdoctoral Research position at the Max Planck Institute for the Science of Light in Erlangen, Germany, before being awarded an Alexander von Humboldt Research Fellowship in 2013 for his work on hybrid plasmonic–photonic whispering gallery mode sensors. |
Chair
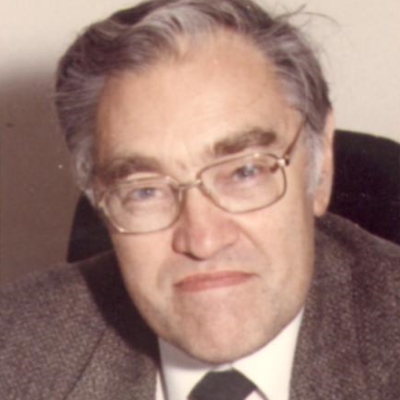
Professor Roy Pike FRS, King's College London, UK
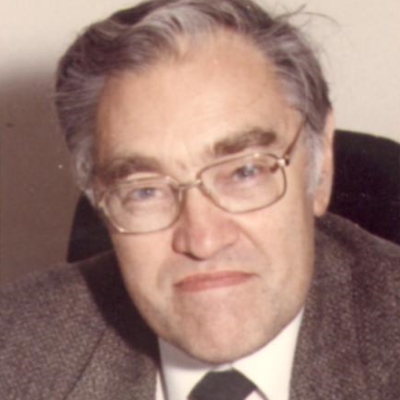
Professor Roy Pike FRS, King's College London, UK
Professor Pike has first degrees in both Mathematics and Physics and a PhD in X-ray diffraction from University College Cardiff, where he is now a College Fellow. He won a Fulbright Scholarship to the Physics Faculty, MIT, returning to the UK Royal Radar Establishment where he rose to Chief Scientific Officer with a visiting Professorship of Mathematics at Imperial College, London. He was then appointed Clerk Maxwell Professor of Theoretical Physics at King’s College, London, also Head of School of Physical Sciences and Engineering and a College Fellow.
His main research fields are theoretical physics, quantum optics and the burgeoning mathematical discipline of inverse problems, founding its first Journal. Awards include Royal Society Charles Parsons Prize, McRobert Award of the Confederation of Engineering Institutions, Annual Achievement Award of the Worshipful Company of Scientific Instrument Makers, Civil Service Award to Inventors and Guthrie Medal and Prize of the Institute of Physics.
13:30 - 14:00 |
Can we make a spin qubit activated switch for lossless quantum photonic circuits?
Lossless switches for single photons are a key element in photonic quantum circuits for quantum information processing and quantum simulations. One requires that, dependent on the state of one quantum bit (qubit), one may switch the logic state of another qubit. Essentially, this would require the state of one photon to switch another photon (for example from horizontal to vertical polarisation). For photons, this process is impossible to achieve deterministically (i.e. predictably without loss) without a very strongly non-linear material. Dr Oulton will discuss that this strong non-linearity may come from the interaction with a single quantum emitter. She will outline how a trapped electron spin in a quantum dot, a nanosized region of semiconductor, may be used to achieve this strong non-linearity. Dr Oulton will then go on to discuss how photonic structures surrounding the quantum dot may be used to increase the interaction strength, discussing previous approaches using high quality factor optical cavities. Before explaining that these high quality factor structures, which are difficult to manufacture, are perhaps not necessary and that present, easily fabricated structures may be used, bringing the lossless single photon switch closer to reality. ![]() Professor Ruth Oulton, University of Bristol, UK
![]() Professor Ruth Oulton, University of Bristol, UKRuth Oulton’s field of research involves the study of nanoscale semiconductor devices that enable the exchange of “quantum” information between a single electron and a single photon. The idea is that they can use the rules of quantum mechanics to perform computing and measurements in a completely new way. She take ideas from quantum theory and information science, bring them together with what we are beginning to understand about semiconductors on the nanoscale, and to make working quantum devices that engineers will use as part of their everyday toolkit. In her recent work she studies single electron spins in atomic-like systems, and studies how the angular momentum of the photon and spin exchange information, and how photonic design can influence this. In other interdisciplinary side projects she studies the role of photonic structures in plants such as seaweed and begonias. |
|
---|---|---|
14:00 - 14:15 |
Quantum information processing with photons in dielectric circuits
Scalable quantum technologies require faithful conversion between matter qubits storing the quantum information and photonic qubits carrying the information in integrated circuits and waveguides. In this talk I show how photons generated by quantum dots in waveguides can be manipulated to create a variety of resources necessary for scalable quantum technologies. ![]() Dr Pieter Kok, University of Sheffield, UK
![]() Dr Pieter Kok, University of Sheffield, UKPieter Kok studied physics at the university of Utrecht in the Netherlands, and gained his PhD in quantum optics and quantum information from the university of Wales in Bangor in 2001. He worked as a postdoctoral researcher at NASA’s Jet Propulsion Laboratory in Pasadena, at Hewlett-Packard Laboratories in Bristol, and at the university of Oxford. He is currently a Senior Lecturer at the university of Sheffield, studying the creation of entanglement between photons and quantum dots for the purposes of quantum computing, quantum imaging and quantum precision metrology. |
|
14:15 - 14:30 |
Light-matter interactions for scalable quantum photonics
The high frequency of optical signals makes them ideal carriers of classical and quantum information, capable of supporting large alphabets and high data rates over long distances, with zero thermal noise even at room temperature. It has long been known that optics could provide a route to large scale quantum information processing in ambient conditions. But despite advances in waveguide and detector technology, large scale quantum photonics remains impossible because logic operations and quantum correlations can only be generated probabilistically. Here I will describe how coherently driven media can be used to modify propagating light fields to overcome this scalability challenge. ![]() Dr Josh Nunn, University of Oxford, UK
![]() Dr Josh Nunn, University of Oxford, UKAfter his DPhil on 'Quantum memories' at Oxford, Josh Nunn took up a Departmental Lectureship there, then a brief postdoctoral research fellowship at the NRC in Canada, before returning to Oxford as a Royal Society URF, where his research focusses on quantum light-matter interactions as an enabling technology for quantum information processing. In particular he is developing quantum memories for storing light in vapours and crystals, along with strong optical non-linearities and coherent conversion between the optical and microwave domains. |
|
15:30 - 16:00 |
Photonic contributions to quantum technology
Quantum Physics has focused on light, matter and their interaction, from the early days of quantum mechanics right down to the present day. Much of this work has concentrated on the nature of quantum correlations beyond what is allowed classically. Although understanding the duality of wave and particle challenges our classical intuition, one cannot escape asking hard questions on this issue, and indeed this subject was at the heart of Bohr-Einstein debate in the early years of the 20th century. The emergence of quantum optics and especially studies of the nature of nonclassical light and its exploitation in quantum computing and quantum cryptography have put this back at the heart of current physics Progress in identifying, generating and characterizing nonclassical states has been spectacular. Quantum Information Science in part has grown out of this progress: the quantum world allows information to be encoded, manipulated and transmitted in ways quite different from classical physics. This paper will discuss the formation, propagation and manipulation of single photon wavepackets, explain how these can be used in simple quantum networks (for example in quantum walks and in Boson Sampling), and describe recent work on detecting single photons non-destructively. ![]() Sir Peter Knight FRS, Imperial College London, UK
![]() Sir Peter Knight FRS, Imperial College London, UKPeter Knight is Senior Research Investigator at Imperial College. He retired in 2010 as Deputy Rector (Research) at Imperial. He was knighted in 2005 for his work in optical physics. Knight was the 2004 President of the Optical Society of America and 2011-2013 President of the Institute of Physics. He is Editor of Contemporary Physics, Chair of the UK National Quantum Technology Programme Strategy Advisory Board, chairs the Quantum Metrology Institute at the National Physical Laboratory, was until 2010 chair of the UK Defence Scientific Advisory Council and remains a UK Government science advisor. His research centres on quantum optics and quantum technology. He has won the Thomas Young Medal and the Glazebrook Medal of the Institute of Physics, the Ives Medal and the Walther Medal and Prize of the OSA, the Royal Medal of the Royal Society and the Faraday Prize of the IET. |
|
16:15 - 17:00 | Panel discussion |