Links to external sources may no longer work as intended. The content may not represent the latest thinking in this area or the Society’s current position on the topic.
Enhancing photosynthesis in crop plants: targets for improvement
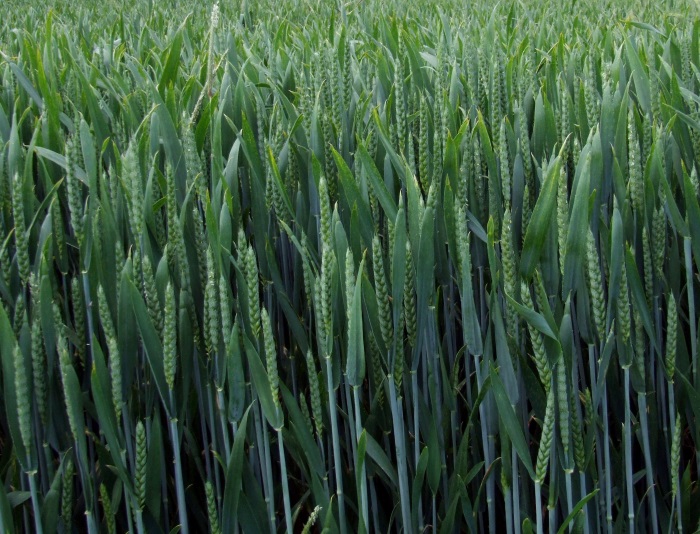
Scientific discussion meeting organised by Professor Christine Foyer, Professor Alexander Ruban, Professor Peter Nixon and Professor Alfred William Rutherford FRS.
Photosynthesis is the major driver of life on earth. It can provide novel solutions for crop improvement in a changing climate, if we consolidate information concerning functional operation, current and future limitations, impacts on stress/growth responses and potential targets and markers. This forum seeks to identify new knowledge and novel concepts in order to facilitate the transition from basic to applied knowledge.
Recorded audio of the presentations will be available below. The meeting papers are now available in Philosophical Transactions of the Royal Society B.
Schedule
Chair
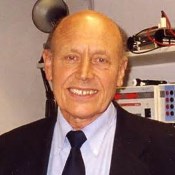
Professor James Barber FRS, Imperial College London, UK
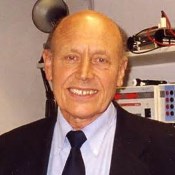
Professor James Barber FRS, Imperial College London, UK
James Barber is Emeritus Ernst Chain Professor of Biochemistry, Senior Research Fellow at Imperial College London and the Cannon Visiting Professor to Nanyang Technological University (NTU) in Singapore. He is a Fellow of the Royal Society (FRS), Fellow of the Royal Society of Chemistry (FRSC), Member of European Academy and Foreign Member of the Swedish Royal Academy of Sciences. He has Honorary Doctorates of Stockholm University, University of East Anglia and NTU. He has been awarded several medals and prizes including Flintoff Medal of RSC, Novartis Medal (UK Biochem. Soc), Wheland Medal (Univ. of Chicago), Eni-Ital gas/ ENI Prize, Interdisciplinary Prize Medal of the RSC, Porter Medal of the International Photochemical Societies (Europe, USA and Asia) and the Communication Award of the International Society of Photosynthesis Research. In 2009 he was the Lee Kuan Yew Distinguished Visitor to Singapore. Much of his research has focused on PSII and the water splitting process that it catalyses and obtained its crystal structure in 2004. He is now investigating inorganic systems to mimic PSII in order develop technology for non-polluting solar fuels.
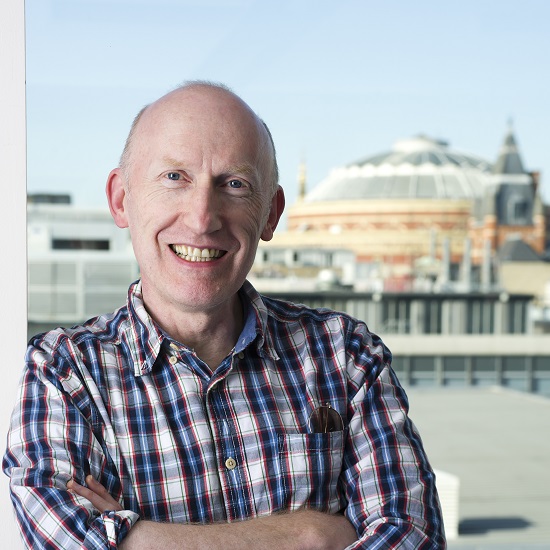
Professor A William Rutherford FRS, Imperial College London, UK
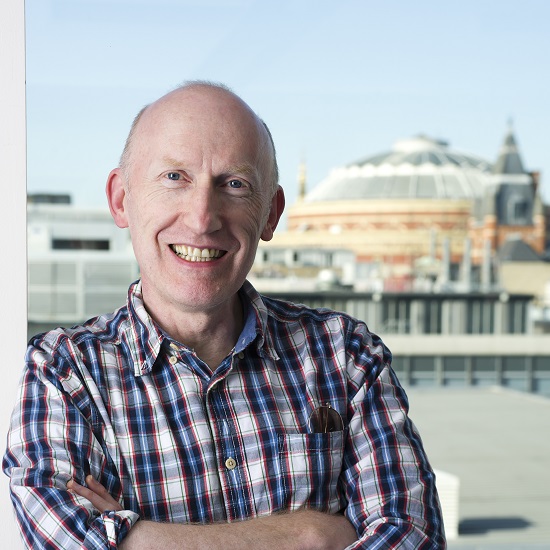
Professor A William Rutherford FRS, Imperial College London, UK
Bill Rutherford uses physico-chemical methods to understand photosynthesis. He has particular interests in the bioenergetics and evolution of photosynthetic electron transfer and water oxidation. Having studied biochemistry at the University of Liverpool, his PhD at UCL involved biophysics of bacterial photosynthesis. As a post doc at the University of Illinois, he moved into oxygenic photosynthesis, discovering the excited triplet state of chlorophyll formed by radical-pair recombination. This state causes photo-oxidative stress that can limit plant growth, bleach coral, etc. As a post doc in Riken, Japan, he determined the origin of thermoluminescence in oxygenic photosynthesis, establishing it as research method. His work was central in establishing the common evolutionary origins of all the photosynthetic reaction centres. He was a CNRS researcher in Saclay, near Paris, before moving to Imperial College London in 2011, where he holds the Chair of Biochemistry of Solar Energy.
09:05 - 09:30 |
Understanding energy limitations in oxygenic photosynthesis
Aerobic biological electron transfer systems avoid side-reactions with O2 by redox tuning. Two physico-chemical numbers are key to these processes: i) -160mV, the redox midpoint potential of the O2/O2-• couple, determining the favourability of superoxide formation; and ii) ~1eV, the amount of energy needed to convert 3O2, molecular oxygen, to its highly reactive and damaging singlet form, 1O2. The occurrence of anomalously energy-inefficient reactions in aerobic bioenergetics can be understood in these terms. Protective and regulatory mechanisms can also be rationalised within this model (including an unexpected new sink-to-source regulatory mechanism in photosynthesis, which I will present). From this perspective Photosystem II (PSII) is “energy squeezed”, having insufficient energy in the 680nm photon to be able to achieve i) high quantum yield charge separation, ii) PSII chemistry (reduction of quinone and oxidation of water), and iii) the appropriate driving forces (over-potentials), while at the same time avoiding 1O2-mediated photodamage from back-reactions. PSII is particularly susceptible to photodamage since it must interface 1-photon-per-electron photochemistry with its multi-electron catalytic chemistry, inevitably generating intermediate states that back-react (i.e. S2, S3, QA- and QB-). This “energy squeeze” may set the “red-limit” for oxygenic photosynthesis. The existence of long-wavelength Chld-containing PSII in Acaryochloris appears to contradict this suggestion. We have suggested however that this improvement in efficiency is only possible due a decrease in the “energy headroom”, that part of the over-potential needed to deal with fluctuations in the environment (light intensity): i.e. efficiency gains are paid for by loss of resilience. If so, extending the spectrum of oxygenic photosynthesis to longer wavelengths will encounter problems. We are currently studying long-wavelength species to test these predictions. ![]() Professor A William Rutherford FRS, Imperial College London, UK
![]() Professor A William Rutherford FRS, Imperial College London, UKBill Rutherford uses physico-chemical methods to understand photosynthesis. He has particular interests in the bioenergetics and evolution of photosynthetic electron transfer and water oxidation. Having studied biochemistry at the University of Liverpool, his PhD at UCL involved biophysics of bacterial photosynthesis. As a post doc at the University of Illinois, he moved into oxygenic photosynthesis, discovering the excited triplet state of chlorophyll formed by radical-pair recombination. This state causes photo-oxidative stress that can limit plant growth, bleach coral, etc. As a post doc in Riken, Japan, he determined the origin of thermoluminescence in oxygenic photosynthesis, establishing it as research method. His work was central in establishing the common evolutionary origins of all the photosynthetic reaction centres. He was a CNRS researcher in Saclay, near Paris, before moving to Imperial College London in 2011, where he holds the Chair of Biochemistry of Solar Energy. |
|
---|---|---|
09:30 - 10:00 |
Why is photosynthesis in nature so inefficient?
Over the past two decades, together with several colleagues, I have used remotely sensed observations of oceanic chlorophyll and analogous observations of terrestrial plants to derive global production of the planet. Simultaneously, we build and deployed dozens of high resolution fluorometers that measured the quantum efficiency of photosystem II across the world oceans from changes in the amplitude of variable fluorescence. Over the past ca. 5 years we attempted to close the budget on the absorbed solar radiation absorbed by phytoplankton. To this end, we built and deployed picosecond lifetime based instruments. From tens of thousands of paired measurements of variable fluorescence amplitudes and lifetimes, we conclude that in the real world oceans only ~35% of absorbed solar photons are used for photochemistry, ~7% are dissipated as fluorescence, and the remainding ~58% are dissipated as heat. The exceptionally high loss of absorbed solar radiation that is dissipated as heat appears to be a consequence of nutrient limitation of photosynthetic processes. Two nutrients are especially identified: dissolved inorganic nitrogen and soluble iron. The data suggest that these nutrients, which can be episodically supplied by turbulent mixing events (e.g., storms) allow for very rapid repair of the PSII reaction centers. I will discuss how these processes in phytoplankton potentially allow for pathways to enhance photosynthesis in crop plants. ![]() Professor Paul Falkowski, Rutgers University, USA
![]() Professor Paul Falkowski, Rutgers University, USAPaul G. Falkowski is the Bennett L. Smith Professor and director of the Environmental Biophysics and Molecular Ecology Program at Rutgers University. His scientific interests include evolution of the Earth systems, paleoecology, photosynthesis, biophysics, biogeochemical cycles, and symbiosis. Falkowski earned his B.S. and M.Sc. degrees from the City College of the City University of New York and his Ph.D. from the University of British Columbia. After a post-doctoral fellowship at the University of Rhode Island, he joined Brookhaven National Laboratory in 1976 as a scientist in the newly formed Oceanographic Sciences Division. He moved to Rutgers University in 1998. He works on global photosynthesis, especially in the oceans. He is a member of United States National Academy of Sciences. Falkowski lives in Princeton, New Jersey, with his wife, Sari Ruskin. |
|
11:00 - 11:30 |
Functions of chloroplast proteins in stress signalling
Signalling between the chloroplasts and the nucleus is required to maintain photosynthesis and other functions. The regulated expression of nuclear genes by chloroplast signals is known as retrograde signalling. Redox signals arising in the chloroplast are considered to be an important in retrograde signalling but the mechanisms involved remain poorly defined. Proteins such as WHIRLY1, which is a ssDNA-binding protein localised in the chloroplasts and nuclei, have the potential to fulfil redox signalling functions. WHIRLY1 is required for plastid genome stability and plastid gene transcription. We have characterised WHIRLY1 functions in barley using RNAi-knockdown lines (W1-1, W1-7 and W1-9) that have very low levels of HvWHIRLY1 transcripts. Leaves of the WHIRLY1-deficient plants establish photosynthesis more slowly than the wild type, but otherwise are similar to the wild type, Photosynthesis rates were similar in all lines but W1-1, W1-7 and W1-9 leaves had significantly more chlorophyll and less sucrose than the wild type. Transcripts encoding specific sub-sets of chloroplast-localised proteins such as ribosomal proteins, subunits of the RNA polymerase and the thylakoid NADH and cytochrome b6/f complexes were much more abundant in the W1-7 leaves than the wild type. The roles of WHIRLY1 in the acclimation of photosynthesis to stress will be discussed, together with possible retrograde signalling mechanisms. ![]() Professor Christine Foyer, Centre of Plant Sciences, University of Leeds, UK
![]() Professor Christine Foyer, Centre of Plant Sciences, University of Leeds, UKChristine Helen Foyer is the Professor of Plant Sciences at the University of Leeds, Leeds, UK. She is also a Winthrop Professor at The University of Western Australia, Perth, Australia and a Pao Yu-Kong Chair Professor of Zhejiang University, Hangzhou, China. She is a member of the French Academy of Agriculture and the Secretary General of the Federation of European Plant Biologists. She is member of BBSRCCommittee B and the BBSRC (UK) Pool of Experts. She is an Annals of Botany Company Board Member, an Associate Editor for Plant, Cell and Environment and on the Editorial Boards of PhysiologiaPlantarum, Journal of Experimental Botany and Frontiers in Plant Sciences. She is a Scientific Advisory Board member forthe Helmholtz Center Munich. Her current H-Index is 83. Her research interests concern how primary processes (photosynthesis, respiration) alter the reduction/oxidation (redox) status of the cell and influence plant growth and defence responses to stresses such as drought, chilling, high light and aphid infestation. |
|
11:30 - 12:00 |
Strategies and tools to improve crop productivity by targeting photosynthesis
Crop productivity needs to substantially increase to meet global food and feed demand of a rapidly growing world population. Agricultural technology developers are pursuing a variety of approaches based on both traditional technologies like genetic improvement, pest control and mechanization as well as new technologies like genomics, gene manipulation and environmental modeling to develop crops that are capable of meeting growing demand. Photosynthesis is a key biochemical process that many suggest is not yet optimized for industrial agriculture or the modern global environment. We are interested in identifying control points in maize photoassimilation that are amenable to gene manipulation to improve overall productivity. Our approach encompasses: developing and using novel gene discovery techniques, translating our discoveries into traits, and evaluating each trait in a stepwise manner that reflects a modern production environment. Our aim is to provide step change advancement in overall crop productivity and deliver this new technology into the hands of growers. ![]() Dr Michael Nuccio, Syngenta Crop Protection, LLC, USA
![]() Dr Michael Nuccio, Syngenta Crop Protection, LLC, USAI completed a B.S. in Biochemistry and a Ph.D. in Plant Biology at Texas A&M University. I did Post-Doctoral work in Plant Metabolic Biochemistry with Andrew Hanson at the University of Florida before joining Novartis (now Syngenta) in 2000 as a staff scientist. During my time with Syngenta I’ve had a number of technical, functional and project management roles. I am currently a Principle Research Scientist focused on trait discovery. My primary responsibility is applied genetic engineering to develop water optimization and yield enhancement technology for field crops. My work includes developing trait expression control tools and computational applications to facilitate trait discovery. Over the last five years I have also been responsible for identifying and securing research collaborations to support the company’s Agronomic Trait development objectives. My professional work produced several peer-reviewed publications, patents and patent applications. |
Chair
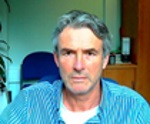
Professor Neil Hunter FRS, University of Sheffield, UK
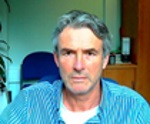
Professor Neil Hunter FRS, University of Sheffield, UK
Professor Neil Hunter FRS, Krebs Professor of Biochemistry, University of Sheffield. Born 1954, Yorkshire, U.K. B.Sc, Leicester University 1975, Ph.D, Bristol University 1978. Postdoctoral fellowships at Rutgers University and Bristol University. Lecturer at Imperial College London 1984-88, Sheffield University 1988-present.
Memberships, fellowships, honorary degrees. Biochemical Society, Society of General Microbiology, Biophysical Society, American Chemical Society; Charles and Joanna Busch Postdoctoral Fellowship, 1978; SRC Postdoctoral Fellowship, 1980; D.Sc, Bristol University, 1996; Elected to the Fellowship of the Royal Society in 2009; Honorary Professor, Qinqdao Institute of Bioenergy and Bioprocess Technology 2012; Visiting Professorship, Chinese Academy of Sciences, 2012, Honorary Professor, Shanghai JiaoTong University. European Research Council Advanced Award 2014-19.
Research Activities. Neil Hunter investigates the molecular genetics, biochemistry and biophysics of photosynthesis. The biosynthesis of carotenoid and chlorophyll pigments; the assembly of pigments and apoproteins into light harvesting and reaction centre pigment-protein complexes; the structure, function and membrane organisation of these complexes and their integrated roles in energy harvesting and trapping; the surface nanofabrication of functional photosystem arrays.
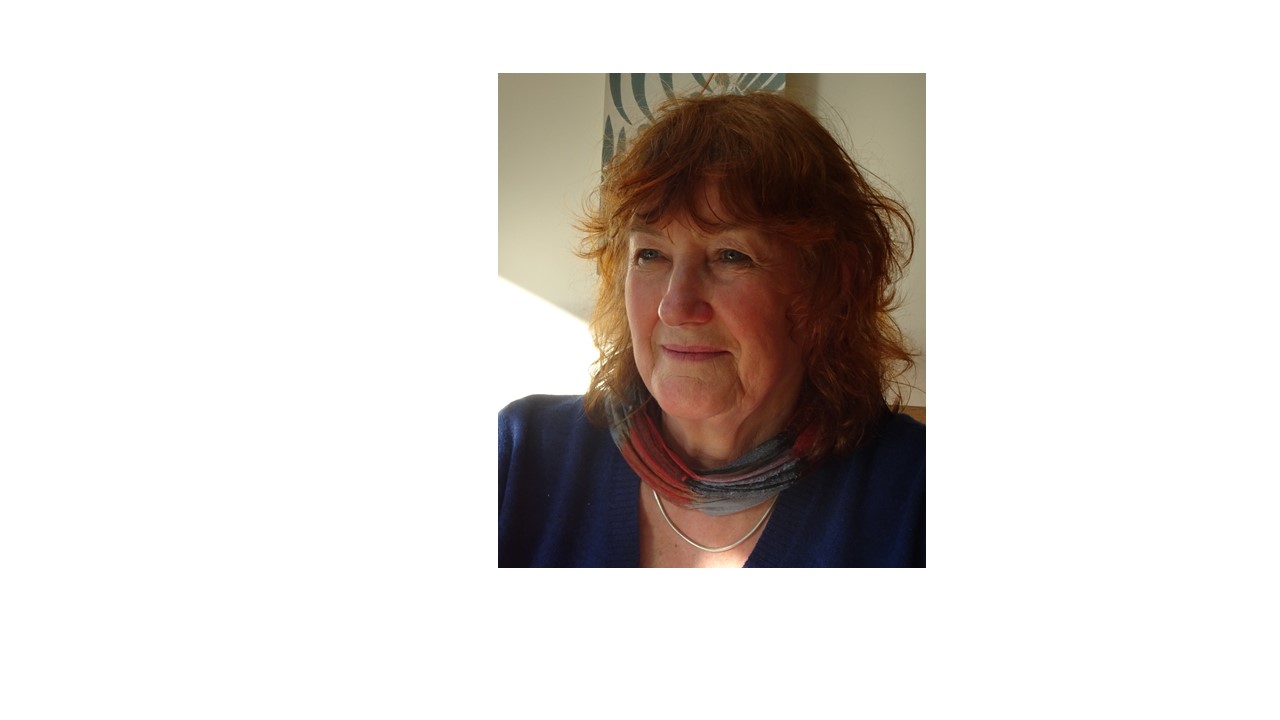
Dr Alison Telfer, Imperial College London, UK
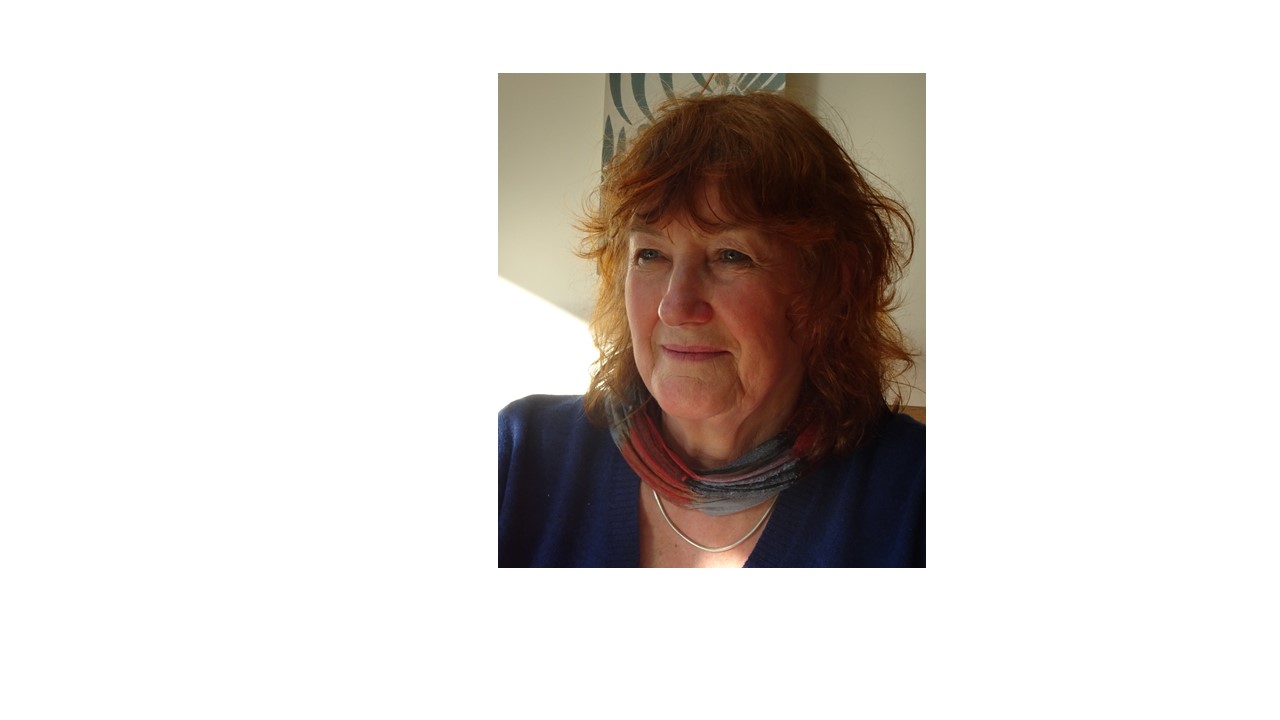
Dr Alison Telfer, Imperial College London, UK
After studying Botany at the University of Oxford, Alison Telfer received her PhD at London University, King’s College, in Plant Biochemistry specialising in photosynthesis, which has been her research topic ever since. She has been at Imperial College London as a Research Assistant with Professor Jim Barber since 1972 and is currently an Honorary Research Fellow in the Department of Life Sciences, collaborating with Professors Bill Rutherford and Peter Nixon. She is a bench scientist and has specialised in chlorophyll fluorescence measurements, adaptation to changes in light quality and quantity and spectroscopic studies of photosystem two. She has specialised in photoprotection mechanisms and direct measurement of singlet oxygen, which has recently been demonstrated to be an important signalling molecule in plants. In more recent year I have begun to study cyanobacteria with red-shifted chlorophylls, i and f, and the role of these chlorophylls in electron transfer and energy conservation.
13:30 - 14:00 |
The quantum design of photosynthesis
In photosynthesis absorbed sun light produces collective excitations (excitons) that form a coherent superposition of electronic and vibrational states of the individual pigments. Two-dimensional (2D) electronic spectroscopy allows a visualization of how these coherences are involved in the primary processes of energy and charge transfer. Based on quantitative modeling we identify the exciton-vibrational coherences observed in 2D photon echo of the photosystem II reaction center (PSII-RC). We find that the vibrations resonant with the exciton splittings can modify the delocalization of the exciton states and produce additional states, thus promoting directed energy transfer and allowing a switch between the two charge separation pathways. We conclude that the coincidence of the frequencies of the most intense vibrations with the splittings within the manifold of exciton and charge-transfer states in the PSII-RC is not occurring by chance, but reflects a fundamental principle of how energy conversion in photosynthesis was optimized. ![]() Professor Dr Rienk van Grondelle, VU University, Amsterdam, Netherlands
![]() Professor Dr Rienk van Grondelle, VU University, Amsterdam, NetherlandsRienk van Grondelle studied physics at the VU University.He obtained his PhD under the supervision of Prof. Dr. Lou Duysens. In 1982 he returned to the VU University, in 1987 he was appointed full professor. There he has built a large group studying the early events in photosynthesis. RvG has made major contributions to elucidate the fundamental physical mechanisms that underlie light harvesting and charge separation. He has developed theoretical tools to understand complex spectroscopic data. Using multi-dimensional electronic spectroscopy he recently showed that ultrafast charge separation is driven by specific molecular vibrations that allow electronic coherences to stay alive. He proposed a molecular model for photoprotection and demonstrated that the major plant light harvesting complex operates as a nanoswitch, controlled by its biological environment. These results, of utmost importance for our understanding of photosynthesis, inspire technological solutions for artificial and/or redesigned photosynthesis, as a route towards sustainable energy production. |
|
---|---|---|
14:00 - 14:30 |
Quantifying photoprotection
Non-photochemical chlorophyll fluorescence quenching (NPQ) is broadly considered to reflect the major mechanism of rapid regulation of light harvesting which defends the photosystem II reaction center (RCII) against photodamage that leads to the photoinhibition of photosynthesis. Whilst there is a great deal of the knowledge concerning the elements that trigger, tune and actually cause the quenching, little is known about its protective efficiency, the critical light intensity that is ‘safe’ for the photosynthetic organism to live given that it has a capacity for a certain level of NPQ. A newly-developed methodology aimed at radically changing our understanding of the effectiveness of the NPQ process, by quantifying its photoprotective potential, will be presented. The technique is essential if we are to fully understand the trade-offs between the metabolic costs of photoinhibition and the reduction in quantum yield caused by engaging NPQ. In this approach the value of photochemical quenching (qP) measured in the dark following illumination enables monitoring of the state of active PSII reaction centres in order to detect the early signs of photoinhibition. The method allows for the determination of the amplitude of photoprotective NPQ (pNPQ) and its potential to protect against photoinhibition. It also allows accurate quantification of the relationship between the protective component of pNPQ and actinic light intensity. This in turn makes possible the estimation of the maximum light intensity tolerated by the PSII reaction centers in a plant population, the photoptotective effectiveness of NPQ in plants with different levels of PsbS protein or zeaxanthin, and the fraction of captured energy that may be unnecessarily, or ‘wastefully’, dissipated. ![]() Professor Alexander V. Ruban, Queen Mary University London, UK
![]() Professor Alexander V. Ruban, Queen Mary University London, UKAlexander Ruban is a Professor in Biophysics at Queen Mary University of London and holds a 'Professeur des Universites (Biochimie et biologie moleculaire)' title awarded by the French Ministry of Education. He accomplished his undergraduate and masters studies at the Kiev State University (Ukraine) and obtained First Class Honours in Biophysics. He received his PhD at the Institute of Photobiology at the Academy of Sciences of Belarus. Since then Prof Ruban has been working at the Institute of Plant Physiology in Ukraine and later at the University of Sheffield in Prof Peter Horton’s laboratory. From 2006 he moved to Queen Mary Univerity of London. His research interests are focused on the photosynthetic light harvesting and mechanisms of protection against light stress in plants and algae. Specific research interests are the role of the membrane macrostructure in the photosynthetic adaptation, study of properties of carotenoids and their role in modulation of membrane protein conformation and functions. He contributed to the fundamental understanding of the molecular design of the photosynthetic light harvesting machinery introducing the concept of light adaptation 'memory' via the allosteric action of the xanthophyll cycle. |
|
15:30 - 16:00 |
The photosystem II repair cycle as a target for enhancing photosynthesis
Exposure of plants to excessive light intensities, particularly in combination with other abiotic stresses, such as elevated temperature and lack of water, causes a decline in the maximum rate of photosynthesis leading to a state of chronic photoinhibition. It is now well established that the oxygen-evolving photosystem II (PSII) complex of the thylakoid membrane is a primary target of light damage and that the D1 reaction centre polypeptide is most prone to irreversible damage. In vivo damaged PSII complexes can be efficiently repaired via the so-called PSII repair cycle which involves partial disassembly of the inactivated complex, proteolytic degradation of the damaged subunit and specific replacement by a newly synthesized copy followed by reassembly of the active complex. However, when the rate of PSII repair is unable to keep up with the rate of damage, there is a net loss of oxygen-evolving PSII complexes and decline of photosynthetic activity. Many of the proteases and accessory factors involved in the PSII repair cycle have now been identified and substantial progress has been made in understanding their function at the molecular level. In this talk, I will summarise the current view of PSII repair in cyanobacteria and chloroplasts and discuss the feasibility of improving the robustness of photosynthesis in crop plants at the level of the PSII repair cycle. ![]() Professor Peter J Nixon, Imperial College London, UK
![]() Professor Peter J Nixon, Imperial College London, UKPeter Nixon is currently a Professor of Biochemistry within the Department of Life Sciences at Imperial College London and a visiting Professor within the School of Biological Sciences at Nanyang Technological University, Singapore. His research focuses on the assembly and maintenance of the photosynthetic apparatus in the thylakoid membrane and more recently the use of synthetic biology approaches to develop cyanobacterial and chloroplasts as ‘solar biorefineries’ for the production of high-value products. He is co-convenor of the Photosynthesis and Metabolism Group of the Society of Experimental Biology. |
|
16:00 - 16:30 |
Biochemical characterisation and physiological role of the plastid terminal oxidase PTOX
Protein levels of the plastid terminal oxidase PTOX increase upon abiotic stress. PTOX may protect the photosynthetic apparatus when electron transport is limited. However, overexpression of PTOX in Nicotiana tabacum, increased the production of reactive oxygen species and exacerbated photoinhibition. The active site of PTOX comprises a non-heme diiron centre that catalyses the oxidation of plastoquinol and the reduction of O2 to H2O. We have performed for the first time a biochemical characterization of purified PTOX. The activity of PTOX was determined to be much higher than what had been previously estimated from chlorophyll fluorescence. The main reaction of PTOX is the reduction of O2 to H2O but PTOX generates O2•- in a side reaction in a substrate- and pH-dependent manner. PTOX activity in planta was investigated using Nicotiana tabacum expressing PTOX1 from Chlamydomonas reinhardtii. PTOX competed efficiently with photosynthetic electron flow. High CO2 concentrations inactivated PTOX most likely because of an acidification of the stroma. Immunoblots showed that PTOX detached from the membrane in dark-adapted leaves or in the presence of uncouplers. A model is proposed in which the membrane association of PTOX is controlled by stromal pH. When the stromal pH is neutral, PTOX exists as a soluble form and is enzymatically inactive avoiding the interference of PTOX with linear electron flow. When the stromal pH is alkaline and the photosynthetic electron chain is highly reduced under stress conditions, PTOX binds to the membrane, has access to its substrate and can serve as safety valve. ![]() Dr Anja Krieger-Liszkay, CEA Saclay, France
![]() Dr Anja Krieger-Liszkay, CEA Saclay, FranceMy main research interest is acclimation of higher plants to abiotic stress with an emphasis on regulation of photosynthetic electron transport and generation of reactive oxygen species. I characterize physiologically important processes by a combination of biophysical/biochemical/physiological techniques integrating in vivo observations and studies characterizing isolated systems on a molecular level. During my PhD thesis supervised by E. Weis at the University Düsseldorf and Postdoctoral studies (with A. W. Rutherford (CEA Saclay), with U. Heber (Würzburg University) and at Freiburg University) I have characterized a molecular switch which modulates the yield of singlet oxygen formation in photosystem II. After my appointment as researcher at the CNRS (CEA Saclay) I extended this work towards the characterization of signaling pathways triggered by singlet oxygen and hydrogen peroxide. Nowadays, I have started to characterize the plastid terminal oxidase and I got interested in redox regulation at the level of photosystem I. |
Chair
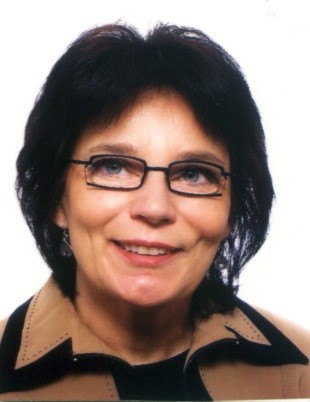
Professor Eva-Mari Aro, University of Turku, Finland
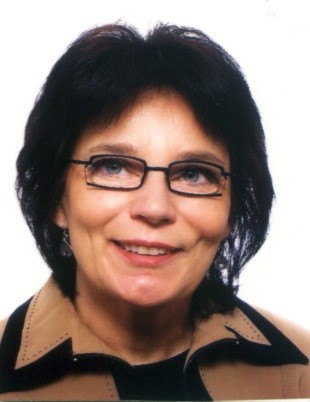
Professor Eva-Mari Aro, University of Turku, Finland
Eva-Mari Aro is a professor in Molecular Plant Biology at Turku University, Finland. Academy professor 1998-2008 and 2014-2018. Research: photosynthesis, plant stress biology, cyanobacteria for sustainable applications. President/past president of the International Society of Photosynthesis Research 2004-2010, board member of European Plant Science Organisation and European Academies of Science Advisory Council (EASAC), President and vice president of the Finnish Academy of Science and Letters (2012-2015). Chair of the Finnish Centers of Excellence: 2008-2014 "Integrative Photosynthesis and Bioactive Compound Research at Systems Biology Level" and 2014-2019 “Molecular Biology of Primary Producers”. Honorary doctor of the Chinese Academy of Sciences, University of Umeå and University of Helsinki.
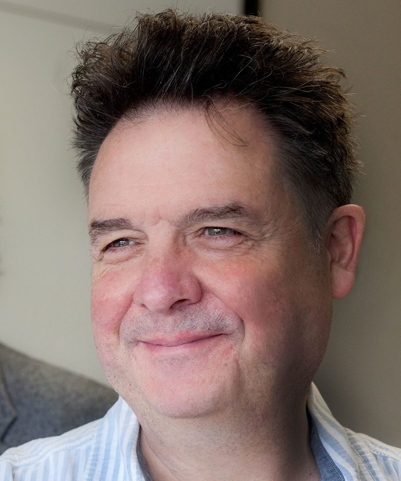
Dr Jeremy Harbinson, Wageningen University, Netherlands
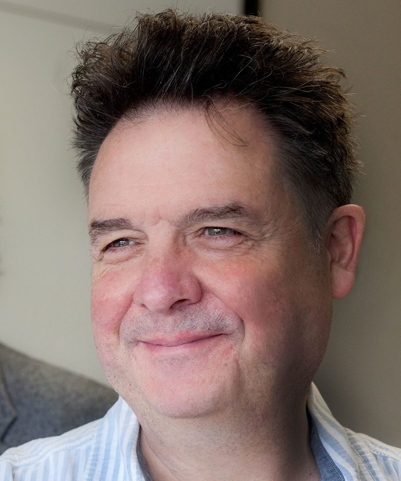
Dr Jeremy Harbinson, Wageningen University, Netherlands
Born in Belfast, Northern Ireland, Dr Harbinson studied plant sciences in Cambridge, where he later did a PhD in plant ecophysiology under the supervision of Ian Woodward. During this PhD he developed an interest in photosynthesis and the use non-destructive, biophysically based methods, such as chlorophyll fluorescence, to monitor the operation and regulation of photosynthesis in vivo. He then did a post-doc at the John Innes Institute from 1984 to 1990, working with Cliff Hedley. In 1990 he moved to Wageningen, the Netherlands, where he still work. Until 2000 he worked in a DLO Institute, after which he moved to Wageningen University where he work in the Horticulture group. Dr Harbinson’s current research interests are in the operation and regulation of photosynthesis, and the high-throughput phenotyping of photosynthesis and it use to understanding better the genetic basis of the natural variation in photosynthetic traits.
09:00 - 09:30 |
Improving plant photosynthesis by its redesign in bacteria
The light reactions of plant photosynthesis are an evolutionary patchwork, providing ample scope for their improvement. This particularly concerns its light-harvesting components and the susceptibility of photosystems to photodamage. Classical approaches involve genetic engineering and, more indirectly, conventional breeding. In the long-term, synthetic biology might allow the redesign or de novo creation of entire photosystems that are more efficient because they are less susceptible to photodamage and produce fewer harmful reactive oxygen species. This redesign of entire photosystems is a formidable challenge, but the gain in photosynthetic efficiency when photosystems require less repair and photoprotection will be significant. It is clear that crop plants are the least suited test systems for such approaches, given their long life cycle and inaccessibility to efficient genetic engineering. Therefore, redesigning photosystems will not only require a very deep understanding of the structure and dynamics of the natural photosynthetic light reactions, but also innovative concepts of what a perfect photosystem should look like, as well as novel model organisms in which such concepts can be realized, tested, and reiteratively improved. Obviously, bacteria are the model system of choice and corresponding concepts will be elaborated in this presentation. ![]() Professor Dr Dario Leister, Ludwig-Maximilians-University Munich (LMU), Germany
![]() Professor Dr Dario Leister, Ludwig-Maximilians-University Munich (LMU), GermanyDario Leister is a professor of plant molecular biology at Ludwig-Maximilians-University in Munich. His lab is engaged in the regulation of photosynthesis and the integration of photosynthetic processes in the eukaryotic cell, focusing on intracellular signal transduction, posttranslational protein modification, thylakoid biogenesis, and synthetic biology. He holds a PhD in genetics from the Max Planck Institute for Plant Breeding Research. |
|
---|---|---|
09:30 - 10:00 |
Dynamic responses of photosynthesis: minding the gap between the lab and the field
Some of the most pressing open questions about photosynthesis concern how it is adapted to function in living organisms to balance the needs for efficient energy capture and the avoidance of toxic side reactions. I will describe a series of enabling technologies designed to bridge the gaps between the lab and the field that can be and how these tools can be used to observe the biophysical machinery of photosynthesis in action under the conditions of the field. I will describe new results using these tools to explore the relationships between photochemistry and photoprotection in a wide range of wild type and mutant plants, both in the lab and the field. These results identify potential biophysical “Achilles Heels” of photosynthesis that involve the interactions of electron and proton transfer reactions. In one case, loss of thylakoid proton motive force leads to buildup of electrons on, and subsequent photodamage of, photosystem I. In another case, excessive proton motive force that drives ATP synthesis and down-regulates light capture also induces singlet oxygen production by stimulating recombination reactions within photosystem II. I will also show data that suggests that this inherent weakness in the photosynthetic process limits photosynthesis in the field, and likely drove the evolution of a large number of “ancillary regulatory components” of photosynthesis to tune its responses to the environment. Finally, I will discuss the prospects of using this basic knowledge and new phenotyping tools to improve the breeding and and management of crops, especially in the developing world. ![]() Professor David Kramer, Michigan State University, USA
![]() Professor David Kramer, Michigan State University, USADavid M. Kramer is the Hannah Distinguished Professor of Bioenergetics and Photosynthesis in the Biochemistry and Molecular Biology Department and the MSU-Department of Energy Plant Research Lab at Michigan State University. In 1990, he received his Ph.D. in Biophysics at University of Illinois, Urbana-Champaign, followed by Post-doc at the Institute de Biologie Physico-Chimique in Paris and a 15-year tenure as a faculty member at Washington State University. He took his current position at MSU in 2010. His research seeks to understand how plants convert light energy into forms usable for life, how these processes function at both molecular and physiological levels, how they are regulated and controlled in response to fluctuating environmental conditions, how they define the energy budget of plants and the ecosystem and how they might be improved to increase the efficiency and sustainability of agriculture. This work has led his research team to develop a series of novel spectroscopic tools for probing photosynthetic reactions both in vitro and in vivo. To disseminate these tools, he co-founded Phenometrics, Inc. PhotosynQ.org and the MSU Center for Advanced Algal and Plant Phenotyping. |
|
11:00 - 11:30 |
Structure and function of ATP synthase and its role as new dug target against tuberculosis
F1Fo-ATP synthases are paradigmatic molecular machines, which use the transmembrane electrochemical ion gradient to power ATP synthesis. The enzymes belong to the class of rotary ATPases, which all share a common architecture principle, consisting of a rotor and stator entity. While ions are shuttled through the Fo complex of the enzyme, torque is generated at the rotor/stator and transferred to the F1-catalytic subunits for ATP synthesis. In the opposite direction, ATP hydrolysis can be used to drive ion pumping. In my talk I will present the structure of complete ATP synthase analysed by X-ray crystallography and cryo-electron microscopy. Professor Meier will also focus on biochemical and structural investigations of the ATP synthase rotor rings with respect to their cell physiological function in bioenergetics and as a novel target to treat pulmonary tuberculosis. ![]() Professor Thomas Meier, Imperial College London
![]() Professor Thomas Meier, Imperial College LondonThomas is currently the Chair in Structural Biology at Imperial College London. Prior to this he was a research group leader at the Institute of Biophysics, Germany, and the Institute of Microbiology, Switzerland. He has a Diploma and a PhD in Natural Sciences (Biology), from the Swiss Federal Institute of Technology (ETH) Zurich. |
|
11:30 - 12:00 |
Alternative carbon fixation pathways for improved crop plant productivity
Plant productivity is limited by the oxygenation reactino of Rubisco, which leads to the energetically costly photorespiratory pathway. Plants and cyanobacteria have, to varying degree, carbon concentrating mechanisms, that concentrate carbon dioxide around Rubisco to reduce the level of the oxygenation reaction. Transferring these mechanisms to crop plants is a major goal. In the longer term, it may be possible to escape using Rubisco and the Calvin cycle completely, and to fix carbon with a new pathway that is not oxygen sensitive. One example is the autotrophic 3-hydroxypropionate (3HP) pathway of the photosynthetic Chloroflexus group of bacteria. There are other synthetic pathways designed from individual enzymes. I will discuss the potential and problems of transferring new autotrophic carbon fixation pathways to plants, algae and cyanobacteria. For example, under conditions of low photorespiration, the Calvin cycle is very efficient. I will describe our flux-balance modelling work on cyanobacterial metabolism under different conditions with different carbon fixation pathways in place. We have made some progress in characterising the enzymes of the 3HP pathway, and in expressing them in the model cyanobacterium Synechocystis 6803. ![]() Dr James W. Murray, Imperial College London, UK
![]() Dr James W. Murray, Imperial College London, UKJames Murray received his BA in biochemistry from Cambridge in 2000, and his DPhil in structural biology from Oxford in 2005. He worked with Jim Barber at Imperial College on the structure of Photosystem II, before receiving a David Phillips Fellowship to work on protein design for biomimetic photosynthesis. He is currently a lecturer in the department of Life Sciences at Imperial College. Dr Murray's research interest is in the structural basis of the light and dark reactions of photosynthesis. He is working on ways to modify the carbon fixation reactions in cyanobacteria to improve on the efficiency while retaining tolerance of oxygen. He has also interested in mechanisms by which nitrogenase may be protected from oxygen. |
Chair
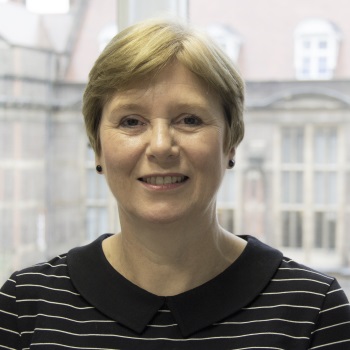
Professor Alison G Smith, University of Cambridge, UK
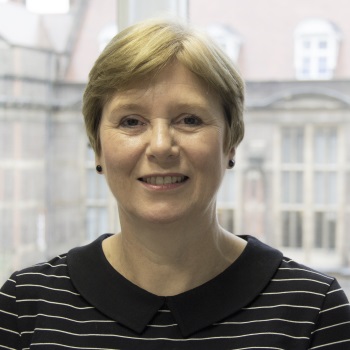
Professor Alison G Smith, University of Cambridge, UK
Alison Smith is Professor of Plant Biochemistry in the Department of Plant Sciences. Her research interests are focussed around the metabolism of plants, algae and bacteria, in particular of vitamins and cofactors, and of biofuel precursors. Her lab employs a wide range of techniques from biochemistry through molecular biology to genomics, and biophysical and analytical methods, as well as mathematical modelling. Current projects include the use of synthetic biology approaches in metabolic engineering of plants and algae to enhance production of vitamins and other chemicals that might reduce the use of fossil fuels. A second area is understanding algal-bacterial interactions, following Smith’s discovery that half of known microalgal species need an external source of vitamin B12, which they get from bacteria - in turn the bacteria get fixed carbon from the algae. This has implications both for the production of dense algal cultures in open ponds and for aquatic ecosystems generally.
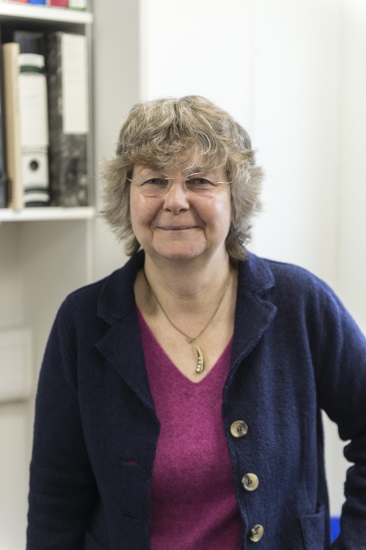
Professor Alison M. Smith, John Innes Centre, UK
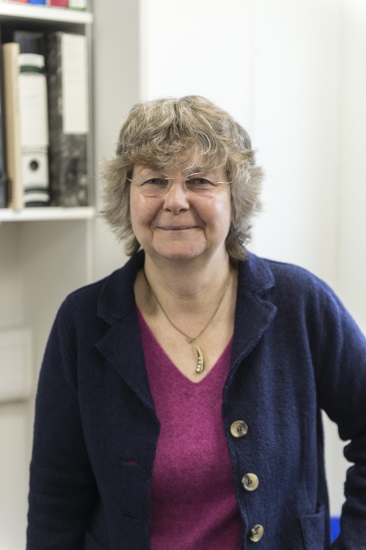
Professor Alison M. Smith, John Innes Centre, UK
Alison Smith is the Programme Leader for plant and microbial metabolism (at the John Innes Centre in Norwich, UK, and an Honorary Professor at the University of East Anglia in Norwich. Her interest is in the synthesis and degradation of starch and sugars in relation to plant growth and development. Her lab studies both “model” plants and crop plants including barley and wheat: research topics include how carbon assimilated in photosynthesis is partitioned between sugars and starch, how sugars arriving in non-photosynthetic organs are partitioned between metabolism and storage, and how the degradation of starch is co-ordinated with the requirement for sugars for metabolism and biosynthesis – in leaves at night and in non-photosynthetic organs. Alison also has a broad interest in how fundamental discoveries about plant biology can be used to promote crop productivity and enhance food security in the face of climate change.
13:30 - 14:00 |
Improving photosynthesis to improve crop yield
Increasing demands of the growing world population for food and fuel are putting ever greater pressure on the need to develop higher yielding crop varieties. It has been estimated that increases of 50% will be required in the yield of grain crops such as wheat and rice if food supply is to meet the demands of the increasing world population. The primary determinant of crop yield is the cumulative rate of photosynthesis over the growing season which is the result of the crop’s ability to capture light, the efficiency by which this light is converted to biomass and how much biomass is converted into the usable product e.g. grain in the case of wheat and rice. There is compelling evidence from transgenic studies that the manipulation of the Calvin-Benson cycle enzyme SBPase could increase yield in a range of species, including wheat, tobacco and Arabidopsis. More recently we have shown that overexpression of SBPase in combination with FBPaldolase, ictB (from cyanobacteria) or a component of the algal electron transport chain can lead to a further increase in photosynthesis and biomass. Our approaches to the manipulation of photosynthesis will be presented together with results from both greenhouse and field studies. ![]() Professor Christine Raines, University of Essex, UK
![]() Professor Christine Raines, University of Essex, UKChristine Raines graduated with a B.Sc. (Hons) Agricultural Botany, First Class from Glasgow University in 1982. Following which she was awarded a Glasgow University Scholarship and studied for a Ph.D. in Photosynthesis graduating in 1986. Christine’s post-doctoral research started in late 1985 at the Institute of Plant Science Research, Cambridge where she initiated a project cloning nuclear genes encoding enzymes of the C3 cycle. In 1988 Christine was appointed to a faculty position at the University of Essex being promoted to Professor in 2004. Christine is currently Head of the School of Biological Sciences at Essex. Christine also holds a number of external roles; Editor in Chief, Journal of Experimental Botany (2011- ), Co-Opted Member of UK Plant Federation Executive (2012- ). Past roles include Associate Editor, Journal of Experimental Botany (2006-2011), Chair of Plant Section, Society of Experimental Biology (2009- ), BBSRC Biofuels Committee (2007/8), EPSRC Bioenergy Committee (2008), Nuffield Bioethics Committee on Biofuels (2009-2011). Christine’s research interests are in the area of plant molecular physiology including the isolation and characterisation of photosynthetic genes, analysis of gene expression and production and analysis of transgenic plants. A major focus of the work in the Raines’ lab currently is on improvement of photosynthetic carbon assimilation in transgenic plants to increase plant yield. Christine has authored or co-authored approx 70 peer-reviewed research papers and 6 reviews or book chapters. |
|
---|---|---|
14:00 - 14:30 |
Overcoming the limitations of Rubisco
The light driven assimilation of CO2 in photosynthesis is the primary determinant of crop biomass and yield. Whilst the current theoretical maximum efficiency of photosynthesis of C3 crops is 4.5%, it averages less than 1% in the field. Improving this conversion efficiency is a key opportunity to both increase yield and also the efficiency with which other resources are used. Overcoming the limitations of Rubisco (ribulose-1,5-bisphosphate (RuBP) carboxylase/oxygenase) is a simple and direct way towards improving this efficiency. This is because some characteristics of Rubisco make it surprisingly inefficient and compromise photosynthetic productivity. For example, Rubisco catalyses a wasteful reaction with oxygen that leads to the release of previously fixed CO2 and NH3 and the consumption of energy during photorespiration. Furthermore, Rubisco is slow and large amounts are needed to support adequate photosynthetic rates. The catalytic properties of Rubiscos isolated from diverse sources vary considerably, suggesting that changes in the speed, affinity, or specificity can be introduced to improve Rubisco performance in specific crops and environments. An analysis of natural catalytic diversity has revealed amino acids under positive selection and allowed prediction of amino acid substitutions that could improve Rubisco in different crops. Tobacco lines expressing ‘faster Rubisco’ - that are capable of higher photosynthetic rates per unit Rubisco at high CO2 concentrations than the native enzyme - have already been generated, with further changes in progress. ![]() Professor Martin Parry, Lancaster University, UK
![]() Professor Martin Parry, Lancaster University, UKMartin Parry is the Professor of Plant Science for Food Security at Lancaster University. His aim is to understand how yield and quality are determined by gene composition and sequences in different environments (e.g. drought and temperature stress). This will enable him to manipulate the appropriate molecular and biochemical controls to increase in crop performance in a predictable way for current and future environments. With his group, he has identified and quantified heritable genetic variation that can be exploited in crop breeding and using disruptive biotechnological approaches, has explored ways to increase photosynthesis and yield. In 2014, in recognition of his contributions to the practice, growth and development of applied biology, Martin was made an Honorary Member of the Association of Applied Biologist. In 2014 he was also awarded the China National Friendship Award by Vice Premier Ma Kai in Beijing. |
|
15:30 - 16:00 |
Understanding the evolution of C4 photosynthesis
Along with mimicry and the camera-like eye, the C4 pathway is a remarkable example of the repeated evolution of a complex trait. In fact, despite its complexity, C4 photosynthesis has evolved in at least 66 independent plant lineages. I will discuss molecular mechanisms that have likely facilitated the repeated evolution of the C4 trait. This will include the repeated use of the same cis-elements and homologous transcription factors, to control C4 gene expression, as well as the use of pre-existing gene regulatory networks. I will also use information from the C3 model A. thaliana to illustrate some of these phenomena. ![]() Professor Julian Hibberd, University of Cambridge, UK
![]() Professor Julian Hibberd, University of Cambridge, UKResearch focuses on the evolution of C4 photosynthesis from the ancestral C3 state. This has included analysis of genes that are recruited into the C4 pathway as well as the proteins they encode. Through analysis of C3 species, work has provided insight into the ancestral role of proteins that are recruited into C4 photosynthesis. After developing the most closely related C4 species to Arabidopsis as a model, the laboratory identified the first regulatory DNA and RNA elements that underpin the compartmentalized expression of multiple genes in the C4 leaf. |
|
16:00 - 16:30 |
Engineering carboxysome cores and bacterial microcompartments for enhancing photosynthesis
Dissociating the complexity of photosynthetic processes into modules is a shift in perspective from the single gene/gene product to discrete functional and evolutionary units. Modules can be defined as semi-autonomous functional units, with relatively strong intramodule functional connectivity among components and weaker, yet important, inter-module connections and interfaces. By virtue of their potential for “plug and play” into new contexts, modules can be viewed as units of both evolution and engineering. By coupling modular thinking with the technical advances that have made large scale DNA fabrication affordable, the prospects of engineering organisms by installing new functional modules becomes attainable. Bacterial microcompartments (BMCs) such as carboxysomes illustrate biological modularity in the form of a multienzyme-containing proteinaceous organelle. The carboxysome is a self-assembling metabolic module for CO2 fixation found in all cyanobacteria. These large (~100-500 nm) polyhedral bodies sequester Carbonic Anhydrase and RuBisCO within a protein shell, thereby concentrating substrates and protecting RuBisCO from oxygen generated by the light reactions. Because carboxysomes and other BMCs function to organize reactions, sequester substrates, cofactors, or toxic intermediates and to protection of oxygen sensitive enzymes, they have received considerable attention for bioengineering of heterologous pathways, in addition to the goal of installing carboxysomes directly into plants. There are two central challenges to these goals: design and assembly of multi-enzyme cores and tailoring of shell proteins to serve as the interface between the cytosol and the encapsulated reactions. These challenges and proof-of-concept solutions will be discussed.
![]() Professor Cheryl Kerfeld, Michigan State University and Berkeley National Laboratory, USA
![]() Professor Cheryl Kerfeld, Michigan State University and Berkeley National Laboratory, USACheryl A. Kerfeld is the Hannah Distinguished Professor of Structural Bioengineering in the DOE Plant Research Laboratory and the Department of Biochemistry and Molecular Biology at Michigan State University. She also holds appointments with the Physical Biosciences Division of Lawrence Berkeley National Laboratory, the Department of Plant and Microbial Biology at UC Berkeley and the Berkeley Synthetic Biology Institute. Her research group focuses on structure-based characterisation and engineering of photoprotection and the carbon concentrating mechanism in cyanobacteria; her group is also developing bacterial microcompartment-based systems for metabolic engineering. The Kerfeld group combines methods in bioinformatics, cellular imaging, synthetic and structural biology for the engineering bacterial metabolism. |
|
16:30 - 17:00 | Future directions |