Links to external sources may no longer work as intended. The content may not represent the latest thinking in this area or the Society’s current position on the topic.
Chemical biology approaches to assessing and modulating mitochondria
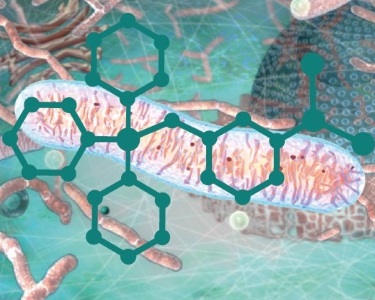
Theo Murphy scientific meeting organised by Dr Michael Murphy.
Mitochondria are central to many current biomedical problems. In these, variations in chemical processes are critical, but progress in understanding these and in developing therapies has been hampered by lack of methods to measure and manipulate mitochondrial 'chemistry'. This meeting will explore the interface of chemical biology and mitochondrial studies to open up new avenues for research and therapeutic interventions.
Speaker biographies and the schedule of talks are available below. Alternatively you can download the draft programme (PDF). Speaker abstracts will be available closer to the meeting date. Recorded audio of the presentations, subject to the speakers' permission, will be made available on this page after the meeting has taken place.
Attending this event
This is a residential conference, which allows for increased discussion and networking. It is free to attend, however, participants need to cover their accommodation and catering costs if required. Catering should be booked via the Royal Society registration system when registering for the meeting. Lunch is priced at £24 per day, and the conference dinner on the evening of Monday 26 September is priced at £40.
There is an additional £24 arrival buffet dinner on the evening of 25 September – this is for those who have booked accommodation at Chicheley Hall and wish to have dinner on the arrival night. If you are not staying at Chicheley Hall then of course this will not apply to you. Should you want to book this arrival buffet please contact the events team using the link below:
Enquiries: contact the events team.
Organisers
Schedule
Chair
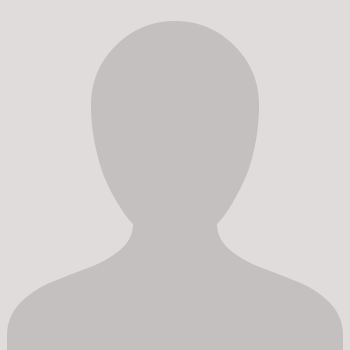
Sir Salvador Moncada FRS, University College London
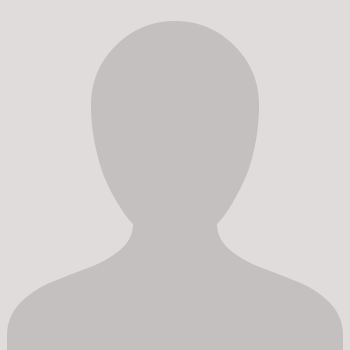
Sir Salvador Moncada FRS, University College London
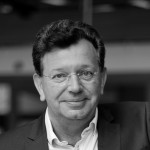
Professor Jan Smeitink, Radboud University Nijmegen, The Netherlands
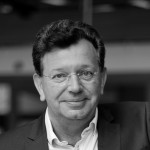
Professor Jan Smeitink, Radboud University Nijmegen, The Netherlands
Jan Smeitink obtained his training in Paediatrics at the Radboud university medical center, Nijmegen, The Netherlands. He completed his training in the field of Inborn Errors of Metabolism at the Wilhelmina Children’s Hospital, Utrecht, The Netherlands. He has been Head of the Department of Metabolic Diseases at the Radboud university medical center since 1996, and has a special interest in the study of mitochondria in health and disease. Jan Smeitink and his team developed clinical scoring systems, described different new phenotypes of patients harbouring mitochondrial disorders, elucidated most of the nuclear complex I genes, described the first mutations in these genes, described the first complex I assembly pathway and detected new assembly genes and mutations. They found the first translation elongation defect, studied the cell biological consequences of oxidative phosphorylation disorders, in particular complex I. They developed numerous new assays. Current focus is on drug development for which Smeitink established Khondrion BV, a mitochondrial medicine company.
09:05 - 09:35 |
Strategies to assess and intervene in mitochondria
Over the past decade mitochondrial function and dysfunction have turned out to be so central to biomedical questions that we are no longer surprised to read papers where mitochondria are involved in pathways as diverse as innate immunity, oxygen sensing and response to viral infections. Consequently we want to know more about how mitochondria function and go wrong in vivo. Furthermore, as mitochondria are cropping up in so many human pathologies there is a growing interest in developing therapies focussed on preventing mitochondrial damage. In both these areas the development of biological chemistry approaches is a clear way to both develop new probes of mitochondrial function in vivo and in coming up with new therapies. Here Professor Murphy will survey approaches that have been used to date and suggest possible ways forward for the emerging field of the biological chemistry of the mitochondrion. ![]() Professor Mike Murphy FMedSci, University of Cambridge
![]() Professor Mike Murphy FMedSci, University of CambridgeMike Murphy received his BA in chemistry at Trinity College, Dublin in 1984 and his PhD in Biochemistry at Cambridge University in 1987. After stints in the USA, Zimbabwe, and Ireland he took up a faculty position in the Biochemistry Department at the University of Otago, Dunedin, New Zealand in 1992. In 2001 he moved to the MRC Mitochondrial Biology Unit in Cambridge, UK (then called the MRC Dunn Human Nutrition Unit) where he is a programme leader. Murphy's research focuses on the roles of reactive oxygen species in mitochondrial function and pathology. In particular he has pioneered the targeting of bioactive and probe molecules to mitochondria in vivo. Murphy is Professor of Mitochondrial Redox Biology at the University of Cambridge, a Wellcome Trust Investigator, honorary research Professor at the University of Otago, New Zealand, a recipient of the Keilin Medal from the Biochemical Society, an honorary Fellow of the Royal Society of New Zealand and a Fellow of the Academy of Medical Sciences (FMedSci). |
|
---|---|---|
09:35 - 10:10 |
Delivering large bioactive molecules to mitochondria
Mitochondria are central to human health and disease, hence there is considerable interest in developing mitochondrion-targeted therapies that require the delivery of peptides or nucleic acid oligomers. However, progress has been impeded by the lack of a measure of mitochondrial import of these molecules. Here, we address this need by quantitatively detecting molecules within the mitochondrial matrix. We used a mitochondrion-targeted cyclooctyne (MitoOct) that accumulates several-hundredfold in the matrix, driven by the membrane potential. There, MitoOct reacts through click chemistry with an azide on the target molecule to form a diagnostic product that can be quantified by mass spectrometry. Because the membrane potential-dependent MitoOct concentration in the matrix is essential for conjugation, we can now determine definitively whether a putative mitochondrion-targeted molecule reaches the matrix. This "ClickIn" approach will facilitate development of mitochondrion-targeted therapies. ![]() Professor Robert Lightowlers, Wellcome Trust Centre for Mitochondrial Research, Newcastle University, UK
![]() Professor Robert Lightowlers, Wellcome Trust Centre for Mitochondrial Research, Newcastle University, UKRobert Lightowlers received his BSc (Hons) in Biological Sciences from UEA in 1983, and a PhD in Microbial Genetics from ANU Canberra in 1988. Following a postdoctoral position at the Institute of Molecular Biology in Eugene, Oregon, USA, where he gained a lifelong interest in mitochondria and mitochondrial disease, Lightowlers returned to the UK, where he established his laboratory at Newcastle University and, with Professor Doug Turnbull, the Mitochondrial Research Group (MRG). Lightowlers has been employed by Newcastle University since that date, being awarded a Personal Chair in 2000. The MRG has expanded enormously and led to formation of the Wellcome Trust Centre for Mitochondrial Research in 2012. Lightowlers was appointed Director of the Institute for Cell and Molecular Biosciences in 2012. His main research topic during this time has been into determining the molecular mechanisms that underlie expression of this genome in man and searching for a cure for these disorders. |
|
11:05 - 11:40 |
Peptide probes for mitochondrial chemical biology
A major challenge to the study of mitochondrial processes and the development of mito-targeted therapies is presented by the impermeability of the innermost mitochondrial membrane and its highly negative membrane potential, which exclude most exogenous molecules from the organelle. We have developed a class of peptide-based mitochondria-targeting vectors that deliver various cargos to this previously impenetrable organelle. We have used these vectors to understand the chemical requirements for mitochondrial entry, to study the effects of mitochondrial DNA damage, and to establish the presence of proteins not previously included in the mitochondrial proteome. Insights into the unique chemical and biochemical features of this organelle gained from the use of these conjugates will be presented. ![]() Professor Shana Kelley, University of Toronto, Canada
![]() Professor Shana Kelley, University of Toronto, CanadaDr Shana Kelley is a Distinguished Professor of Pharmaceutical Sciences, Chemistry, Biochemistry, and Biomedical Engineering at the University of Toronto. Dr Kelley received her PhD from the California Institute of Technology and was a NIH postdoctoral fellow at the Scripps Research Institute. The Kelley research group works in a variety of areas spanning bioanalytical chemistry, chemical biology and nanotechnology. Shana’s group has developed novel electrochemical sensors that enable ultrasensitive nucleic acids detection for clinical diagnostics, and has also investigated a new set of chemical probes that interact with mammalian mitochondria. Dr Kelley’s work has been recognised with a variety of distinctions, including being named one of ‘Canada’s Top 40 under 40’, a NSERC E.W.R. Steacie Fellow, the 2011 Steacie Prize, and the 2016 NSERC Brockhouse Prize. She has also been recognised with the Pittsburgh Conference Achievement Award, an Alfred P. Sloan Research Fellowship, a Camille Dreyfus Teacher-Scholar award, a NSF CAREER Award, a Dreyfus New Faculty Award, and was also named a ‘Top 100 Innovator’ by MIT’s Technology Review. She is a founder of two molecular diagnostics companies, GeneOhm Sciences (acquired by Becton Dickinson in 2005) and Xagenic Inc. |
|
11:40 - 12:10 |
Regulation of mammalian mtDNA gene expression
Expression of mammalian mitochondrial DNA (mtDNA) is regulated from both strands through special promoter elements denoted the heavy and light strand promoters and the activity of the basal mitochondrial transcription machinery. The nuclear-encoded basal transcription machinery of mammalian mitochondria was found to be a three-component system consisting of the mitochondrial RNA Polymerase (POLRMT) and the mitochondrial transcription factors A (TFAM) and B2 (TFB2M). Together these three factors are absolutely necessary and sufficient to obtain promoter-specific initiation of mtDNA transcription in vitro and in vivo. Mitochondrial transcription can be reconstituted in a pure in vitro system consisting of a promoter-containing DNA fragment and the basal transcription machinery, which was used in a high-throughput approach to identify low-molecular weight inhibitors targeting POLRMT (Inhibitors of mitochondrial transcription, IMTs) in collaboration with the Lead Discovery Center in Dortmund, Germany. In the present study, we have identified potent and specific inhibitors of POLRMT that were analyzed for their cellular activity. In line with the central role of POLRMT in mitochondrial transcription, IMT treatment led to a dose- and time-dependent decrease in mitochondrial gene expression. Strikingly, IMTs also affected growth of tumor cell spheroids in cell culture and display cytotoxicity against some human tumor cell lines in vitro. Application of IMTs to mouse cohorts leads to reduction of mitochondrial gene expression in different tissues, but is very well tolerated. In the next step, mice harboring human xenograft tumors will be treated and long-term toxicity will be investigated. ![]() Professor Nils-Göran Larsson, Karolinska Institutet, Sweden
![]() Professor Nils-Göran Larsson, Karolinska Institutet, SwedenNils-Göran Larsson studied medicine and defended his PhD thesis in mitochondrial genetics in 1992 at the University of Gothenburg, Sweden. In 1994, he became a specialist in paediatrics. From 1994 – 1997 he worked as a HHMI postdoctoral fellow at Stanford University School of Medicine, California. He returned to Sweden, in 1997 and started working at Karolinska Institutet, where he became professor in mitochondrial genetics in 2004. He is a member of the Nobel Assembly at Karolinska Institutet and a member of the Royal Academy of Sciences. He has won several national awards for his research. In 2008, he took up a position as Director at the newly founded Max Planck Institute for Biology of Ageing in Cologne, Germany. He is currently working at Karolinska Institutet and remains affiliated with the Max Planck Institute for Biology of Ageing. |
Chair
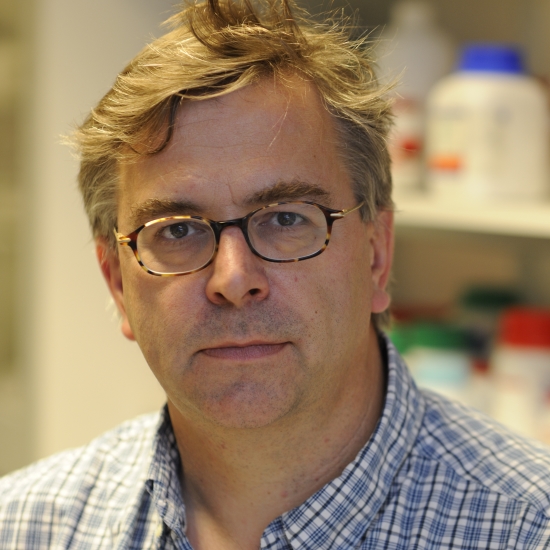
Professor Mike Murphy FMedSci, University of Cambridge
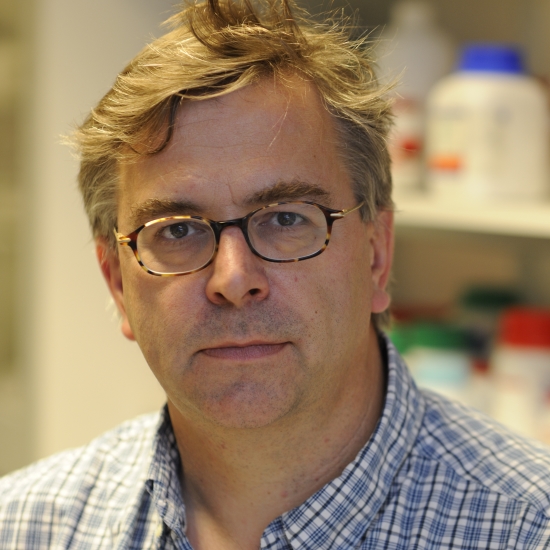
Professor Mike Murphy FMedSci, University of Cambridge
Mike Murphy received his BA in chemistry at Trinity College, Dublin in 1984 and his PhD in Biochemistry at Cambridge University in 1987. After stints in the USA, Zimbabwe, and Ireland he took up a faculty position in the Biochemistry Department at the University of Otago, Dunedin, New Zealand in 1992. In 2001 he moved to the MRC Mitochondrial Biology Unit in Cambridge, UK (then called the MRC Dunn Human Nutrition Unit) where he is a programme leader. Murphy's research focuses on the roles of reactive oxygen species in mitochondrial function and pathology. In particular he has pioneered the targeting of bioactive and probe molecules to mitochondria in vivo. Murphy is Professor of Mitochondrial Redox Biology at the University of Cambridge, a Wellcome Trust Investigator, honorary research Professor at the University of Otago, New Zealand, a recipient of the Keilin Medal from the Biochemical Society, an honorary Fellow of the Royal Society of New Zealand and a Fellow of the Academy of Medical Sciences (FMedSci).
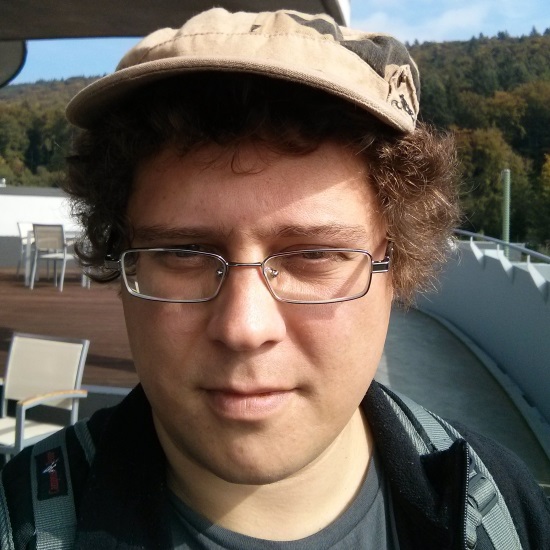
Dr Vsevolud Belousov, Russian Academy of Sciences, Russia
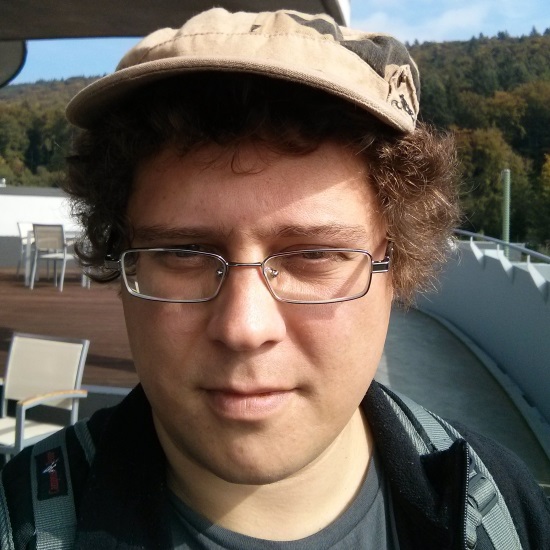
Dr Vsevolud Belousov, Russian Academy of Sciences, Russia
Vsevolod Belousov received his Masters degree in biochemistry at Moscow State University, Russia in 1998 and PhD in Biochemistry at AN Belozersky Institute of Physical and Chemical Biology in 2002. After two years in Evrogen, where he was developing photoactivatable fluorescent proteins, he joined Segrey Lukyanov’s lab in the Shemyakin-Ovchinnikov Institute of Bioorganic Chemistry in Moscow to work on a genetically encoded H2O2 indicator. Since 2008 he has been a Redox biology group leader in the Institute of Bioorganic Chemistry. His interests are in genetically encoded redox probes development, super-resolution microscopy, optogenetics, metabolic engineering and anti-ischemic drugs development.
13:30 - 14:05 |
Use of fluorescent proteins as mitochondria redox probes
Hydrogen peroxide produced by mitochondria can act as a signaling molecule. It is increasingly realized that H2O2 signal transmission depends on thiol peroxidases which form redox relay chains with other proteins. The redox relay principle can be exploited to monitor endogenous H2O2 generation inside and outside mitochondria, in living cells and in real-time. These concepts and approaches now help to clarify which environmental, genetic or pharmacological perturbations impact on mitochondrial H2O2 emissions and signaling. ![]() Dr Tobias Dick, German Cancer Research Center (DKFZ), Germany
![]() Dr Tobias Dick, German Cancer Research Center (DKFZ), GermanyTobias Dick graduated in biochemistry (FU Berlin) and obtained a PhD in molecular immunology with Hans-Georg Rammensee at the University of Tübingen. He was a Postdoctoral Fellow with Peter Cresswell at Yale University and then continued as an independent group leader in the field of Redox Biology at the German Cancer Research Center (DKFZ) in Heidelberg. In 2010, he was appointed Head of Division of Redox Biology at DKFZ. Dr Dick works on the molecular mechanisms of redox signalling and protein redox regulation. He develops tools for the visualisation and manipulation of redox processes in vivo. He uses these tools to investigate adaptive stress responses in normal and tumour cells. |
|
---|---|---|
14:05 - 14:40 |
Molecular imaging approaches to studying redox biology in the brain
The exploration of the brain and its distinctive role in forming the centre of consciousness offers a grand challenge for achieving a molecular-level understanding of its unique functions, including learning and memory, as well as senses like sight, smell, and taste. As such, the brain also represents a frontier for developing new therapeutics for aging, stroke, and neurodegenerative diseases. We are developing molecular imaging approaches as a way to identify and study the underlying chemistry that governs brain activity. This talk will present our latest results in the discovery and understanding of reactive oxygen, sulphur, and carbon species as emerging new chemical signals and their influence on neural circuitry. Professor Christopher Chang, University of California, Berkeley, USA
Professor Christopher Chang, University of California, Berkeley, USAChristopher J. Chang is the Class of 1942 Chair Professor of Chemistry and Molecular and Cell Biology and HHMI Investigator at UC Berkeley, as well as a Faculty Scientist in the Chemical Sciences Division of Lawrence Berkeley National Laboratory. He was born in Ames, IA and received his B.S. and M.S. degrees from Caltech in 1997, working with Professor Harry Gray. After spending a year as a Fulbright scholar in Strasbourg, France with Dr Jean-Pierre Sauvage, Chris received his PhD from MIT in 2002 under the supervision of Professor Dan Nocera. He stayed at MIT as a postdoctoral fellow with Professor Steve Lippard and then began his independent career at UC Berkeley in 2004. Research in the Chang lab is focused on chemical biology and inorganic chemistry, with particular interests in molecular imaging and catalysis applied to neuroscience, metabolic and infectious diseases, and sustainable energy. His group's research has been honoured by awards from the Dreyfus, Beckman, Sloan, and Packard Foundations, Amgen, AstraZeneca, and Novartis, AFAR, Technology Review, ACS (Cope Scholar, Eli Lilly Award in Biological Chemistry), RSC (Transition Metal Chemistry), and the Society for Biological Inorganic Chemistry, and in 2013 Chris was awarded the Noyce Prize at UC Berkeley for excellence in Undergraduate Teaching. Most recently Chris received the 2013 ACS Nobel Laureate Signature Award in Graduate Education, 2013 Baekeland Prize, and 2015 Blavatnik Laureate in Chemistry. He is a Senior Editor at ACS Central Science. |
|
15:30 - 16:00 |
Chemical biology of H2S signaling: the role of mitochondria
Hydrogen sulphide (H2S) is a gasotransmitter involved in the regulation of blood pressure and synaptic plasticity. More importantly H2S has a strong therapeutic potential in treating ischemia-reperfusion injury. The mechanisms behind many (patho)physiological roles assigned to H2S are, however, still elusive. The cross-talk of H2S with NO (and its metabolites) started emerging as a mechanistic concept that can explain some of the physiological effects assigned to H2S. Several new signalling molecules have been identified as products of the above-mentioned cross-talk. Endogenous H2S generation seems to be important for the process of tran-S-nitrosation in the cells, presumably through the formation of thionitrous acid (HSNO). Mitochondrial hem centres play particular role in HSNO generation. Furthermore, H2S reacts directly with NO to generate nitroxyl (HNO), which then activates the TRPA1 channel to allow Ca2+ influx into sensory nerve endings. This stimulates the release of the strongest known vasodilator, calcitonin gene-related peptide. On the other hand, protein persulfidation, an oxidative posttranslational modification of cysteine residues, is also believed to be responsible for most of biological effects controlled by H2S. Majority of protein persulfidation is located in mitochondria and mercaptopyruvate sulfur transferase, an H2S producing enzyme predominantly located in mitochondria, plays important role in this process. Furthermore, in order to be regulatory, protein persulfidation would have to be tightly regulated, i.e. the mechanism for protein de-persulfidation would have to exist. We discovered recently that thioredoxin system acts as depersulfidase, controlling thus the H2S signaling. The role of mitochondria in this process will be additionally discussed. ![]() Dr Milos Filopovic, University of Bordeaux, IBGC UMR 5095 and CNRS, IBGC, UMR 5095, France
![]() Dr Milos Filopovic, University of Bordeaux, IBGC UMR 5095 and CNRS, IBGC, UMR 5095, FranceMilos R. Filipovic graduated biochemistry from the University of Belgrade, Serbia, where he also obtained his PhD in 2008. After a short visit to L’Ecole Normal Supérieure in Paris, as a FEBS fellow, Milos joined the Bioinorganic Chemistry Division at Friedrich-Alexander University Erlangen-Nuremberg, first as a postdoc, and then as an independent group leader/habilitant. In 2015 he received ATIP-Avenir and Idex Junior Chair grants, and was subsequently appointed as a group leader at CNRS, Institute of Cellular Biochemistry and Genetics UMR5095, at the University of Bordeaux. His research is focused on biochemical mechanisms behind the intracellular signalling by gasotransmitters (nitric oxide and hydrogen sulphide), on their cross-talk and on post-translational modifications of proteins caused by these gaseous molecules. Most recently he has become particularly interested in signalling by H2S in mitochondria. |
|
16:00 - 16:35 |
Nitric oxide and mitochondria
Nitric Oxide (NO) inhibits mitochondrial cytochrome c oxidase (Complex IV) in a reversible manner and at physiological concentrations. Its affinity for NO is greater than that for oxygen (O2) suggesting that NO might regulate O2 consumption or interfere with its usage in pathological situations. For review see, [1]. We discovered that long-term inhibition of Complex IV led to persistent inhibition of complex I, a process which is dependent on free radical generation most probably from the mitochondria.[2]. Inhibition of Complex I is dependent on the nitrosation of a critical cysteine which is exposed during the conformational change of the enzyme from its active to its deactive form in hypoxia (A/D transition) [3]. This locks Complex I in its nitrosated state arresting its activation and affecting cellular energy production. We speculated, that hypoxic deactivation may act as a protective intrinsic mechanism against ischemia/reperfusion injury, but at the same time could initiate mitochondria-dependent pathophysiology during oxidative or nitrosative stress [3, Nitric Oxide (NO) inhibits mitochondrial cytochrome c oxidase (Complex IV) in a reversible manner and at physiological concentrations. Its affinity for NO is greater than that for oxygen (O2) suggesting that NO might regulate O2 consumption or interfere with its usage in pathological situations. For review see, [1]. We discovered that long-term inhibition of Complex IV led to persistent inhibition of complex I, a process which is dependent on free radical generation most probably from the mitochondria.[2]. Inhibition of Complex I is dependent on the nitrosation of a critical cysteine which is exposed during the conformational change of the enzyme from its active to its deactive form in hypoxia (A/D transition) [3]. This locks Complex I in its nitrosated state arresting its activation and affecting cellular energy production. We speculated, that hypoxic deactivation may act as a protective intrinsic mechanism against ischemia/reperfusion injury, but at the same time could initiate mitochondria-dependent pathophysiology during oxidative or nitrosative stress [3, 4] 1. Moncada, S. and J.D. Erusalimsky, Does nitric oxide modulate mitochondrial energy generation and apoptosis? Nat Rev Mol Cell Biol, 2002. 3(3): p. 214-20. ![]() Sir Salvador Moncada FRS, University College London
![]() Sir Salvador Moncada FRS, University College London
"Salvador Moncada, MD, obtained his PhD in 1973 at the Royal College of Surgeons in London. He then moved to the Wellcome Research Laboratories where he initiated the work leading to the discovery of the enzyme thromboxane synthase and the vasodilator prostacyclin. He was also responsible for the identification of nitric oxide as a biological mediator and the elucidation of the metabolic pathway leading to its synthesis. Since 1996 Professor Moncada has directed the Wolfson Institute for Biomedical Research at University College London. He continued his research in the areas of mitochondrial biology and cell metabolism where he made significant contributions. His current research is concentrated in the area of cell proliferation. Professor Moncada is a Fellow of the Royal Society and a Foreign Associate of the National Academy of Science of the USA and in 2010 he received a Knighthood for his services to Science."
|
Chair
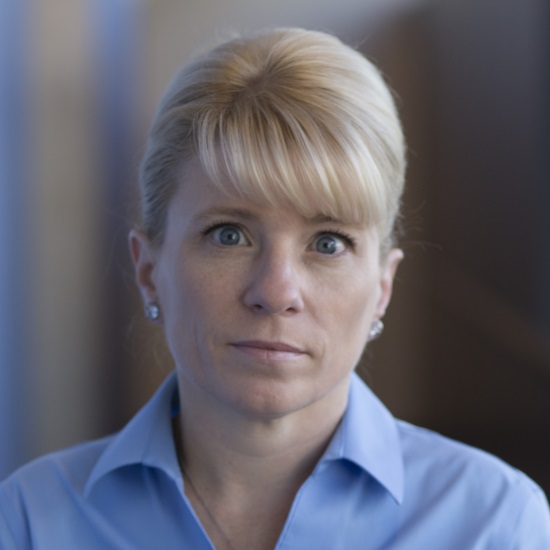
Professor Shana Kelley, University of Toronto, Canada
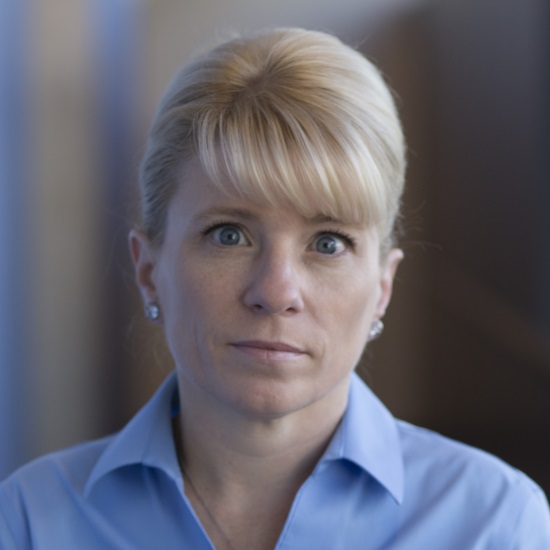
Professor Shana Kelley, University of Toronto, Canada
Dr Shana Kelley is a Distinguished Professor of Pharmaceutical Sciences, Chemistry, Biochemistry, and Biomedical Engineering at the University of Toronto. Dr Kelley received her PhD from the California Institute of Technology and was a NIH postdoctoral fellow at the Scripps Research Institute. The Kelley research group works in a variety of areas spanning bioanalytical chemistry, chemical biology and nanotechnology. Shana’s group has developed novel electrochemical sensors that enable ultrasensitive nucleic acids detection for clinical diagnostics, and has also investigated a new set of chemical probes that interact with mammalian mitochondria. Dr Kelley’s work has been recognised with a variety of distinctions, including being named one of ‘Canada’s Top 40 under 40’, a NSERC E.W.R. Steacie Fellow, the 2011 Steacie Prize, and the 2016 NSERC Brockhouse Prize. She has also been recognised with the Pittsburgh Conference Achievement Award, an Alfred P. Sloan Research Fellowship, a Camille Dreyfus Teacher-Scholar award, a NSF CAREER Award, a Dreyfus New Faculty Award, and was also named a ‘Top 100 Innovator’ by MIT’s Technology Review. She is a founder of two molecular diagnostics companies, GeneOhm Sciences (acquired by Becton Dickinson in 2005) and Xagenic Inc.
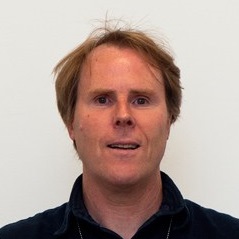
Professor Robert Lightowlers, Wellcome Trust Centre for Mitochondrial Research, Newcastle University, UK
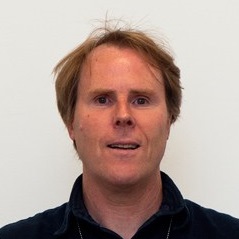
Professor Robert Lightowlers, Wellcome Trust Centre for Mitochondrial Research, Newcastle University, UK
Robert Lightowlers received his BSc (Hons) in Biological Sciences from UEA in 1983, and a PhD in Microbial Genetics from ANU Canberra in 1988. Following a postdoctoral position at the Institute of Molecular Biology in Eugene, Oregon, USA, where he gained a lifelong interest in mitochondria and mitochondrial disease, Lightowlers returned to the UK, where he established his laboratory at Newcastle University and, with Professor Doug Turnbull, the Mitochondrial Research Group (MRG). Lightowlers has been employed by Newcastle University since that date, being awarded a Personal Chair in 2000. The MRG has expanded enormously and led to formation of the Wellcome Trust Centre for Mitochondrial Research in 2012. Lightowlers was appointed Director of the Institute for Cell and Molecular Biosciences in 2012. His main research topic during this time has been into determining the molecular mechanisms that underlie expression of this genome in man and searching for a cure for these disorders.
09:00 - 09:35 |
Mitochondrial redox probes
There is currently considerable interest in elucidating the relationship between the levels of reactive oxygen species (ROS) and health and disease: while transient increases in ROS levels are necessary for physiological processes, chronically-elevated ROS levels (oxidative stress) are associated with various pathologies. In particular, mitochondrial ROS levels are known to be key to the function of the organelle and the cell. A particular challenge in imaging oxidative stress is to distinguish chronic elevations in oxidative capacity from natural perturbations that arise from signalling events. This cannot be sufficiently met by current ROS probes that are based on irreversible reactions, and we are therefore developing a new class of fluorescent probes that reversibly sense their environment. Utilising flavins as biologically-relevant, reversible redox switches, we have developed two mitochondrially-localised fluorescent redox probes, which we have been able to utilise in various biological contexts. NpFR2 is an intensity-based probe, which is colourless in reducing environments, and emits a green fluorescence under conditions of oxidative stress. In order to minimise probe concentration effects, we developed FRR1, which reports on oxidative capacity by a change in emission colour rather than intensity. We have demonstrated the applicability of these probes in flow cytometry and fluorescence lifetime imaging microscopy, as well as conventional microscopy experiments. ![]() Dr Elizabeth New, University of Sydney, Australia
![]() Dr Elizabeth New, University of Sydney, AustraliaDr Elizabeth New undertook her undergraduate and Masters studies at the University of Sydney. She completed her PhD studies in 2010 at the University of Durham with Professor David Parker. Liz was then a Royal Commission for the Exhibition of 1851 Research Fellow at the University of California, Berkeley, working with Professor Chris Chang. In 2012, she returned to the University of Sydney, holding a Discovery Early Career Research Fellowship from the Australian Research Council from 2012-2014, and a Westpac Research Fellowship from 2016. Liz’s research interests lie in the development of small molecule sensors for the study of oxidative stress and metal ions in biology. |
|
---|---|---|
09:35 - 10:10 |
Mitochondria targeted mass spec probes
Over the past few years I have been working on developing mass spectrometric approaches to assess mitochondrial function and production of reactive species in mitochondria in vivo. Typically these approaches utilise the mitochondria-targeting of compounds in vivo by the conjugation of reactive moieties to a lipophilic triphenylphosphonium cation. The reactive moieties then react within mitochondria in vivo to generate distinctive products that report on mitochondrial function. The products are then analysed ex vivo by LC-MS/MS. Here I will describe some of these approaches and the kinds of biological problem that I have been able to address. This will include assessment of mitochondrial hydrogen peroxide with MitoB, mitochondrial membrane potential with MitoClick, mitochondrial methylglyoxal by MitoG and mitochondrial superoxdie by MitoNeo. ![]() Dr Angela Logan, MRC Mitochondrial Biology Unit, Cambridge, UK
![]() Dr Angela Logan, MRC Mitochondrial Biology Unit, Cambridge, UKAngela Logan has an MSci in biochemistry from the University of Glasgow and received her PhD from the University of Cambridge in 2010 on the relationship between reactive oxygen species and oxidative damage to mitochondrial DNA. Angela has worked in a postdoctoral role in Mike Murphy’s lab since 2010 specialising in the use of mass spectrometry probes to investigate mitochondria. She oversees the running of two triple quad mass specs for analysis of these probes and has been involved in the development and implementation of several new mass spec probes, including probes to assess mitochondrial hydrogen peroxide and mitochondrial membrane potential, and expanding the use of these probes to investigate mitochondrial in various animal models. |
|
11:00 - 11:35 |
Mitochondria-targeted metformins as antitumor agents
Reports indicate that mitochondria-targeted cationic agents induce antiproliferative effects in tumor cells without markedly affecting normal cells. For example, conjugating a nitroxide, quinone, or chromanol group of tocopherol to the triphenylphosphonium (TPP+) moiety via an aliphatic linker chain selectively enhanced their antiproliferative effect in tumor cells. These effects were largely attributed to the selective uptake and retention of TPP+-containing compounds in tumor mitochondria. The objective was to enhance the efficacy of metformin, a synthetic analog of a naturally occurring biguanide. Metformin is an approved antidiabetic drug; currently, we are exploring repurposing the drug for cancer treatment. Metformin exists as a hydrophilic cation at a physiological pH and weakly targets mitochondria. It exerts biological activity through alterations of cellular bioenergetics without itself undergoing any metabolism. We hypothesized that increasing the mitochondria-targeting potential of metformin by attaching a positively charged lipophilic substituent would enhance its antitumor effect. We synthesized various metformin analogs by attaching TPP+ to metformin via different alkyl chain lengths and found that these modified analogs increasingly target mitochondria. In particular, the analog Mito-Met10, synthesized by attaching TPP+ to metformin via a 10-carbon aliphatic side chain, was nearly 1,000 times more potent than metformin at inhibiting cell proliferation in pancreatic ductal adenocarcinoma (PDAC). The enhanced potency of Mito-Met10 is attributed to the inhibition of mitochondrial complex 1 and the subsequent AMPK activation stimulated by reactive oxygen species. Mito-Met10 had relatively little or no effect in nontransformed control cells. Mito-Met10 administration more potently inhibited PDAC growth in preclinical mouse models. In my talk, I will discuss how improved mitochondrial targeting of metformin may lead to more effective therapeutic options in treating cancers including PDAC. ![]() Professor Balaraman Kalyanaraman, Medical College of Wisconsin, USA
![]() Professor Balaraman Kalyanaraman, Medical College of Wisconsin, USABalaraman Kalyanaraman received his B.S. in Chemistry from the University of Madras, India, and his M.S. from the Indian Institute of Technology, Bombay. In 1978, he received his PhD in Chemistry from the University of Alabama, Tuscaloosa, and postdoctoral training at the NIEHS in Research Triangle Park, NC. In 1981, he joined the Medical College of Wisconsin (MCW), Milwaukee, where he serves as Chairman and Professor of Biophysics. In 2000 he founded MCW’s Free Radical Research Center. He co-directs the MCW Cancer Center’s Cancer Biology program, and directs the Bioenergetics Shared Resource. He has received the International EPR Society Silver Medal for Outstanding Research in the Application of EPR in Biology and Medicine, Lifetime Achievement Award from the Society for Free Radical Biology and Medicine, and was named the Harry R. & Angeline E. Quadracci Professor in Parkinson’s Research, and an Honorary Professor of Medicine by the School of Medicine, University of the Republic in Montevideo, Uruguay. Raman has served on the editorial boards for Biochemical Journal, Free Radical Research, and Free Radical Biology and Medicine. |
|
11:35 - 12:10 |
Mitochondrial metabolites and cancer
Mutations of the tricarboxylic acid cycle (TCA cycle) enzyme fumarate hydratase (FH) cause the hereditary cancer syndrome Hereditary Leiomyomatosis and Renal Cell Cancer (HLRCC). FH-deficient renal cancers are highly aggressive and metastasise even when small, leading to an abysmal clinical outcome. The link between loss of FH and tumour formation is still under intense investigation. Evidence suggests that fumarate, a small molecule metabolite that accumulates in FH-deficient cells, may contribute to tumorigenesis in HLRCC. For instance, accumulation of fumarate has been associated with the stabilisation of the Hypoxia Inducible Factors HIFs and to the activation of the antioxidant master regulator NRF2, via succination of its negative regulator Keap1. However, the contribution of these signalling cascades to tumorigenesis of HLRCC has been debated and the oncogenic role of fumarate still unclear. Here we used a multidisciplinary approach to investigate the consequences of the loss of FH and present evidence that FH-deficient cells undergo a fumarate-dependent epithelial-to-mesenchymal-transition, a phenotypic switch associated with cancer initiation, invasion, and metastasis. We propose that this phenotypic switch might prime cell to transformation and contribute to the tumorigenesis and metastatisation of FH-deficient cancers. ![]() Dr Christian Frezza, MRC Cancer Unit, University of Cambridge, UK
![]() Dr Christian Frezza, MRC Cancer Unit, University of Cambridge, UKChristian Frezza is an MRC Programme Leader at the MRC Cancer Unit in Cambridge. He studied Medicinal Chemistry at the University of Padova, Italy, and gained his MSc in 2002, after a period of research on mitochondrial toxicity induced by photoactivable anticancer drugs. Christian then joined the laboratory of Luca Scorrano in Padova to start a PhD on mitochondrial dynamics and apoptosis. In 2008 he moved to the Beatson Institute of Cancer Research in Glasgow, as a recipient of an EMBO Long Term Fellowship, where he investigated the role of mitochondrial defects in tumorigenesis. Christian’s research is focussed on the understanding of cellular metabolism and the intricacies of the process of cellular transformation, particularly focusing on the role of small molecules metabolite in tumorigenesis. His major goal now is to exploit this biochemical knowledge to pioneer novel therapeutic strategies for cancer. |
Chair
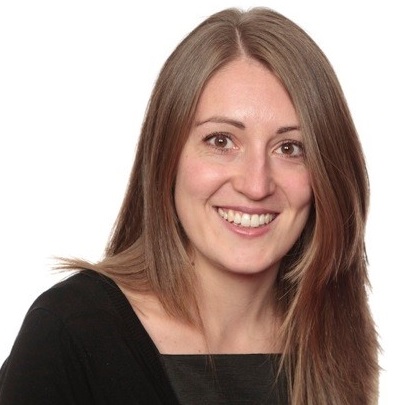
Dr Helena Cochemé, MRC Clinical Sciences Centre, UK
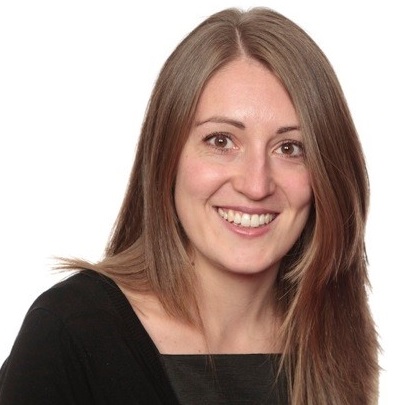
Dr Helena Cochemé, MRC Clinical Sciences Centre, UK
Helena Cochemé graduated with a BSc in Biology from Imperial College London in 2001, and a PhD in Biochemisty from the University of Cambridge in 2006, based at the MRC Mitochondrial Biology Unit (then called the MRC Dunn Human Nutrition Unit) in the group of Mike Murphy, where she developed an interest in mitochondrial oxidative stress, redox biology and ROS probes. Following a post-doc at the Institute of Healthy Ageing, University College London in the laboratory of Linda Partridge from 2007-2013, she was appointed a Group Leader at the MRC Clinical Sciences Centre, London in 2013. Her research uses the fruit fly Drosophila as an in vivo model system to investigate the role of redox signalling in stress responses, metabolic disease, and ageing.
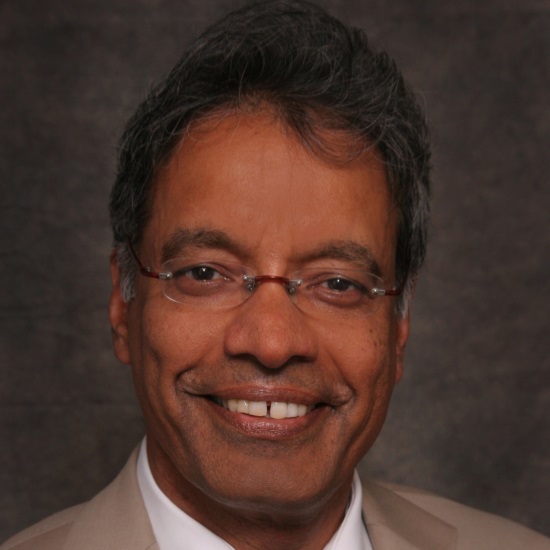
Professor Balaraman Kalyanaraman, Medical College of Wisconsin, USA
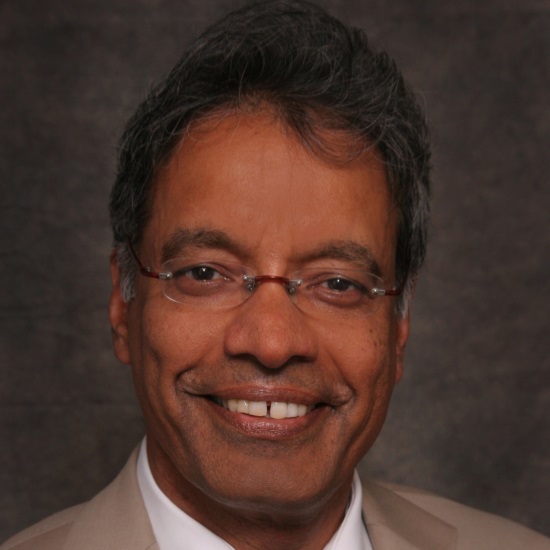
Professor Balaraman Kalyanaraman, Medical College of Wisconsin, USA
Balaraman Kalyanaraman received his B.S. in Chemistry from the University of Madras, India, and his M.S. from the Indian Institute of Technology, Bombay. In 1978, he received his PhD in Chemistry from the University of Alabama, Tuscaloosa, and postdoctoral training at the NIEHS in Research Triangle Park, NC. In 1981, he joined the Medical College of Wisconsin (MCW), Milwaukee, where he serves as Chairman and Professor of Biophysics. In 2000 he founded MCW’s Free Radical Research Center. He co-directs the MCW Cancer Center’s Cancer Biology program, and directs the Bioenergetics Shared Resource. He has received the International EPR Society Silver Medal for Outstanding Research in the Application of EPR in Biology and Medicine, Lifetime Achievement Award from the Society for Free Radical Biology and Medicine, and was named the Harry R. & Angeline E. Quadracci Professor in Parkinson’s Research, and an Honorary Professor of Medicine by the School of Medicine, University of the Republic in Montevideo, Uruguay. Raman has served on the editorial boards for Biochemical Journal, Free Radical Research, and Free Radical Biology and Medicine.
13:30 - 14:05 |
Molecular probes for assessing mitochondrial function
Mitochondrial dysfunction is involved in a wide range of diseases and implicated in the process of ageing itself. Designer small molecules can help elucidate the mitochondrial processes involved in a way that is complementary to molecular biology. The lecture will include examples of: Exomarkers for the quantification of reactive species in the mitochondria of whole living organisms. Such exomarkers are reporters of specific endogenous reactive species, generated from exogenously added molecular probes, which are targeted to the mitochondria. We have developed molecular probes that report on a variety of mitochondrial reactive species including hydrogen peroxide, superoxide, and hydrogen sulfide. Simple modulators of mitochondrial function. These include molecules that protect mitochondria and are potential drugs, but also mitotoxics. The latter increase the amount of specific endogenous reactive species in the mitochondria, so that the cellular response can be investigated and theories of ageing, cardiovascular disease and neurodegeneration tested. Photoactivatable probes for spatial and temporal control or labeling of mitochondria. These include those designed to track mitochondrial movement, switch off mitochondrial function, or release a drug in response to light. Smart molecules that respond to the mitochondrial environment, including those that incorporate a negative feedback loop. Mitochondrial targeting is ensured by the incorporation of a triphenylphosphonium (TPP) group. ![]() Professor Richard Hartley, University of Glasgow, UK
![]() Professor Richard Hartley, University of Glasgow, UKRichard Hartley graduated with a 1st in Natural Sciences from the University of Cambridge in 1988. He carried out his PhD in chemical synthesis in Cambridge under the direction of Dr Stuart Warren, and then moved to Canada in 1991 to work with Professor T. H. Chan at McGill University, Montreal. He took up his first academic post as a Newman Scholar in University College Dublin in 1994, and became a Lecturer in Bioorganic Chemistry in Glasgow in 1995. He has risen through the ranks at the same institution and is now Professor of Chemical Biology. Professor Hartley develops small molecular probes for elucidating biological processes both on a cellular and a whole organism level. His current work focuses on mitochondria. His particular successes have been the development of probes to produce exomarkers, potential therapeutics for diseases involving mitochondrial dysregulation, and photoactivatable probes that allow control of mitochondrial activity. |
|
---|---|---|
14:05 - 14:40 |
The mother of invention in thiol redox proteomics
A variety of redox modifications and switches, including S-oxidation (sulfenylation and sulfinylation) converge within mitochondria, but they are challenging to detect inside living systems. We present the design, synthesis, and biological applications of Mitochondria 2,4-Piperidinedione-1 (MitoPD1), a new type of bifunctional probe for trapping and tagging sulfenylated mitochondrial proteins. MitoPD1 combines a chemoselective C-nucleophile and a mitochondrial targeting phosphonium moiety for detection of mitochondrial S-sulfenylation. MitoPD1 is rapidly accumulated by energized mitochondria and can be used to visualize S-sulfenylated proteins by Western Blot using an antibody against the TPP moiety. Fractionation of mitochondria into membrane and matrix fractions after they had been incubated with MitoPD1 indicated that the majority of labeling occurs in the matrix and matrix-facing membrane proteins. These and additional data will be presented at the upcoming Chemical Biology Approaches to Assessing and Modulating Mitochondria meeting in September. ![]() Dr Kate Carroll, The Scripps Research Institute, Florida, USA
![]() Dr Kate Carroll, The Scripps Research Institute, Florida, USAKate S. Carroll is an Associate Professor with tenure in the Department of Chemistry at The Scripps Research Institute in Jupiter, Florida. She received her BA degree in Biochemistry from Mills College in 1996 and PhD in Biochemistry from Stanford University in 2003. Her postdoctoral work was completed at the University of California, Berkeley, where she was a Damon Runyon-Walter Winchell Chancer Fund Fellow with Professor Carolyn Bertozzi. She was an Assistant Professor at the University of Michigan until 2010, when she joined the Chemistry faculty at Scripps. Dr Carroll is a permanent member of the Synthetic and Biological Chemistry NIH Study Section A and currently serves on the editorial board of Cell Chemistry and Biology, Molecular Biosystems, FASEB Journal, The Journal of Biology Chemistry, and is a contributing member of ‘Faculty of 1000’. She is also the recipient of the ACS Pfizer Award in Enzyme Chemistry (2013), Camille Dreyfus Teacher-Scholar Award (2010), Scientist Development Award from American Heart Association (2008), and Special Fellow Award from the Leukemia and Lymphoma Society (2006). Research interests: Professor Carroll’s research interests span the disciplines of chemistry and biology with an emphasis on studies of sulphur biochemistry pertinent to disease states. Her lab focuses on the development of novel tools to study redox modifications of cysteine thiols, profiling changes in protein oxidation associated with disease, and exploiting this information for development of diagnostic and therapeutic approaches. In addition, her group investigates sulfur metabolism pathways that are essential for infection and long-term survival of human pathogens, such as Mycobacterium tuberculosis. |
|
15:30 - 16:05 |
Mitochondrial disease treatment: the Saga of KH176
In recent years, there has been substantial progress on many fronts in our understanding of diseases affecting the mitochondrial energy generating OXPHOS system. Model systems using lower eukaryotes and comparative studies have provided invaluable insights into the many aspects of mitochondrial biology. The interplay between the mitochondrial and nuclear genomes in OXPHOS function is also better understood, and there is now an extensive collection of ever increasing genetic defects that have been described in both genomes of patients with OXPHOS defects. More and more mouse models of mitochondrial diseases have been created. Various new therapeutic intervention strategies, ranging from gene therapy towards small molecules, are under development. Some still at the level individual cells others in early clinical trial stages. I will review the state of the art of our strategy to develop small molecule therapies for mitochondrial disease with KH176 as the prototypic example. ![]() Professor Jan Smeitink, Radboud University Nijmegen, The Netherlands
![]() Professor Jan Smeitink, Radboud University Nijmegen, The NetherlandsJan Smeitink obtained his training in Paediatrics at the Radboud university medical center, Nijmegen, The Netherlands. He completed his training in the field of Inborn Errors of Metabolism at the Wilhelmina Children’s Hospital, Utrecht, The Netherlands. He has been Head of the Department of Metabolic Diseases at the Radboud university medical center since 1996, and has a special interest in the study of mitochondria in health and disease. Jan Smeitink and his team developed clinical scoring systems, described different new phenotypes of patients harbouring mitochondrial disorders, elucidated most of the nuclear complex I genes, described the first mutations in these genes, described the first complex I assembly pathway and detected new assembly genes and mutations. They found the first translation elongation defect, studied the cell biological consequences of oxidative phosphorylation disorders, in particular complex I. They developed numerous new assays. Current focus is on drug development for which Smeitink established Khondrion BV, a mitochondrial medicine company. |
|
16:05 - 17:00 |
Panel discussion: future challenges and opportunities
![]() Professor Mike Murphy FMedSci, University of Cambridge
![]() Professor Mike Murphy FMedSci, University of CambridgeMike Murphy received his BA in chemistry at Trinity College, Dublin in 1984 and his PhD in Biochemistry at Cambridge University in 1987. After stints in the USA, Zimbabwe, and Ireland he took up a faculty position in the Biochemistry Department at the University of Otago, Dunedin, New Zealand in 1992. In 2001 he moved to the MRC Mitochondrial Biology Unit in Cambridge, UK (then called the MRC Dunn Human Nutrition Unit) where he is a programme leader. Murphy's research focuses on the roles of reactive oxygen species in mitochondrial function and pathology. In particular he has pioneered the targeting of bioactive and probe molecules to mitochondria in vivo. Murphy is Professor of Mitochondrial Redox Biology at the University of Cambridge, a Wellcome Trust Investigator, honorary research Professor at the University of Otago, New Zealand, a recipient of the Keilin Medal from the Biochemical Society, an honorary Fellow of the Royal Society of New Zealand and a Fellow of the Academy of Medical Sciences (FMedSci). |