Links to external sources may no longer work as intended. The content may not represent the latest thinking in this area or the Society’s current position on the topic.
Faulting, friction and weakening: from slow to fast motion
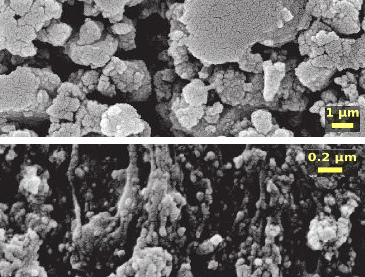
Theo Murphy international scientific discussion meeting organised by Dr Stefan Nielsen, Dr Tom Mitchell, Dr Alexandre Schubnel and Professor James R. Rice ForMemRS
Event details
Faults accommodate momentous deformations, from earthquakes lasting seconds to silent creep lasting millions of years. Versatile processes of fluid flow, friction and rheology develop at all scales. Recent advances blur the frontier between brittle and plastic, fast and slow strain behaviours in ceramics and faults. These will be explored by confronting different disciplines, from material sciences to geology, seismology, experiments and modelling.
Attending this event
This is a residential conference, which allows for increased discussion and networking. It is free to attend, however, participants need to cover their accommodation and catering costs if required.
For any enquiries please contact the events team.
Organisers
Schedule
Chair
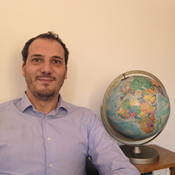
Dr Alexandre Schubnel, Ecole Normale Supérieure, France
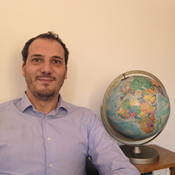
Dr Alexandre Schubnel, Ecole Normale Supérieure, France
Alexandre Schubnel has a double appointment as an associate researcher at the Centre National de la Recherche Scientifique, and as an associate professor at the laboratoire de Géologie of Ecole Normale Supérieure in Paris, France. His research interests lie in Rock Physics and Rock Mechanics. His major contribution is the development of laboratory seismology. Indeed, fracture experiments performed on rocks at in-situ conditions demonstrated that accelerations recorded in the kilohertz range on centimeter-sized samples were self-similar to the ones one can expect at the kilometric scale during a large earthquake, and as such, were successful at reproducing supershear ruptures on laboratory rock samples for the first time. Contemporaneously, AS and colleagues performed experiments at Earth’s mantle conditions, which successfully reproduced deep-focus earthquakes in the laboratory. Put together, these two studies demonstrate that dynamic rupture propagation is self-similar, and thus, laboratory earthquakes are not simple earthquake analogs, but real -yet tiny earthquakes. As such, his work has opened the door to “laboratory seismology”, i.e. the possibility of reproducing earthquakes and studying their source complexity, in the laboratory.
AS was the recipient in 2014 of both the bronze medal of CNRS and the Gouilloud-Schlumberger (young researcher prize in Earth Sciences) of the French National Academy of Sciences.
09:00 - 09:45 |
Subduction zone earthquakes: A case study on the application of lab data to understand earthquake processes
Earthquakes within the oceanic lithosphere, and the slab-wedge interface, occur over an exceptionally wide range of conditions. Seismicity is observed at normal stresses ranging from ~200 to ~8000 MPa (even greater for deep focus events), temperatures from less than 100oC up to at least 600oC and under both dry and water saturated conditions. The range of conditions where these events occur provides the opportunity to explore the efficacy of using lab-derived mechanical properties to investigate processes responsible for seismicity. Furthermore, we know more about the rheological properties of olivine - the most abundant mineral in the oceanic lithosphere - than perhaps any other mineral that is stable where earthquakes occur. Thus, we can directly test models for how asperity-scale deformation processes are manifest in frictional behavior, at both laboratory and geologic conditions. Finally, the occurrence of earthquakes at conditions where well-calibrated phase transitions occur provides the opportunity to evaluate the role of reactions for promoting a wide range of slip behaviors observed in subduction zone settings. In this presentation, I will first outline the conditions where earthquakes occur within the outer rise regions of subduction zones and compare these data with predictions based on extrapolation of lab data on the high-temperature frictional behavior of olivine. Using these observations as a constraint, I will then discuss mechanisms responsible for intermediate depth earthquakes, including the role of dehydration reactions in both peridotite and gabbroic lithologies. ![]() Professor Greg Hirth, Brown University, USA
![]() Professor Greg Hirth, Brown University, USAGreg Hirth studies experimental rock mechanics, deformation mechanisms and frictional properties in both crustal and mantle lithologies, structural geology, and the application of lab data to geophysical and geological problems. He focuses on the processes that control the mechanical behavior of rocks using optical and electron microscopy in conjunction with theoretical considerations. He received his BS from Indiana University, and PhD from Brown University working on the experimental deformation of quartz aggregates. After two years as a Postdoctoral Researcher at the University of Minnesota, he spent 14 years as a Research Scientist at the Woods Hole Oceanographic Institution. He returned to Brown as a Professor in 2007. He is a Fellow of the Mineralogical Society of America and the American Geophysical Union |
---|---|
09:45 - 10:30 |
Superplasticity in rocks
Uniaxial constant-displacement-rate tensile tests were conducted on samples of polycrystalline forsterite + periclase, forsterite + diopside and forsterite + enstatite + diopside. At subsolidus temperatures of 1250-450 degree, a pressure of one atmosphere and a strain rate of 10-5~10-4 /sec, elongation up to 500% was achieved, exhibiting the superplastic behavior (Hiraga et al. 2010 Nature). Grains of the same phases aggregated perpendicular to the tensile direction which is identified in natural mylonite composed of fine-grained minerals (Hiraga et al. 2013 Geology). During superplastic flow, dynamic grain growth and constant relative grain size among the phases which is determined by Zener relationship are observed, the latter of which is also identified in nature (Tasaka et al. JGR 2014). Crystallographic preferred orientation develops during superplastic flow at a certain condition (Miyazaki et al. 2013 Nature) which we consider the result of significant grain rotation during superplasticity. To confirm this prediction, we conducted experiments to observe such grain rotation directly. The result indicates that grain-boundary-sliding is a primary source for grain rotation. We will also discuss some issues on extrapolating flow laws constructed at experimental conditions to nature that can vary a wide range from 10-15 (mantle flow) to 102 /sec (seismic friction) in strain rate (!), for example. ![]() Professor Takehiro Hiraga, University of Tokyo, Japan
![]() Professor Takehiro Hiraga, University of Tokyo, JapanTakehiko Hiraga is an Associate Professor at the Earthquake Research Institute, University of Tokyo since 2006. He has established a unique laboratory, focused on crystal defects and in particular, on the structure, composition, and mechanical properties of grain boundaries in earth materials. Through several years of research and development, his lab has refined techniques that allow them to produce nano-materials which are ideal for investigating the properties of grain boundaries in rocks. Accomplishments with this synthetic geomaterial include developing a grain growth law for a polyminerallic system, superplastic deformation, and the development of a crystallographic preferred orientation during superplasticity. All these processes are controlled by grain boundary phenomena such as grain boundary diffusion, grain boundary sliding, and grain boundary migration. The goal of his research is to more fully understand grain boundary processes based on nano-scale structure and chemistry. This understanding can be used to explain a number of dynamic processes in the earth’s interior including flow and brittle failure of the crust and mantle. |
10:30 - 11:00 | Coffee |
11:00 - 11:45 |
Can grain size sensitive flow lubricate faults during the initial stages of earthquake propagation?
Recent friction experiments carried out under upper crustal P-T conditions have shown that microstructures typical of high temperature creep develop in the slip zone of experimental faults. These mechanisms are more commonly thought to control aseismic viscous flow and shear zone strength in the lower crust/upper mantle. In this study, displacement-controlled experiments have been performed on carbonate gouges at seismic slip rates (1 ms-1), to investigate whether they may also control the frictional strength of seismic faults at the higher strain rates attained in the brittle crust. At relatively low displacements (< 1cm) and temperatures (≤ 100 °C), brittle fracturing and cataclasis produce shear localisation and grain size reduction in a thin slip zone (150 μm). With increasing displacement (up to 15 cm) and temperatures (T up to 600 °C), due to frictional heating, intracrystalline plasticity mechanisms start to accommodate intragranular strain in the slip zone, and play a key role in producing nanoscale subgrains (≤ 100 nm). With further displacement and temperature rise, the onset of weakening coincides with the formation in the slip zone of equiaxial, nanograin aggregates exhibiting polygonal grain boundaries, no shape or crystal preferred orientation and low dislocation densities, possibly due to high temperature (> 900 °C) grain boundary sliding (GBS) deformation mechanisms. The observed micro-textures are strikingly similar to those predicted by theoretical studies, and those observed during experiments on metals and fine-grained carbonates, where superplastic behaviour has been inferred. To a first approximation, the measured drop in strength is in agreement with our flow stress calculations, suggesting that strain could be accommodated more efficiently by these mechanisms within the weaker bulk slip zone, rather than by frictional sliding along the main slip surfaces in the slip zone. Frictionally induced, grain size-sensitive GBS deformation mechanisms can thus account for the self-lubrication and dynamic weakening of carbonate faults during earthquake propagation in nature. ![]() Dr Nicola de Paola, Durham University, UK
![]() Dr Nicola de Paola, Durham University, UKNicola De Paola graduated in 2005 with a PhD in structural Geology at Durham University (UK), where he is currently employed as a Senior Lecturer in Structural Geology and Rock Mechanics. He has recently established and is currently managing the new Rock Mechanics Laboratory, in the Earth Sciences Department at Durham University (UK). The low to high velocity rotary shear apparatus (LHVRS) installed in the Rock Mechanics Laboratory, is one of the most up-to-date and efficient high velocity shear apparatus anywhere in the world. His research activity is related to the following topics: A) Mechanics of exhumed shear zones: Fault zone structure and architecture; Deformation processes and inferred slip behaviour; Fluids involvement in seismic faulting. B) Rock mechanics and laboratory experiments: Porosity and permeability evolution; Rheology of intact and fractured rocks; Fluids involvement in earthquake mechanics; Investigation of seismic faulting by high-velocity friction experiments. |
11:45 - 12:30 |
Phase-Transformation-Induced Nanometric Lubrication of Earthquake Sliding
Frictional sliding is strongly dependent on normal stress, hence the increase of pressure with depth in Earth prohibits slip initiation deeper than ~50km and requires that deeper earthquakes initiate by a mechanism other than overcoming of static friction. However, there is no similar constraint on the sliding mechanisms. High-pressure faulting is initiated by phase transformation and yields a thin nanocrystalline oxide “gouge” exhibiting extraordinarily low friction, even in the absence of a fluid. The only known physical mechanism that can explain this lubrication is flow of the “gouge” by grain-boundary sliding (gbs). High-speed friction experiments on a wide variety of rock types show similar very low frictional resistance of topologically indistinguishable, fully-dense, randomly-oriented nanocrystalline gouge consisting of oxides not present initially, suggesting that lubrication occurs by the same mechanism as in high-pressure faulting – flow by gbs of a nanocrystalline “gouge” formed by phase transformation in the first second(s) of sliding. Microstructures within the Punchbowl Fault, a deeply-eroded ancestral branch of the San Andreas Fault, are consistent with this argument. This mechanism intrinsically resolves two major conflicts between earlier laboratory results and natural faulting -- lack of a thermal aureole around major faults (San Andreas Fault heat-flow paradox) and the rarity of pseudotachylytes (shear-heating-induced endothermic reactions prevent temperature rise to melting). Despite this strong indirect evidence of extremely weak rheology of nanocrystalline oxides, there is no direct experimental confirmation. Accordingly, we are investigating the rheology of fully-dense nanocrystalline MgO. Preliminary results show that at moderate temperatures viscosity drops profoundly. ![]() Professor Harry W. Green, University of California, Riverside, USA
![]() Professor Harry W. Green, University of California, Riverside, USAHarry Green is Distinguished Professor of Geology and Geophysics at University of California, Riverside. He received all academic degrees at UCLA and postdoc in Materials Science at Case Western Reserve University. Taught 22 years UC Davis and last 21 years UC Riverside. Elected Fellow of AGU, MSA and AAAS. Recipient Bowen Award, AGU; Roebling Medal, MSA; numerous named lectureships. Professor Green investigates high-temperature, high-pressure rheology and petrology, principally of mantle rocks, and how stress and deformation interact with metamorphism and phase transformations. Concerning earthquakes, he and students/postdocs discovered faulting during the olivine®spinel phase transformation and its relevance to deep-focus earthquakes; determined that dehydration embrittlement is a viable earthquake mechanism, even if ∆V of reaction negative; and most recently, has demonstrated that the profound weakening observed in modern earthquake experiments is a result of phase transformation and consequent generation of a nanocrystalline gouge. He currently investigates the physics underlying this phenomenon. |
Chair
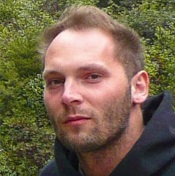
Dr Tom Mitchell, UCL, UK
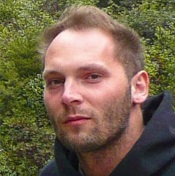
Dr Tom Mitchell, UCL, UK
Tom Mitchell is a lecturer in Earthquake Geology, Fault Mechanics and Experimental Rock Deformation at UCL. His research to date has been primarily concerned with two main areas;
- The experimental deformation of rocks under simulated geological conditions, in order to help interpret natural processes such as faulting and earthquake mechanics
- Detailed field studies on the structure and properties of strike-slip fault zones over a range of scales to further understand fault growth processes, subsequent mechanics, and bulk hydraulic and seismological properties of a fault zone
13:30 - 14:15 |
Interpreting slow and fast earthquakes in terms of friction
Dynamic stress drop events, or stick-slip, were identified as laboratory analogues of earthquakes nearly fifty years ago [Byerlee and Brace, 1968]. Developing scaling laws for relating stick-slip to earthquakes requires an understanding of both fault surface properties and loading frame characteristics (i.e., dynamic unloading response). Advances in high speed instrumentation reveal basic characteristics of stick-slip on bare granite-on-granite surfaces, including rapid stress drop, slip duration of 0.1-0.3 ms, average slip speed of 1-20 m/s, and at normal stresses above about 300 MPa, the common occurrence of surface melt and total stress drop. Dynamic friction systematically drops with increasing normal stress to µ ~0.2. Thermocouples embedded 2.5 mm from the fault surface provide total frictional heat production up to 120 kJ/m2, while 1D thermal modeling predicts peak surface temperature approaching 2000°C (consistent with observed surface melt) in 400 MPa confining pressure experiments. For slower stick-slip at low normal stress, a spring and lumped-mass-slider model provides a good representation of rupture dynamics. However, for short-duration events, the length and stiffness of the loading piston may be important in determining stress drop and event duration. Increasing confining pressure leads to systematic changes in stick-slip properties such as increased surface temperature, stress drop, event duration and average slip rate, as well as decreased average dynamic friction. Understanding these dynamic rupture processes in the laboratory will provide constraints on source mechanics of earthquakes. ![]() Dr David Lockner, US Geological Survey, USA
![]() Dr David Lockner, US Geological Survey, USADavid Lockner received an AB and MS in Geology from the University of Rochester and a PhD in Geophysics from the Massachusetts Inst. of Tech. He has spent his professional career at the U.S. Geological Survey, Earthquake Science Center in Menlo Park, where he runs a Rock Physics and Deformation laboratory. He has specialized in measuring physical properties of rocks and fault materials at the high pressures and temperatures found at earthquake nucleation depths. His interests include measurement of acoustic emissions produced during brittle deformation, rock friction and fracture, and other physical properties such as permeability, wave speed, electrical resistivity, and poroelastic properties. Current research interests include earthquake source properties, laboratory ‘stick-slip’ as an analog of earthquake rupture, and characterization of friction and transport properties of active faults. He is a recipient of the US Dept. of Interior Distinguished Service Award, the O. Yu. Schmidt Medal of the Russian Academy of Sciences, and a Fellow of the American Geophysical Union. |
---|---|
14:15 - 15:00 |
Fault gouge deformation during seismic slip
Improving our ability to recognize fault rock microstructures that are “diagnostic” of particular dynamic slip conditions (e.g. slip velocity, fluid pressure) is a major focus of current research in structural geology. As one example of recent progress, my talk will focus on new experiments designed to test the long-held idea that foliated fault rocks (i.e. foliated gouges and cataclasites) are the product of distributed (aseismic) fault creep. Foliated gouges and cataclasites are often found together with localized slip surfaces, the latter indicating potentially unstable (seismic) behavior. One possibility is that fault zones containing foliated fault rocks and discrete slip surfaces preserve the effects of both seismic slip and slower aseismic creep. An alternative possibility explored in our experiments is that some foliated fault rocks and localized slip surfaces develop contemporaneously during seismic slip. We studied the microstructural evolution of calcite-dolomite gouges deformed experimentally at slip velocities <1.13 m s-1 and for total displacements of 0.03 - 1 m, in the range expected for the average coseismic slip during earthquakes of Mw 3-7. As strain progressively localized in the gouge layers at the onset of high-velocity shearing, an initial mixed assemblage of calcite and dolomite grains evolved quickly to an organized, foliated fabric. Significant fabric evolution and grain size reduction in the bulk gouge occurred before and during dynamic weakening. Strain was localized to a bounding slip surface by the end of dynamic weakening and thus microstructural evolution in the bulk gouge ceased. Our experiments suggest that certain types of foliated gouge and cataclasite can form by distributed “brittle flow” as strain localizes to a slip surface during coseismic shearing. If true, the microstructure of such foliated gouges and cataclasites in natural fault zones will provide a new opportunity to collect data on the coseismic shear zone (e.g. evolution of thickness and grain size distribution) that have been difficult to access by other means. Our experimental foliated cataclasites that formed at high slip velocity bear striking resemblance to natural examples of foliated cataclasite preserved along active normal faults in the central Apennines of Italy, and ongoing work is focused on quantitatively comparing natural and experimental examples. ![]() Dr Steven Smith, University of Otago, New Zealand
![]() Dr Steven Smith, University of Otago, New ZealandSteven Smith is a lecturer in Structural Geology at the University of Otago, New Zealand. He obtained his PhD from Durham University (UK) in 2009 working on extensional detachment faults in central Italy. This was followed by 4 years of postdoctoral research at INGV, Rome, as part of a team of researchers developing the SHIVA rock deformation apparatus. His research integrates field and experimental data to understand the structure of fault zones and the microstructural record of fault slip. Current work includes investigating the mechanical behavior and microstructural evolution of natural and experimental fault gouges during the seismic cycle. |
15:00 - 15:30 | Tea |
15:30 - 16:16 |
Field geology observations on earthquake faults
![]() Dr Christie Rowe, McGill University, Canada
![]() Dr Christie Rowe, McGill University, CanadaChristie Rowe is a field geologist specializing in structural studies of faults and shear zones. She received her AB from Smith College, and PhD from UC Santa Cruz working on subduction thrust faults in the Kodiak Complex, Alaska. She was a lecturer at University of Cape Town for three years. This was followed by more challenging cultural exchange: 2 years post-doctoral fellowship in a seismology group (Brodsky). Since 2011 she has been the Wares Scholar at McGill University in Montréal, teaching field mapping and structural geology. She uses multi-scale observations of exhumed faults to interpret the rock record of brittle and brittle-ductile deformation, focusing on variation in deformation styles and strain rate across the seismic cycle. |
16:15 - 17:00 |
On the effective stress law for frictional sliding, and fault slip triggered by means of fluid injection
Fluid injection into deep rocks is increasingly used in connection with energy extraction and for disposal of fluid wastes. It is well known that small- to medium-scale seismicity can be induced or triggered by fluid injection. Fluctuations in pore fluid pressure may also be associated with natural seismicity. The energy release in anthropogenically-induced seismicity is strongly sensitive to the amount and pressure of fluid injected, as a result of the way in which seismic moment release is related to slipped area. It is also strongly affected by the hydraulic conductance of the faulted rock mass. Bearing in mind the scaling issues that apply, fluid injection driven fault motion can be studied on laboratory-sized samples. Here, we investigate both stable and unstable induced fault slip on pre-cut planar surfaces in two sandstones, Darley Dale and Pennant sandstones, with or without granular gouge. The rocks were chosen for their very different permeabilities to fluid flow, differing by a factor of 106, whilst their mineralogies are broadly comparable. In permeable Darley Dale sandstone fluid can access the fault plane through the rock matrix and the effective stress law is followed precisely. Pore pressure change shifts the whole Mohr circle laterally. In tight Pennant sandstone fluid only injects into the fault plane itself, and the stress state in the rock matrix is not affected. Sudden access by overpressured fluid to the fault plane via hydrofracture causes seismogenic fault slips. ![]() Professor Ernest H. Rutter, University of Manchester, UK
![]() Professor Ernest H. Rutter, University of Manchester, UKErnest Rutter is Professor of Structural Geology at the University of Manchester School of Earth, Atmospheric and Environmental Sciences. His research includes studies of natural rock fracture and flow through field studies, plus laboratory-based experimental rock mechanics and petrophysics, aimed at understanding rock behaviour in nature and under engineering conditions. The latter has implications for the stability of excavations and boreholes employed in the exploitation of natural resources, and the flow of fluids though rocks. Professor Rutter has been awarded the Wollaston Fund and the Lyell Medal of the Geological Society of London, the Néel Medal of the European Geosciences Union. He is an elected Fellow of the American Geophysical Union. |
17:00 - 17:45 | Poster session |
Chair
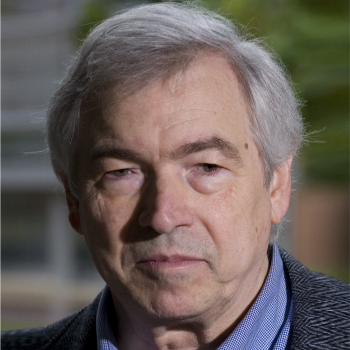
Professor James R. Rice ForMemRS, Harvard University, USA
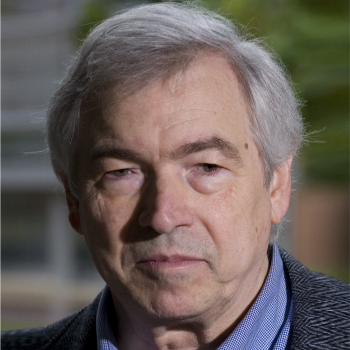
Professor James R. Rice ForMemRS, Harvard University, USA
James R. Rice is the Mallinckrodt Professor of Engineering Sciences and Geophysics at Harvard University, where he has been since 1981. From 1965 to 1981 he was an engineering faculty member at Brown University, and his earlier education was in mechanics at Lehigh University. His focus in recent years is on solid and fluid mechanics, and related materials and thermal science, as directed to earth and environmental problems. Those include fault zone shear processes, earthquake nucleation, dynamic rupture propagation, and aseismic deformation transients, tsunami propagation, meltwater interactions with glacier and ice sheet dynamics, landslide processes, and general hydrologic phenomena involving fluid interactions in deformation, flow and failure of earth materials. Earlier, his work focused on plastic deformation and cracking processes, principally in metals, as they arise in mechanical and materials engineering and on related computational and analytical methodology.
09:00 - 09:45 |
The mechanics of slow earthquakes and the spectrum of fault slip behaviors
Slow earthquakes represent an important conundrum in earthquake science. Whereas normal earthquakes are understood as dynamic instabilities with rupture expansion dictated by elastic wave speeds, there is no such understanding for slow earthquakes with rupture durations of months or more and fault slip speeds of ~100 micron/s or less. What determines the rupture propagation velocity in slow earthquakes and in other forms of quasi-dynamic rupture? What processes limit stress drop and fault slip speed in slow earthquakes? Existing lab studies provide some help, however these are mainly anecdotal and rarely include examples of repetitive slow slip that could isolate the underlying mechanisms. In this talk I summarize recent lab work documenting the complete spectrum of stick-slip behaviors, with systematic measurements of repetitive, stick-slip from transient slow slip to fast stick-slip. The work includes independent measurement of the stability parameter, kc = (b-a)/Dc. Slow slip occurs near the threshold between stable and unstable failure, controlled by the interplay of fault zone frictional properties, including rate dependence of the friction rate parameter a-b, and elastic stiffness of the surrounding rock. Our results suggest that slow earthquakes and transient fault slip behaviors can arise from the same governing frictional dynamics as normal earthquakes. The mechanism we propose could act in concert with other proposed processes, including fluid pressure and dilatancy, and provides a generic mechanism for slow earthquakes, consistent with the wide range of conditions for which slow slip has been observed. ![]() Dr Chris Marone, The Pennsylvania State University, USA
![]() Dr Chris Marone, The Pennsylvania State University, USAChris Marone is a professor of Geophysics in the Department of Geosciences at The Pennsylvania State University. He runs the rock mechanics lab at Penn State, specializing in design and construction of equipment for high-resolution measurement of rock properties. He co-directs the Center for Geomechanics, Geofluids, and Geohazards and is associate director of the Institute for Natural Gas Research at Penn State. His interests center on fault mechanics, friction, rock mechanics, earthquake physics, and poromechanics. Recent research foci include: 1) rate-state friction mechanics of fault rocks, fault healing and the application of laboratory derived friction laws to the spectrum of fault slip behaviors, 2) the mechanics of slow earthquakes, 3) poromechanics of deforming rocks, 4) earthquake triggering and the role of dynamic stressing in frictional instability, 5) granular mechanics, jamming and unjamming, and 6) the role of shear fabric and clay mineralogy on the frictional strength and constitutive properties of fault rocks. |
---|---|
09:45 - 10:30 |
Dynamic fault weakening due to thermal pressurization of pore fluids
High-velocity friction experiments and geophysical observations suggest that mature faults weaken dramatically during seismic slip. However, it is still unclear which coseismic weakening mechanisms are most important. Thermal pressurization is one possible coseismic weakening mechanism driven by the thermal expansion of native pore fluids, which leads to elevated pore pressures and significant coseismic weakening. While thermal pressurization has been studied theoretically for many decades, and invoked in recent earthquake simulations, its activation in laboratory experiments has remained elusive. Here we show that friction experiments on confined, fluid-saturated, thermally-cracked, low-permeability (1.3*10‐18 to 6.1*10‐19 m2) diabase rocks, at constant normal stresses in the range 25-100 MPa and initial pore pressures in the range 10–25 MPa, yield a rapid decay of shear stress following a step‐change in sliding velocity from 10 μm/s to 4.8 mm/s. In one test, the decrease in shear stress of ~20% over the first 28 mm of slip at 4.8 mm/s agrees closely with the theoretical solution for slip on a plane [Rice, 2006], with an inferred weakening length parameter L* of ~500 mm, close to the value predicted by inserting laboratory-determined rock and fluid properties into Rice’s formula for L*. In another test, steps from 10 μm/s to three different velocities - 1.2 mm/s, 2.4 mm/s, and 4.8 mm/s - yield data that all fit the Rice solution with values of L* that vary systematically with velocity as predicted by the theory. To our knowledge, this is the best experimental validation of thermal pressurization to date. ![]() Professor David Goldsby, University of Pennsylvania, USA
![]() Professor David Goldsby, University of Pennsylvania, USADavid Goldsby is an Associate Professor in the Department of Earth and Environmental Sciences at the University of Pennsylvania. He is an ‘experimental geophysicist’ with research interests in materials science, tribology, rock mechanics, glaciology and earthquake mechanics. A particular focus of Dr. Goldsby’s research is understanding deformation mechanisms in planetary materials and how they control large-scale geophysical phenomena, such as the nucleation of earthquakes and the motion of the Earth’s great ice sheets. Dr. Goldsby completed his PhD in Geophysics at the University of Minnesota in 1997, and was an Associate Professor (Research) at Brown University until 2014. He is a long-time member of the American Geophysical Union and the Southern California Earthquake Center, and Facilities Chair of the new rock mechanics consortium DEFORM (Deformation Experimentalists at the Frontier Of Rock and Mineral Research). |
10:30 - 11:00 | Coffee |
11:00 - 11:45 |
Effects of load path on rock damage at high strain rates: implications for earthquake rupture
Various fault damage fabrics, from gouge and rock flower in the principal slip zone, to fragmented and pulverized rocks in the fault damage zone, have been attributed to brittle deformation at high strain rates during earthquake rupture. It has been proposed that these textures are indicative of deformation at high strain rates during catastrophic events such as earthquake rupture. These fault zone fabrics are significant both in terms of the information they contain about the mechanisms by which they were formed and for how they influence future earthquake ruptures. Experimental studies of these textures have focused primarily on experiments using the Split Hopkinson Pressure Bar, with which samples are loaded by a uniaxial compressive pulse, and the results of these studies suggest overwhelmingly that there exists a critical threshold in stress-strain rate space through which rock failure transitions from failure along a few discrete fracture planes to pulverization. We present new experimental results on Arkansas Novaculite and Westerly Granite in which we demonstrate that in addition to peak stress and strain rate, the fragmentation-pulverization transition is strongly dependent on the complete loading path as defined classically in stress-strain space. We also present new data on fracture surface area produced during the experiments. The results have important implications for the coseismic work budget and the scaling of SHPB experimental results to natural fault zones. ![]() Dr W. Ashley Griffith, University of Texas, Arlington, USA
![]() Dr W. Ashley Griffith, University of Texas, Arlington, USAAshley Griffith is an assistant professor of Earth and Environmental Science at the University of Texas at Arlington. His research focuses on structural geology and geomechanics, with emphasis on the behavior of fractures in the earth's crust. Current and past research applications include earthquake physics (including frictional behavior of rocks and coseismic off-fault deformation), crustal deformation, igneous and clastic intrusions, rock physical properties, and geological hazards in underground openings. Dr. Griffith served as an NSF international postdoctoral scholar at the Istituto Nazionale di Geofisica e Vulcanologia in Rome from 2008-09. He earned a Ph.D. in Geological and Environmental Science from Stanford University in 2008, an M.S. in Geosciences from the University of Massachusetts in 2003, and a B.S. in Geology from the College of William and Mary in 1999. |
11:45 - 12:30 |
Professor Giulio Di Toro, University of Manchester, UK
Moderate to large earthquakes often propagate along faults cutting carbonate rocks (dolostones, limestones, etc.). Compared to silicate-bearing rocks, which melt, weaken and wear when sheared at seismic slip rates (ca. 1 m/s), carbonate rocks do not melt, the minimum friction coefficient is very low (down to 5% of static friction) and the wear rate negligible. In cohesive carbonate rocks, experiments simulating seismic deformation conditions and stopped at slip initiation (< 5 mm) suggest that initial frictional decay down to 50% of static friction is associated with CO2 emission plus formation of nanograins and amorphous carbon. Experiments stopped at larger slips (> 5 mm) show that the slipping zone consists of a foam-like sintered surface overlying a microporous fabric made of calcite and lime (plus periclase in dolostones) nanograins. Experiments with pressurized H2O show that the contribution of thermal pressurization is negligible. We propose that initial frictional weakening is due to the formation of patches of amorphous carbon (solid lubricant) at asperity contacts. With progressive slip and bulk temperature increase, nanograins accommodate large strain rates (ca. 104 s-1) by grain size dependent processes, as suggested by several authors. The presence of a microporous fabric boosts pore-controlled diffusive process propelled by CO2 gas exhaust due to decarbonation. Enhanced pore-controlled diffusive processes allow (1) efficient mass transfer during sliding and (2) sintering of the nanograins into a foam-like surface at the end of the experiment. The sintered surface is strikingly similar to the one found in natural faults cutting carbonate rocks. ![]() Professor Giulio Di Toro, University of Manchester, UK
![]() Professor Giulio Di Toro, University of Manchester, UKGiulio Di Toro is Chair in Geology at the University of Manchester and Associate Researcher at the Istituto Nazionale di Geofisica e Vulcanologia (Rome). In collaboration with geologists, geophysicists, engineers and enthusiast graduate and post-graduate scholars, he integrated field data from exhumed seismogenic faults with experimental observations to understand the physico-chemical processes active during the seismic cycle. The scientific and technological results of this collaboration include the investigation of several fault weakening processes that may operate during earthquakes and the development of SHIVA, the most powerful earthquake simulator installed worldwide. His research activities were also supported by two European Research Council Grants (USEMS & NOFEAR, 2008-2019, 4 M€). He was awarded of the Arne Richter Medal (2008) of the European Geosciences Union and of the Premio Tartufari for Geology (2010) of the Accademia Nazionale dei Lincei. He is member of the Academia Europeae and Accademia Galileiana. |
Chair
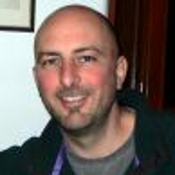
Dr Stefan Nielsen, Durham University, UK
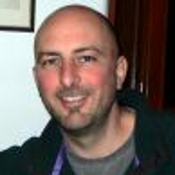
Dr Stefan Nielsen, Durham University, UK
Stefan Nielsen is an Earth scientist with expertise in the area of earthquake source mechanics. His contributions to the theoretical seismology include: (1) The mathematical prediction of self-similar, self-healing rupture pulse which proposes an appealing alternative to the classical Kostrov crack as a mathematical idealization of seismic rupture. This model provides a theoretical explanation of the self-healing rupture pulses which have been documented on seismic faults. (2) The prediction of a powerlaw relation between shear and normal stress in dynamic lubrication of faults during seismic rupture, in the presence of frictional melt (frictional melt is a process whose footprint can be observed on several fossil faults). Several experiments have since confirmed the prediction of S.N.'s lubrication powerlaw. In addition to his theoretical interests, S.N. diversified his research on seismic faulting by conducting field work on exhumed fossil faults and on pseudotachylite (solidified product of coseismic frictional melt). Under the awareness that friction is poorly resolved by seismological data and modeling, S.N. has been developing an experimental approach for analog experimentation on dynamic rupture and for high-velocity frictional experiments on rocks. S.N. is currently Reader at Durham University (UK).
13:30 - 14:15 |
Title TBC
![]() Professor Raul Madariaga, Ecole Normale Superieure, France
![]() Professor Raul Madariaga, Ecole Normale Superieure, FranceRaul Madariaga is professor emeritus in the Department of Geosciences of Ecole Normale Superieure in Paris (ENS). He obtained a degree of Civil engineering from the University of Chile in 1967 and a PhD in geophysics from the Massachusetts Institute of Technology in 1972. After a brief stay at the University of Chile from 1971-1973, he became a researcher at MIT’s Earth and Planetary Sciences from 1974-1977. In 1977 he moved to France where he became a professor of Geophysics at the University Paris 7 and the Institut de Physique du Globe (IPGP). In 1998 he moved as a distinguished professor at ENS, where he is still working. In France he was the head of the Seismological laboratory of IPGP from 1985 to 1996 and at ENS he chaired the Geology Laboratory from 2000 to 2006. Raul became a Fellow of the American Geophysical Union (1991) and was a Senior Member of the Institut Universitaire de France (1993-1998). He received the Prix Antoine D'Abbadie de l'Académie des Sciences (1992), Grand medal of the Rectorate of the University of Chile (1998), Stephan Mueller Medal of the European Geophysical Society (1999) and the Harry F. Reid Medal of the Seismological Society of America (2004). He is the co-author of two books: Madariaga, R. and G. Perrier, Les Tremblements de Terre, Les Editions du CNRS, 1991 and Udias, A. R. Madariaga, E. Buforn, Source Mechanisms of Earthquakes, Cambridge University. |
---|---|
14:15 - 15:00 |
Earthquake sequence simulations using measured mechanical properties for JFAST core samples and blueschist
Since the 2011 Tohoku earthquake, multi-disciplinary observational studies have promoted our understanding of both coseismic and long-term behaviour of the Japan Trench subduction zone. Investigation of tsunami deposits (Sawai et al., 2012 and 2015) inferred recurrence interval of great tsunamigenic earthquakes between 500 and 800 years, while there are more frequent M7 events which fail to propagate to the trench. Long-term heat generation along the fault was inferred to be comparable to the effective friction coefficient (dissipation per slip/overburden) of about 0.025 from heat-flow measurements (Gao and Wang, 2014). The Japan Trench Fast Drilling Project (JFAST) revealed coseismic frictional heating of about 27 MJ/m2 (Fulton et al., 2013) in the shallowest potion of the fault where about 50 m of coseismic slip was generated (e.g., Fujiwara et al., 2011). We also have suggestions for mechanical properties of the fault from experimental side. Fault materials obtained in JFAST was investigated in terms of hydraulic (Tanikawa et al., 2013) and frictional properties (Sawai, 2015, Doctor Thesis) under hydrothermal conditions. Frictional properties of blueschist, stable metamorphic rock in the deeper part of the ruptured area in the Tohoku earthquake, were also investigated under hydrothermal conditions (Sawai et al., submitted to GRL). The model-parameter space of earthquake sequence simulation is huge, and those experiments are useful in decreasing the degree of freedom. In the talk, numerical models of earthquake sequences (e.g,. Lapusta et al., 2000) will be presented which account for the experiments and are consistent with the observational studies raised here. ![]() Dr Hiroyuki Noda, Japan Agency for Marine Science and Technology, Japan
![]() Dr Hiroyuki Noda, Japan Agency for Marine Science and Technology, JapanHiroyuki Noda is a scientist in Japan Agency of Marine-Earth Science and Technology (JAMSTEC). He received his Bachelor of Science in 2003 and Master of Science in 2005 at Department of Geology and Mineralogy, Kyoto University. During his doctoral course, he stayed at Harvard University as an international special student and as a visiting fellow, and received Doctor of Science in 2008 at Department of Geophysics, Kyoto University. Then he worked as a post-doctoral researcher in Hiroshima University and in California Institute of Technology before moving to JAMSTEC in 2011. His research interest includes frictional properties of rock under a wide range of deformation conditions, and its implication to fault motion such as earthquakes and a sequence of them. |
15:00 - 15:30 | Tea |
16:15 - 17:00 |
Heating, weakening and shear localization in earthquake rupture
Field and borehole observations of active earthquake fault zones show that shear is often localized to principal deforming zones of order 0.1 mm to 10 mm width. The presentation addresses how frictional heating in rapid slip weakens faults dramatically, relative to their static frictional strength, and promotes such intense localization. Pronounced weakening occurs even on dry rock-on-rock surfaces, due to flash heating effects, at slip rates above ~0.1 m/s (it is noted that earthquake slip rates are of order 1 m/s). But weakening in rapid shear is also predicted theoretically in thick fault gouge in the presence of fluids (whether native ground fluids or volatiles such as H2O or CO2 released by thermal decomposition reactions), and the predicted localization is compatible with such narrow shear zones as have been observed. The underlying concepts show how fault zone materials with high static friction coefficients, ~0.6 to 0.8, can undergo strongly localized shear at effective dynamic friction coefficients of order 0.1, thus fitting observational constraints, e.g., of earthquakes producing negligible surface heat out-flow and, for shallow events, rarely creating extensive melt. The results to be summarized include those of collaborative research published with Nicolas Brantut (Univ. Col. Lond.), Eric Dunham (Stanford Univ.), Nadia Lapusta (Caltech), Hiroyuki Noda (JAMSTEC, Japan), John D. Platt (Carnegie Inst. Sci.), Alan Rempel (Oregon State Univ.) and John W. Rudnicki (Northwestern Univ.). ![]() Professor James R. Rice ForMemRS, Harvard University, USA
![]() Professor James R. Rice ForMemRS, Harvard University, USAJames R. Rice is the Mallinckrodt Professor of Engineering Sciences and Geophysics at Harvard University, where he has been since 1981. From 1965 to 1981 he was an engineering faculty member at Brown University, and his earlier education was in mechanics at Lehigh University. His focus in recent years is on solid and fluid mechanics, and related materials and thermal science, as directed to earth and environmental problems. Those include fault zone shear processes, earthquake nucleation, dynamic rupture propagation, and aseismic deformation transients, tsunami propagation, meltwater interactions with glacier and ice sheet dynamics, landslide processes, and general hydrologic phenomena involving fluid interactions in deformation, flow and failure of earth materials. Earlier, his work focused on plastic deformation and cracking processes, principally in metals, as they arise in mechanical and materials engineering and on related computational and analytical methodology. |