Links to external sources may no longer work as intended. The content may not represent the latest thinking in this area or the Society’s current position on the topic.
Mechanisms of asymmetric cell division
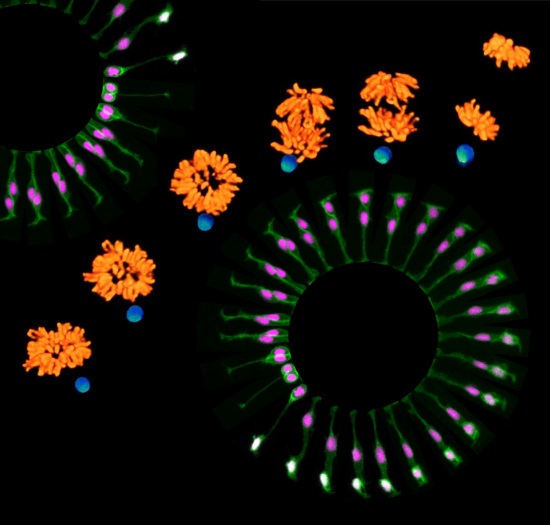
Theo Murphy scientific meeting organised by Dr Shukry Habib and Dr Paula Alexandre
Asymmetric Cell Division (ACD) produces two daughter cells with different fates. This process is implicated in the development of multicellular organisms and tissue maintenance during homeostasis and injury. This meeting focused on the molecular mechanisms that regulate ACD and aimed to create a network amongst early-mid career scientists. The meeting discussed unpublished data, future directions and the implications for human diseases.
Speaker biographies, and the schedule of talks are available below. Speaker abstracts are also available below.
Attending this event
This meeting has taken place.
Recorded audio of the presentations are available below. For more information, please contact the scientific programmes team.
Organisers
Schedule
Chair
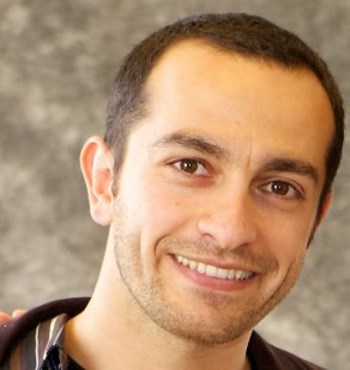
Dr Shukry Habib, King's College London, UK
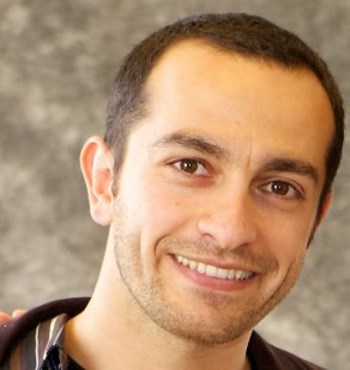
Dr Shukry Habib, King's College London, UK
Shukry Habib pursued his undergraduate studies in biology at the Technion in Israel. He received his Master’s degree in Biochemistry from Tel Aviv University in Israel under the supervision of Professor Abdussalam Azem. Shukry completed his graduate doctorate studies (Dr. rer. nat) in Biochemistry under the supervision of Professor Walter Neupert and Professor Doron Rapaport at the Institute of Physiological Chemistry, LMU in Munich, Germany. His graduate studies focused on the biogenesis of mitochondrial membrane proteins. Shukry became a postdoctoral fellow in the laboratory of Professor Roel Nusse at Stanford University, California. There, incorporating advanced imaging techniques and bioengineering, he established a novel system to study asymmetric cell division of mammalian stem cells. During this project, Shukry joined the Janelia (HHMI) visitor program and worked together with the group of the Nobel Laureate Dr Eric Betzig to visualize cell division at super-resolution under real time. Currently Shukry is a principal investigator at the Centre for Stem Cells and Regenerative Medicine at King's college London.
Shukry has received numerous awards and honors including, Minerva fellowship (Max Planck Society), EMBO fellowship, DFG fellowship and Siebel scholar. He is a member of the UK regenerative medicine platform and was awarded the Sir Henry Dale fellowship (Wellcome Trust and Royal Society, UK), one of the most prestigious awards for young investigators.
09:10 - 09:55 |
Evolutionarily conserved cytokine receptor-Eb1 axis orients asymmetric cell division
Asymmetric cell division (ACD) is a critical mechanism to generate diverse cell types. Drosophila male germline stem cells (GSCs) divide asymmetrically by orienting their spindle toward the niche component hub cells. The hub cells secrete the self-renewal ligand Upd to activate a cytokine receptor-like Dome in GSCs. In the talk, Yamashita shows that Upd-Dome dictates spindle orientation via direct binding to Eb1, a major microtubule regulator. Dome-Eb1 interaction is mediated by SxIP Eb1-binding motif in the cytoplasmic tail of Dome. Strikingly, Yamashita discovers that cytokine-receptor-Eb1 axis is conserved in mammalian cells, where gp130, a mammalian homolog of Dome, regulates centrosome orientation at the immunological synapse in T cells via SxIP motif. Yamashita’s study reveals an evolutionarily conserved mechanism to orient centrosomes and spindles, coupled with engagement of ligand and receptor. ![]() Professor Yukiko Yamashita, University of Michigan Ann Arbor, USA
![]() Professor Yukiko Yamashita, University of Michigan Ann Arbor, USAYukiko Yamashita obtained her PhD from Kyoto University, Japan. She completed the postdoctoral fellowship with Minx Fuller at Stanford University, and then started her own laboratory at the University of Michigan in 2007. She is currently an Associate Professor at the University of Michigan. Yamashita studies how adult stem cells divide asymmetrically, giving rise to one stem cell and one differentiating cell, to maintain tissue homeostasis in the context of the stem cell niche. The particular focus is on how general cell biological processes, such as cell cycle regulation and cytoskeleton organisation, which are shared by many other non-stem cells, are modulated in a stem cell-specific manner to fulfil stem cell function. Yamashita is a recipient of the 2008 Searle Scholar Award, 2009 ASCB WICB junior award, 2011 MacArthur Fellowship, and has been an HHMI investigator since 2014. |
|
---|---|---|
10:00 - 10:25 |
Role of centrosomes in asymmetric cell division: lessons from budding yeast
Along which axis chromosomes segregate is a key determinant of cell fate in asymmetrically dividing cells. In budding yeast, transition from mitosis to G1 is tightly coordinated with the position of the mitotic spindle to assure chromosome segregation along the cell polarity axis prior to mitotic exit and cytokinesis. A centrosome-associated signalling cascade, the mitotic exit network (MEN) triggers exit from mitosis, while the surveillance mechanism of the spindle position checkpoint (SPOC) inhibits MEN if the mitotic spindle fails to align along the cell polarity axis. The mother cell cortex and centrosome-associated kinase Kin4 lies in the heart of SPOC signalling. The Pereira group performed a genetic screen to identify novel regulators of Kin4 and SPOC. In the talk, the analysis of a sub-set of novel regulators will be presented. Furthermore, Pereira discusses how temporal and compartment-specific regulatory networks determine mitotic exit in SPOC and MEN signalling. ![]() Dr Gislene Pereira, University of Heidelberg, Germany
![]() Dr Gislene Pereira, University of Heidelberg, GermanyGislene Pereira is an independent group leader at the Centre for Organismal Studies and Cancer Research Centre at the University of Heidelberg, Germany. She has trained at the University of São Paulo, Brazil (BSc in Pharmacy and MSc in Biochemistry), Ludwig-Maximilliam University, Munich, Germany (PhD in Biochemistry) and at the Beatson and Paterson Institutes for Cancer Research, UK (postdoctoral fellow). Before moving to Heidelberg, she was a Lecturer at the School of Biological Sciences, University of Manchester, UK. Her research interests cover centrosome-driven cell signalling, cilia biogenesis and the control of cell polarity/asymmetric cell division. Her work pioneered the elucidation of a spindle-related mitotic checkpoint and defined the mode of centrosome inheritance in asymmetrically dividing yeast cells, which later proved to be conserved in stem cells of higher eukaryotes. Her laboratory also identified novel regulators of cell polarity establishment and important molecular players that regulate cilia biogenesis at the centrosome. |
|
11:00 - 11:25 |
Chromatin remodelling is essential for Hippo-mediated asymmetric cell division
The polarised partitioning of proteins in cells underlies asymmetric cell division, which is an important driver of development and cellular diversity. Like most cells, the budding yeast Saccharomyces cerevisiae divides asymmetrically to give two distinct daughter cells. This asymmetry mimics that seen in metazoans and the key regulatory proteins are conserved from yeast to human. A well-known example of an asymmetric protein is the transcription factor Ace2, which localises specifically to the daughter nucleus, where it drives a daughter-specific transcriptional network. Thorpe performed a reverse genetic screen to look for regulators of asymmetry based on Ace2 localization phenotype. Thorpe screened a collection of essential genes in order to analyse the effect of core cellular processes in asymmetric cell division. The group identified members of the evolutionary conserved FACT chromatin-remodelling complex and show that these are required for both asymmetric and cell cycle regulated localization of Ace2. ![]() Dr Peter Thorpe, The Francis Crick Institute, UK
![]() Dr Peter Thorpe, The Francis Crick Institute, UKPeter Thorpe completed his PhD at the University of Edinburgh with Noreen Murray studying restriction and modification enzymes. He then moved to work with David Porteous at the MRC Human Genetics Unit to test a new type of therapy to correct mutations that cause cystic fibrosis (CF). This technique relied upon a novel type of gene targeting and he showed that the efficiency of the process was too low for clinical use in CF patients. Consequently, he moved to work with Rodney Rothstein at Columbia University in New York. Rodney developed modern gene targeting techniques in yeast in the 1980s and his laboratory provided an ideal environment to study genetic recombination, in particular by exploiting fluorescent reporters. This led to work exploring how different adult tissues have varying abilities for DNA repair and to the mechanisms underlying cell division asymmetries, which has been his focus since becoming an independent researcher. |
|
11:30 - 11:55 |
Molecular mechanisms of spindle coupling to cortical polarity during asymmetric cell divisions
Asymmetric cell divisions balance stem cell proliferation and differentiation to sustain tissue morphogenesis and homeostasis. During asymmetric divisions, fate determinants and niche contacts segregate unequally between daughters, but little is known on how this is achieved mechanistically. In Drosophila neuroblasts and murine mammary stem cells, the association of the spindle orientation protein LGN with the stem cell adaptor Inscuteable has been connected to asymmetry. Mapelli will present the crystal structure of LGN in complex with the asymmetric domain of Inscuteable, which reveals a stable tetrameric arrangement of intertwined molecules. Biochemical analyses show that Insc:LGN tetramers constitute stable cores for the assembly of conserved Par3-Insc-LGN-G(alpha)iGDP complexes, which cannot be dissociated by NuMA. In mammary stem cells, the asymmetric domain of Insc suffices to drive asymmetric fate, and reverts aberrant symmetric divisions induced by p53 loss. Intriguingly, structural studies also revealed that the C-terminal portion of NuMA assembles in hetero-hexamers with LGN-TPR, which are the functional oligomeric units driving mitotic spindle orientation. Based on these findings, we propose that two independent pools of LGN contribute to asymmetry in different ways: the Insc-bound pool by coordinating the distribution of fate determinants to promote asymmetric fate specification, and the more abundant NuMA-bound pool by providing cortical information to orient the division plane in a Dynein dependent manner. ![]() Dr Marina Mapelli, European Institute of Oncology, Italy
![]() Dr Marina Mapelli, European Institute of Oncology, ItalyMarina Mapelli graduated in Physics at the University of Milan (Italy). She spent her PhD at the EMBL of Heidelberg and Hamburg, and obtained her PhD in Crystallography from the Birkbeck College in London. As a postdoc at the European Institute of Oncology with Professor Andrea Musacchio, she studied Mad2 complexes and the Aurora-B kinase. Upon becoming a group leader at the European Institute of Oncology of Milan in 2009, she became interested in the molecular mechanisms governing asymmetric stem cell divisions, with a focus on how the mitotic spindle is coordinated with cortical polarity and niche contacts. Major contributions from her laboratory to the field include studies of Inscuteable:LGN, Afadin:LGN, and NuMA phosphorylation by Aurora-A. |
|
12:00 - 12:25 |
Subcellular patterning mechanisms in Drosophila neuroblasts
Different cell types are generated by asymmetric segregation of fate determinants during neuroblast divisions in the developing nervous system of Drosophila. The establishment of cortical polarity precedes the localization of determinants such as Miranda, but how this is controlled remains unclear. Using live cell imaging of endogenous reporters for the Par complex and Miranda, Januschke reveals the highly dynamic nature of neuroblast polarization. Januschke shows that Miranda uses two different modes to interact with the NB cortex. In interphase Miranda binds to the plasma membrane via a previously identified aPKC regulated basic and hydrophobic motif. After nuclear envelope breakdown Miranda switches to a binding mode dependent on actin and myosin activity to anchor it to a basal affinity zone. Therefore, different mechanisms control establishment of Miranda asymmetry and maintenance. His findings support a model integrating aPKC-mediated phospho-regulation of determinant localization and differential binding to the actin cortex. ![]() Dr Jens Januschke, University of Dundee, UK
![]() Dr Jens Januschke, University of Dundee, UKDr Jens Januschke started his independent career with a Sir Henry Dale fellowship from the Royal Society and the Wellcome Trust in 2013 in the School of Life Sciences, University of Dundee. Januschke moved to the north after working as a postdoctoral fellow in the Institute for Research in Biomedicine in Barcelona where he studied the role of the microtubule network for spindle orientation and centrosome asymmetry in neural stem cells of the developing fly brain. Before that, Jens studied mRNA localisation and its link to body axis specific during oogenesis in Drosophila in the Institut Jacques Monod in Paris and received basic training in Drosophila genetics and cell biology in the Institute of Developmental Biology in the University of Cologne from where he graduated. Currently, Jens is interested in subcellular patterning mechanisms using Drosophila neuroblasts. The Januschke group aims to understand how asymmetric cell division acts as a tumour suppressive condition by dissecting the dynamic processes leading to neuroblast polarization. |
Chair
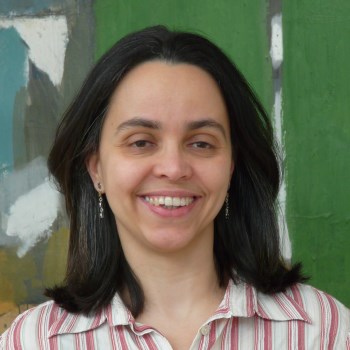
Dr Gislene Pereira, University of Heidelberg, Germany
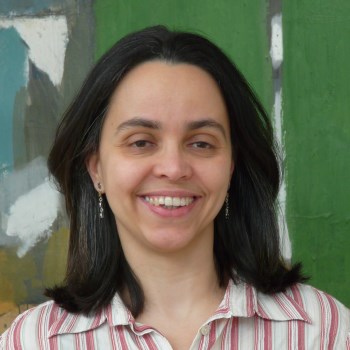
Dr Gislene Pereira, University of Heidelberg, Germany
Gislene Pereira is an independent group leader at the Centre for Organismal Studies and Cancer Research Centre at the University of Heidelberg, Germany. She has trained at the University of São Paulo, Brazil (BSc in Pharmacy and MSc in Biochemistry), Ludwig-Maximilliam University, Munich, Germany (PhD in Biochemistry) and at the Beatson and Paterson Institutes for Cancer Research, UK (postdoctoral fellow). Before moving to Heidelberg, she was a Lecturer at the School of Biological Sciences, University of Manchester, UK. Her research interests cover centrosome-driven cell signalling, cilia biogenesis and the control of cell polarity/asymmetric cell division. Her work pioneered the elucidation of a spindle-related mitotic checkpoint and defined the mode of centrosome inheritance in asymmetrically dividing yeast cells, which later proved to be conserved in stem cells of higher eukaryotes. Her laboratory also identified novel regulators of cell polarity establishment and important molecular players that regulate cilia biogenesis at the centrosome.
13:30 - 13:55 |
Integrating daughter cell self-renewal and fate-choice during retinogenesis
One focus of regenerative medicine is to efficiently and safely replace retinal ganglion cells (RGCs), the output neurons of the retina, which are lost upon glaucoma and optic neuropathies leading to irreversible blindness. To this end, a tightly controlled expansion of the source cell (stem, progenitor or even differentiated neuronal cells) as well as its efficient and safe reprogramming into functional RGCs is needed. Achieving this requires that we understand the complex crosstalk of cell fate determinants, chromatin regulators and self-renewal factors that are at work during the normal genesis of RGCs in vivo. The bHLH transcription factor Ath5 (Atoh7) plays a crucial role in instructing RGC fate acquisition of retinal progenitor cells as well as induced pluripotent stem cells and Müller glia. Expression of ath5, however, is not decisive for RGC commitment and cells escape the RGC fate while progressing through self-renewal states. Poggi is interested in disentangling the integrated molecular interactions, from daughter cell inheritance to transcriptional regulation, which allow ath5-expressing progenitors to elude the RGC differentiation pathway upon asymmetric cell division. To understand this, Poggi began with interrogating gene regulatory networks controlled by Ath5, and to examine them in the physiological cellular context of the three-dimensionally patterned retinal tissue of the developing zebrafish embryo. Here Poggi provides insights suggesting how reciprocal feedbacks between Ath5 and factors influencing multipotency and self-renewal might intersect during asymmetric cell division, to restrict the RGC fate choice of retinal progenitor cells. ![]() Dr Lucia Poggi, University of Heidelberg, Germany
![]() Dr Lucia Poggi, University of Heidelberg, GermanyLucia Poggi received her MSc and PhD in Molecular Cell Biology from the University of Pisa. Following completion of an internship at EMBL Heidelberg, she moved to the University of Cambridge to carry out her postdoctoral work. During this time, Lucia developed time-lapse imaging methods in the retina of the zebrafish embryo to study lineage and modes of division of retinal stem cells in their native environment. After being awarded a DFG Research Grant for Principal Investigator, Lucia was appointed Independent Junior Group Leader in the Centre for Organismal Studies at University of Heidelberg and member of the Interdisciplinary Centre for Neuroscience. She recently joined the Department of Ophthalmology - David J Apple Laboratory at the University of Heidelberg. Her current research interests focus on understanding the mechanisms of asymmetric cell division and daughter cell identity acquisition during eye development and regeneration. |
|
---|---|---|
14:00 - 14:25 |
Asymmetric cell division in Ciona notochord tapering
Tapering body parts are common in nature, but little is known about the developmental mechanisms by which taper arises. The simple 40 cell notochord of the invertebrate chordate Ciona forms a tapered rod, and the Veeman group are using it as a model for dissecting the mechanisms of tapering. Much of the taper reflects the cells at the front and back of the notochord being progressively smaller in volume than cells in the middle. Veeman used a genetic fate mapping strategy based on mosaic expression of an electroporated transgene to show that asymmetric division is the major driver of these anterior to posterior cell volume differences. Cells in the anterior of the notochord primordium divide to give smaller anterior daughters, and cells in the posterior of the primordium divide to give smaller posterior daughters. The volume asymmetries seen are modest compared with many cases of asymmetric division, but two consecutive rounds of division with complex patterns of asymmetry lead to important changes in cell size and ultimately help control the shape of an entire chordate organ. Veeman is currently investigating the cellular and molecular mechanisms driving these novel asymmetric divisions. The mitotic spindle becomes robustly oriented along the AP axis in these cells, but it is not clear if it is being displaced. The group are working to test an alternate hypothesis that some of these asymmetric divisions might involve a centred spindle in the context of an asymmetrically shaped cell. ![]() Dr Michael Veeman, Kansas State University, USA
![]() Dr Michael Veeman, Kansas State University, USAMichael Veeman’s group uses quantitative imaging and functional genomics approaches in the simple chordate Ciona to study how genomes encode spatial information. Ciona has a stereotyped chordate embryonic body plan but in an unusually small, simple embryo well suited to quantitative imaging. One project in the laboratory seeks to understand the functions and mechanisms of asymmetric division in controlling the tapered shape of the larval tail. Another project works towards a systems-level understanding of Ciona notochord morphogenesis. Michael trained at the University of Alberta (BSc), the University of Washington (PhD with Randy Moon) and the University of California Santa Barbara (postdoc with Bill Smith). He has been an Assistant Professor at Kansas State University since 2011. |
|
15:00 - 15:25 |
Live imaging reveals new aspects of zebrafish neurogenesis
During development of central nervous system (CNS) neural progenitors must be able to self-renew while producing neurons by undergoing series of asymmetric divisions. During early stages of zebrafish embryonic development, differentiated neurons appear regularly spaced along the anterior posterior axis of the spinal cord. This suggests that a mechanism that spaces neural progenitors’ asymmetric divisions or differentiating neurons must exist in this region. To determine the cellular and molecular mechanisms that can regulate neuronal patterning in the neural tube, Alexandre used live-imaging in zebrafish embryo to monitor neural progenitors’ divisions and neurons differentiating in vivo. Alexandre discovered that neuronal committed progenitors transiently elongate two long basal processes along the antero-posterior axis of the neural tube. The Alexandre group has evidence that signals delivered by these long processes may prevent neighbours from differentiating. This is the first cellular behaviour found in a vertebrate system that can regulate neuronal patterning in the neural tube. ![]() Dr Paula Alexandre, University College London, UK
![]() Dr Paula Alexandre, University College London, UKPaula Alexandre studied Biochemistry at “Faculdade de Ciencias”, University of Lisbon. Paula obtained her PhD in 2005, working in Dr Marion Wassef’s laboratory at "Ecole Normale Superieure" in Paris and studying the mid hindbrain signals that orchestrate cell movements and refine patterning of the midbrain. During this period she has been awarded several fellowships from "Fundacao para a Ciencia e Tecnologia" (Portugal), "Fondation des Treilles" and "Fondation pour la Recherche Médicale" (France). In 2006, Paula Alexandre joined Professor Jon Clarke’s laboratory at University College London (UCL) as a post doc and later moved with the group to King’s College London (KCL). During this period she developed long-term imaging in a zebrafish embryo to study the mechanisms that regulate progenitors asymmetric divisions during zebrafish neurogenesis and has been awarded an EMBO and IEF Marie Curie’s fellowships. In 2013, funded by the Dorothy Hodgkin Fellowship from the Royal Society, Paula Alexandre joined the UCL Institute of Child Health as a principal investigator where she continues studying the mechanisms that regulate neurogenesis. |
|
15:30 - 15:55 |
Proliferation control in Drosophila neural stem cells
Stem cells are highly abundant during early development but become rare in most adult organs. Stem cell numbers must then be tightly regulated during development, but the molecular mechanisms causing stem cells to exit proliferation at a specific time are unclear. To address the mechanisms triggering stem cell exit during development Homem used Drosophila neural stem cells, the neuroblasts. Neuroblasts undergo size and fate asymmetric divisions to self-renew and generate more differentiated cells. Neuroblasts proliferate during development but all exit cell cycle and disappear before adulthood. Homem’s data show that changes in energy metabolism induced by the steroid hormone Ecdysone together with transcription regulator Mediator initiate an irreversible cascade of events leading to neuroblast differentiation. An increase in the levels of oxidative phosphorylation in neuroblasts leads to uncoupling between cell cycle from cell growth. This results in progressive reduction in neuroblast cell size and ultimately in terminal differentiation. Homem’s findings show that neuroblast size control can be modified by systemic hormonal signalling and reveal a unique connection between metabolism and proliferation in stem cells. ![]() Dr Catarina Homem, CEDOC, Universidade Nova de Lisboa, Portugal
![]() Dr Catarina Homem, CEDOC, Universidade Nova de Lisboa, PortugalCatarina Homem is a Principal Investigator at the Center for Chronic Diseases (CEDOC) at the University Nova of Lisbon, Portugal. Her main research interests are on how stem cell fate and proliferation are regulated during animal development. Her laboratory focuses on the mechanisms of cell fate regulation in neuroblasts, Drosophila's asymmetrically dividing neural stem cells. Catarina Homem did her PhD under the supervision of Dr Mark Peifer at UNC, Chapel Hill, USA where she researched how the actin cytoskeleton dynamically regulates Drosophila morphogenesis. For her postdoctoral work she joined the laboratory of Dr Juergen Knoblich at IMBA in Vienna, Austria where she started exploring the role of metabolism in the regulation of stem cells. |
|
16:00 - 16:25 |
Centrosome asymmetry and Notch signalling in spinal cord neural progenitors
Unequal maturation of centrosomes has been associated with differential fate choices in several models of asymmetric cell division, including vertebrate neural stem cells. Nevertheless, signalling molecules taking advantage of the intrinsic centrosome asymmetry to instruct binary fate choices in sister cells have yet to be identified. Morin’s group found that the mono-ubiquitin ligase Mindbomb1 (Mib1), a regulator of the Notch pathway, localizes to centriolar satellites associated with the daughter centrosome of chick spinal cord progenitors. Using live imaging and fate tracking in the neural tube, we show that during asymmetric divisions, the centrosome carrying this pool of Mib1 is inherited by the prospective neuron. However, in symmetric divisions, a second pool of Mib1 associated with the Golgi apparatus in interphase is released during mitosis, aggregates on the free centrosome, and compensates for the initial Mib1 centrosomal asymmetry. Remarkably, as development of the neural tube proceeds, Mib1 is progressively lost from the Golgi apparatus, correlating with the reduction in symmetric proliferative divisions. Thus, the Golgi apparatus may represent a storage compartment compensating for the centrosome asymmetry of Mib1 in proliferative divisions. As neurogenesis progresses, this compensatory mechanism fades out and neurogenic divisions become predominant. Finally, Morin shows that preventing Mib1 centrosomal association in dividing cells hinders Notch signalling and delays neuronal differentiation in daughter cells. Thus, Morin establishes for the first time a link between centrosome asymmetry and Notch signalling, and propose that changes in the subcellular localization of Mib1 in neural progenitors are instrumental for the progression of neurogenesis. ![]() Dr Xavier Morin, Institut de Biologie de l'Ecole Normale Superieure, France
![]() Dr Xavier Morin, Institut de Biologie de l'Ecole Normale Superieure, FranceAfter graduating from the Ecole Normale Supérieure in Paris in 1995, Xavier Morin obtained a PhD at the Developmental Biology Institute in Marseilles, deciphering the role of transcription factors in mouse autonomous nervous system development. During his postdoc with William Chia at the IMCB Singapore and King’s College London, his contribution to the identification of a key regulator of mitotic spindle orientation and fate decisions in Drosophila sparked his interest in asymmetric division of neural progenitors. Upon returning to France as a junior investigator at the CNRS in 2003, he studied whether concepts discovered in the fly apply to the vertebrate developing nervous system. In 2010, he started the Cell Division and Neurogenesis laboratory at the Biology Institute at the Ecole Normale Supérieure, using the chick embryonic spinal cord to investigate how events occurring during neural progenitors’ division have an impact on the fate of their progeny. |
|
16:30 - 16:55 |
How are mechanical factors controlled during brain formation?
During brain development, neural stem cells define specific niches within different cortical layers to control their cellular environment. Generally, communication to other cells or surrounding matrix by biochemical signalling pathways such as protein-protein interactions or soluble factors have been well studied. Much less studied, however, is how physical properties of the niche can influence behaviour, growth, and differentiation of cells. Among physical properties, stiffness of the matrix has been shown to have direct effects on fate determination in certain stem cell types in vitro. While some studies indicate that stiffness may influence the fate of cultured neural stem cells by unknown mechanisms, little evidence exists on whether this principle holds true during the physiological development of the mammalian brain in vivo. Here, Kosodo has characterized tissue stiffness of the developing mouse brain cortex by using atomic force microscopy to seek a link to the cell fate determination of neural cells via mechanosignalling pathways, and found specific spatiotemporal shifts in stiffness during brain formation. The research aims to establish a novel concept for mammalian neurogenesis and brain development, and the outcomes will likely influence the fields of stem cell and developmental biology by clarifying unknown mechanosignalling mechanisms of somatic stem cells. ![]() Dr Yoichi Kosodo, Korea Brain Research Institute, South Korea
![]() Dr Yoichi Kosodo, Korea Brain Research Institute, South KoreaYoichi Kosodo is a Principal Investigator at the Korea Brain Research Institute (KBRI), Daegu, South Korea. His laboratory investigates mammalian neurogenesis by pursuing the concept of how tissue and cells reciprocally influence and regulate each other to form the robust architecture of our brain. To illustrate such regulatory systems of neurogenesis, the group has been constructing novel models, and examining their consistency by applying interdependent experimental approaches, such as methods of molecular cell biology, live-imaging, quantitative biology and biomaterials. The research group currently focuses on how physical factors in tissue regulates neural differentiation during brain development. |
Chair
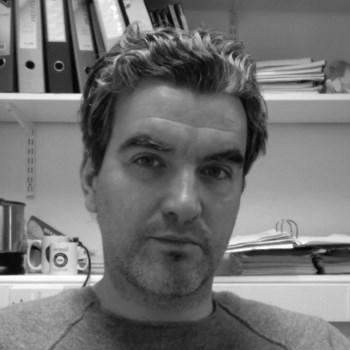
Dr Jens Januschke, University of Dundee, UK
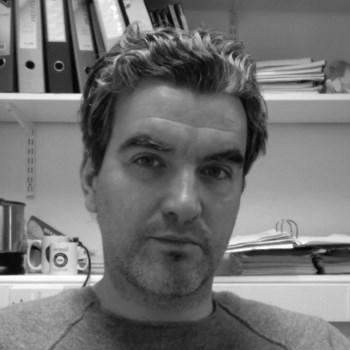
Dr Jens Januschke, University of Dundee, UK
Dr Jens Januschke started his independent career with a Sir Henry Dale fellowship from the Royal Society and the Wellcome Trust in 2013 in the School of Life Sciences, University of Dundee. Januschke moved to the north after working as a postdoctoral fellow in the Institute for Research in Biomedicine in Barcelona where he studied the role of the microtubule network for spindle orientation and centrosome asymmetry in neural stem cells of the developing fly brain. Before that, Jens studied mRNA localisation and its link to body axis specific during oogenesis in Drosophila in the Institut Jacques Monod in Paris and received basic training in Drosophila genetics and cell biology in the Institute of Developmental Biology in the University of Cologne from where he graduated. Currently, Jens is interested in subcellular patterning mechanisms using Drosophila neuroblasts. The Januschke group aims to understand how asymmetric cell division acts as a tumour suppressive condition by dissecting the dynamic processes leading to neuroblast polarization.
09:00 - 09:45 |
Breaking the symmetry – asymmetric histone inheritance in Drosophila male germline stem cell
Many types of stem cells undergo asymmetric cell divisions to give rise to daughter cells with distinct cell fates: one that retains stem cell identity and another that differentiates. During asymmetric cell division, the genomic information is preserved through DNA replication followed by equal partition to the two daughter cells. A long-standing question has been how the epigenetic information of a stem cell is transferred to the daughter cells. Using the Drosophila male germline stem cell lineage, our recent studies showed that epigenetic information is inherited asymmetrically during asymmetric stem cell divisions. Chen's ongoing research is to elucidate how stem cells maintain their epigenetic memory through many divisions and whether the loss of stem cell epigenetic memory leads to diseases. ![]() Dr Xin Chen, Johns Hopkins University, USA
![]() Dr Xin Chen, Johns Hopkins University, USAChen obtained her BSc degree from University of Science and Technology in China in 1996. She did her PhD thesis in Dr Janice A. Fischer’s laboratory at University of Texas at Austin from 1996 to 2001. Chen's PhD thesis was awarded ‘Outstanding Dissertation’, one of the four awardees for the entire 2001–2002 academic year at the University. She proceeded with her postdoc training with Dr Margaret T. Fuller at Stanford University from 2002 to 2007. During her postdoctoral stage, she was among the first group of postdocs who received the NIH Pathway to Independence K99/R00 Award. Chen started her independent position at the Biology Department of Johns Hopkins University in January 2008. The ongoing studies in Chen's laboratory use the Drosophila male germline as a model system to study epigenetic regulation in an adult stem cell lineage. |
|
---|---|---|
09:50 - 10:15 |
Cellular and molecular mechanisms of left-right asymmetric neurogenesis
The Poole group uses C. elegans to reveal fundamental principles of embryonic neuronal cell fate specification. The fully-described lineage provides them with a genetically tractable system to study the early embryonic segregation of neuronal potential with single-cell resolution. One of the embryonic founder blastomeres, known as C, undergoes six successive rounds of cell division to produce left-right (L/R) symmetric pairs of body wall muscles and hypodermal (skin) cells from both sides of the lineage, and a pair of glutamatergic neurons, that arise asymmetrically from the left side of the lineage only. How neuronal potential is segregated in this lineage is not understood. Through classic embryological approaches, 4D-lineage analysis, forward and reverse genetic screening and ‘mapping-by-sequencing’ Poole has uncovered a number of key cellular events and molecular players that regulate L/R asymmetric neurogenesis. Poole has discovered that three successive unequal cell divisions within the lineage result in the asymmetric expression of the key proneural gene hlh-14/ac-sc, which together with its binding partner hlh-2/daughterless are crucially required for L/R asymmetric neurogenesis. The first two of these unequal divisions are regulated by the Mediator complex and the last of these unequal divisions requires hlh-14 itself, in both cases through influencing asymmetric spindle positioning. Together these results suggest that asymmetric segregation of as yet unidentified neuronal determinants has a crucial role in L/R asymmetric neurogenesis and that proneural genes can regulate cell fate and cell size concomitantly. ![]() Dr Richard J. Poole, University College London, UK
![]() Dr Richard J. Poole, University College London, UKRichard is interested in the mechanisms by which diverse types of neurons develop from a fertilized egg. He studied biology at the University of Sussex and his PhD was in Developmental Biology at University College London. Richard then moved to Columbia University to study the mechanisms of left-right asymmetric neuron specification with Professor Oliver Hobert. He established his own research group at UCL in 2012 as a Wellcome Trust Research Career Development Fellow. His team uses the power of C. elegans as an in vivo genetic model system with single-cell resolution to reveal fundamental principles of neural development, which should subsequently provide the basis for novel therapies and brain repair strategies. Richard is focused on uncovering the cellular and molecular mechanisms that regulate left-right asymmetric neurogenesis and more recently is expanding his research in a new direction, to address the mechanisms of plasticity and transdifferentiation during glial-derived neurogenesis. |
|
10:50 - 11:15 |
How stem cells generate diversity among species: a case for root anatomy variability in plants
A key question in evolutionary genetics is how interspecies morphological diversities originate. Plant roots present a large gamma of interspecific phenotypic variation, mostly because roots largely contribute to adaptation to different kind of soils. One example is cortex layer number variability, spanning from none to several. Most of the genetic mechanisms that lead to cortex variability in species with multi-layered cortex are still unknown. Dello Ioio proposes Cardamine hirsuta, a double cortex layered close relative of Arabidopsis thaliana, as a suitable model for comparative studies of cortex proliferation. Utilizing a comparative development approach Dello Ioio found out a novel molecular mechanism controlling ground tissue stem cells activity at the basis of root cortex anatomical variability. ![]() Dr Raffaele Dello Ioio, University of Rome "Sapienza", Italy
![]() Dr Raffaele Dello Ioio, University of Rome "Sapienza", ItalyDello Ioio was born and raised in Rome, Italy. He obtained his MSc in Biology at “Sapienza” University of Rome in 2004. Dello Ioio did his PhD under the supervision of the Professor Sabrina Sabatini (“Sapienza” University of Rome) performing a research about the molecular basis leading to boundaries positioning in roots. Subsequently, he started his post-doctorate in Oxford University and in Max Planck Institute under the supervision of Professor Tsiantis. During the tenure of his postdoc Dello Ioio focused his research on the incoherent genetics underlying boundary formation in roots and on the genetic basis leading to different leaf shapes in nature. In 2014 he was awarded of an independent research grant for young researcher in Italy and he started his group at “Sapienza” University of Rome. Nowadays Dello Ioio's research is focused on the genetic mechanisms leading to different root anatomies in nature. |
|
11:20 - 11:45 |
Subcellular protein localization during asymmetric cell division
Bacterial endospores, amongst the hardiest organisms on earth, are dormant cell types produced by some species to protect the cell’s genetic material during harsh environmental conditions. In the model organism Bacillus subtilis, spores arise from an asymmetric division event, in response to nutrient depletion, that results in the formation of two genetically identical, but morphologically dissimilar, cells that undergo different cell fates. The larger cell then engulfs the smaller cell so that the smaller cell can eventually mature into the spore. During spore formation, proteins must localize to specific subcellular locations to exert their activity at the correct place and time, but the mechanisms underlying these localization events are frequently mysterious. Ramamurthi has discovered that certain shape-sensing proteins localize to specific locations driven by the local shape of the membrane at that location. For example, Ramamurthi found that a cell division protein preferentially embeds in negatively curved (concave) membranes at the onset of asymmetric division to recruit a phosphatase that triggers the compartment-specific activation of a transcription factor in the smaller daughter cell. Similarly, a structural protein that recognizes positively curved (convex) membranes localizes to the surface of the smaller cell after it has been engulfed to recruit other structural proteins that form a static shell that ultimately protects the spore. Ramamurthi proposes that recognition of membrane curvature may be a widely conserved mechanism exploited by proteins that localize specifically during developmental programs. ![]() Dr Kumaran S. Ramamurthi, National Institutes of Health, USA
![]() Dr Kumaran S. Ramamurthi, National Institutes of Health, USADr Ramamurthi received his PhD in Molecular Biology from the University of California, Los Angeles (UCLA), where he studied bacterial toxin secretion. As a postdoctoral fellow at Harvard University, he studied how proteins achieve their proper localization during bacterial cell division and spore formation. A principal finding of these studies was that membrane curvature, defined by the cell’s architecture, can recruit certain shape-sensing proteins to particular subcellular locations. In 2009, he became an Investigator in the National Cancer Institute at the National Institutes of Health (NIH), where his lab employs a combination of genetic, biochemical, cytological, and biophysical approaches to study bacterial cell division and morphogenesis. He currently serves on the General Meeting Planning Committee of the American Society for Microbiology and the Editorial Board of The Journal of Biological Chemistry, and is Co-Director of the NIH-Johns Hopkins University Graduate Partnership Program. |
|
11:50 - 12:15 |
Cell division and the mechanical tissue environment
Cell division timing and orientation must be carefully regulated in order to shape tissues and determine cell fate, preventing defective embryonic development and diseases such as cancer. In single cells, mechanical cues (e.g. tensile forces) are known to influence cell division rate and orientation, but we know very little about how this applies to cells in tissues. Using Xenopus laevis as her experimental model, Woolner aims to reveal how mechanical cues influence cell division in a multi-layered, developing tissue. Subjecting animal cap tissue explants to reproducible tensile (stretching) forces, Woolner observe that division rate increases upon stretch and cells divide oriented along the axis of stretch. The Woolner group are now using this system to investigate potential regulators of division response to tensile force, including non-muscle myosin-2, vinculin and FAK. Furthermore, they are utilising these data to build mathematical models, exploring how cells respond to mechanical forces transmitted across complex tissue environments. ![]() Dr Sarah Woolner, University of Manchester, UK
![]() Dr Sarah Woolner, University of Manchester, UKSarah Woolner was awarded her PhD from UCL in 2005, where she worked with Paul Martin, exploring the cytoskeletal mechanics underlying epithelial fusion in the Drosophila embryo. For her postdoctoral work, Sarah moved to Bill Bement’s laboratory at the University of Wisconsin-Madison. Here she developed techniques to follow mitotic spindles in live Xenopus embryos and used these to uncover novel roles for myosin-10 and actin filaments during mitosis. In 2008, Sarah was awarded a Beit Memorial Fellowship, allowing her to return to the UK to the laboratory of Nancy Papalopulu. Here, she investigated the mechanisms that control mitotic spindle positioning in the developing embryo. In 2012, Sarah was awarded a Wellcome Trust/Royal Society Sir Henry Dale Fellowship to start her own lab in the Wellcome Trust Centre for Cell-Matrix Research at the University of Manchester. Her laboratory is currently exploring how mechanical forces influence cell division in the developing embryo. |
Chair
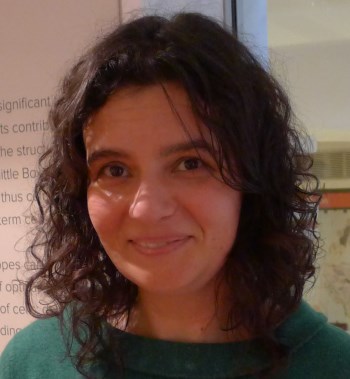
Dr Paula Alexandre, University College London, UK
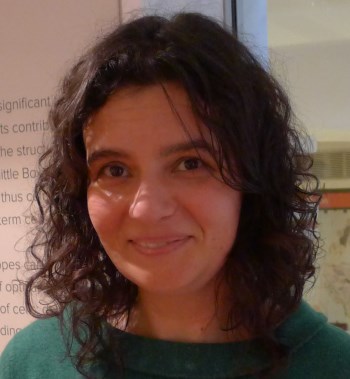
Dr Paula Alexandre, University College London, UK
Paula Alexandre studied Biochemistry at “Faculdade de Ciencias”, University of Lisbon. Paula obtained her PhD in 2005, working in Dr Marion Wassef’s laboratory at "Ecole Normale Superieure" in Paris and studying the mid hindbrain signals that orchestrate cell movements and refine patterning of the midbrain. During this period she has been awarded several fellowships from "Fundacao para a Ciencia e Tecnologia" (Portugal), "Fondation des Treilles" and "Fondation pour la Recherche Médicale" (France). In 2006, Paula Alexandre joined Professor Jon Clarke’s laboratory at University College London (UCL) as a post doc and later moved with the group to King’s College London (KCL). During this period she developed long-term imaging in a zebrafish embryo to study the mechanisms that regulate progenitors asymmetric divisions during zebrafish neurogenesis and has been awarded an EMBO and IEF Marie Curie’s fellowships. In 2013, funded by the Dorothy Hodgkin Fellowship from the Royal Society, Paula Alexandre joined the UCL Institute of Child Health as a principal investigator where she continues studying the mechanisms that regulate neurogenesis.
13:30 - 13:55 |
Membrane remodelling by the ESCRT machinery
In addition to its role in membrane abscission during cytokinesis, viral budding, endosomal sorting and plasma membrane repair, the Endosomal Sorting Complex Required for Transport-III (ESCRT-III) machinery has recently been shown to seal holes in the reforming nuclear envelope (NE) during mitotic exit. ESCRT-III also acts during interphase to repair the NE upon migration-induced rupture, highlighting its key role as an orchestrator of membrane integrity at this organelle. Whilst NE localisation of ESCRT-III is dependent upon the ESCRT-III component CHMP7, it is unclear how this complex is able to engage nuclear membranes. Here, Carlton shows that the N-terminus of CHMP7 acts as a novel membrane-binding module. This membrane-binding ability allows CHMP7 to bind to the Endoplasmic Reticulum (ER), an organelle continuous with the NE, and provides a platform to direct NE-recruitment of ESCRT-III during mitotic exit. CHMP7’s N-terminus comprises tandem Winged-Helix domains and by using homology modelling and structure-function analysis, Carlton identifies point mutations that disrupt membrane-binding and prevent both ER-localisation of CHMP7 and its subsequent enrichment at the reforming NE. These mutations also prevent assembly of downstream ESCRT-III components at the reforming NE and proper establishment of post-mitotic nucleo-cytoplasmic compartmentalisation. These data identify a novel membrane-binding activity within an ESCRT-III subunit that is essential for post-mitotic nuclear regeneration. ![]() Dr Jeremy Carlton, KCL and Francis Crick Institute, UK
![]() Dr Jeremy Carlton, KCL and Francis Crick Institute, UKJeremy obtained a BA in Natural Sciences from Cambridge University and then a PhD from the University of Bristol, where he examined membrane trafficking pathways regulated by the phosphoinositide-binding family of Sorting Nexins in Pete Cullen’s lab. After his PhD, he moved to the laboratory of Professor Juan Martin-Serrano in the Infectious Diseases department of King’s College London to examine how HIV-1 hijacks the ESCRT-machinery to allow its release from cells. Here, Jeremy described a novel and unexpected role for the ESCRT-machinery in cytokinesis and characterised the involvement of ESCRT-III proteins in an Aurora-B regulated abscission checkpoint. After his postdoc, Jeremy moved to the Division of Cancer Studies at King’s College London as a Wellcome Trust Research Career Development Fellow and then a Wellcome Senior Fellow. In Jeremy’s lab, the group continue their focus on membrane trafficking machineries and have described a novel role for the ESCRT-machinery in rebuilding the nuclear envelope during cell division. |
|
---|---|---|
14:00 - 14:25 |
Molecular controls of expansion and neurogenic progenitor differentiation in the mouse cortex
Cortical development starts with the self-renewing expansion of cortical progenitors called neuroepithelium cells (NE). Subsequently, they differentiate into neural progenitors called radial glia (RG), which undergo a limited number of cell divisions generating neurons until they transform into glial progenitors or differentiated ependymal cells. In other words, cortical progenitors undergo fundamental changes in their characteristics during early corticogenesis: from self-renewing progenitors that expand by S-divisions to neurogenic progenitors with limited self-renewing capacity that competently generate neurons by AS-divisions. As most neurons are generated by AS-divisions of RG either directly or indirectly via intermediate progenitors, the machinery that initiates AS divisions must be in during the differentiation from NE to RG to allow them to start neurogenesis. Previously, Sahara identified Fgf10 as a positive regulator of NE-RG transition. With a rationale that upstream and/or downstream factors of the signalling act as regulators of the process that confers neurogenic competence to these progenitors, Sahara performed an intersectional analysis of gain-of- and loss-of-function mutants of Fgf10. Sahara will be discussing the roles of downstream genes on cortical progenitor differentiation. ![]() Dr Setsuko Sahara, King's College London, UK
![]() Dr Setsuko Sahara, King's College London, UKNeural progenitors are the source of all types of neurons and glia. As development proceeds, the self-renewing capacity and multipotency of progenitors to generate various neuronal types becomes restricted. Thus except a few regions such as hippocampus, neural progenitors no longer exist in the adult brains. This explains the poor regenerative capacity as well as the vulnerability of our brains against injuries and degenerative diseases. My laboratory’s research focus on decoding the molecular mechanisms that control multipotency and the self-renewing capacity of neural progenitors in order to develop strategies that encourage regeneration of damaged brains. Specifically we are investigating fate-switching mechanisms that change self-renewing progenitors into neurogenic progenitors in the mouse cortex. |
|
15:00 - 15:25 |
From centrosome dysfunctions to microcephaly: mechanistic insights into neural stem cell loss
The brain is the most vulnerable tissue of our body to chromosome number deviations, also called aneuploidy. Marthiens has recently identified that aneuploidy generated by centrosome amplification impairs embryonic neural stem cell survival. This depletion of the neural stem cell pool culminates in severe brain size reduction at birth, a pathological condition known as microcephaly. Importantly, body size remains unaltered. Marthiens found that the mammalian brain is particularly prone to mitotic errors at early stages of development. The origin of this early susceptibility is not known. In order to characterize the origin of this susceptibility in the mammalian brain, Marthiens compared mitotic spindle assembly pathways at early versus late stages of neurogenesis in WT brains. She observed that the mitotic spindle gains robustness during the course of neurogenesis. This change in spindle morphology come with variations in the levels and/or distribution of spindle assembly factors along the spindle apparatus. For example, Marthiens identified Tpx2, a microtubule nucleating and organizing factor, as one of the key proteins involved in the switch from early to late spindle morphology. Her results provide the first evidence that not all mitotic spindles within the same organ are equally competent to segregate chromosomes correctly. Further, she proposes that the switch in spindle assembly modes observed during neuro-development may favour mitotic accuracy as tissue morphogenesis constraints are decreased. Marthiens’ results may explain the preponderance of defective mitosis observed in adverse situations. ![]() Dr Véronique Marthiens, Institut Curie, Paris, France
![]() Dr Véronique Marthiens, Institut Curie, Paris, FranceMarthiens became interested in the mechanisms driving asymmetric division of mouse embryonic neural stem cells during her first post-doctoral training in the group of Professor Charles ffrench-Constant in Cambridge (UK). At this time, she decided to focus her research on the roles of adhesion molecules in neural stem cell fate decision at the time of division. Marthiens then chose to move her interest to the pathological conditions that affect the development of the mammalian cerebral cortex. She joined the group of Dr Renata Basto at the Institut Curie in Paris in 2009 to investigate the consequences of centrosome dysfunctions on brain development and adult homeostasis. Her work unravelled the deleterious impact of a deregulation of centrosome number for neural stem cell division and survival. Marthiens proposed that this condition is reminiscent of some forms of a human pathology, autosomal recessive primary microcephaly (MCPH), where patients are affected by small brain size at birth and mental retardation. |
|
15:30 - 15:55 |
From Asymmetric stem cell division to tissue engineering
In many tissues stem cells undergo asymmetric cell division (ACD) to produce one daughter stem cell and one differentiated cell. ACD is vital for tissue homeostasis and repair. Wnt signals are key proteins that are involved in stem cell maintenance. In vivo, Wnt proteins are often secreted locally and presented to responsive cells in an oriented manner. To examine the consequences of localised Wnt signaling, we immobilized Wnt proteins onto beads and introduced them to embryonic stem cells in culture. At the single-cell level, the Wnt-bead induced oriented asymmetric distribution of Wnt–β-catenin signaling components, oriented the plane of mitotic division, and directed asymmetric inheritance of centrosomes. Importantly, the Wnt-proximal daughter cell expressed high levels of nuclear β-catenin and pluripotency genes, whereas the distal daughter cell acquired hallmarks of differentiation. We have thus demonstrated, for the first time, that localised Wnts can affect cell fate decisions in single, mammalian stem cells by controlling asymmetric cell division. We are now extending our research to include ACD in adult stem cells and using the principles of Wnt-oriented ACD to engineer human tissues. These studies revealed that localised basal Wnt signals can enrich and maintain stem cells in 2D cultures and recapitulate the stem cell niche as a 3D platform. Specifically, the Wnt-platform maintained the stem population of human mesenchymal stem cells (hMSCs) while directing the generation of organised multilayers of increasingly differentiating cells. ![]() Dr Shukry Habib, King's College London, UK
![]() Dr Shukry Habib, King's College London, UKShukry Habib pursued his undergraduate studies in biology at the Technion in Israel. He received his Master’s degree in Biochemistry from Tel Aviv University in Israel under the supervision of Professor Abdussalam Azem. Shukry completed his graduate doctorate studies (Dr. rer. nat) in Biochemistry under the supervision of Professor Walter Neupert and Professor Doron Rapaport at the Institute of Physiological Chemistry, LMU in Munich, Germany. His graduate studies focused on the biogenesis of mitochondrial membrane proteins. Shukry became a postdoctoral fellow in the laboratory of Professor Roel Nusse at Stanford University, California. There, incorporating advanced imaging techniques and bioengineering, he established a novel system to study asymmetric cell division of mammalian stem cells. During this project, Shukry joined the Janelia (HHMI) visitor program and worked together with the group of the Nobel Laureate Dr Eric Betzig to visualize cell division at super-resolution under real time. Currently Shukry is a principal investigator at the Centre for Stem Cells and Regenerative Medicine at King's college London. Shukry has received numerous awards and honors including, Minerva fellowship (Max Planck Society), EMBO fellowship, DFG fellowship and Siebel scholar. He is a member of the UK regenerative medicine platform and was awarded the Sir Henry Dale fellowship (Wellcome Trust and Royal Society, UK), one of the most prestigious awards for young investigators. |