Links to external sources may no longer work as intended. The content may not represent the latest thinking in this area or the Society’s current position on the topic.
The artificial cell: biology-inspired compartmentalisation of chemical function
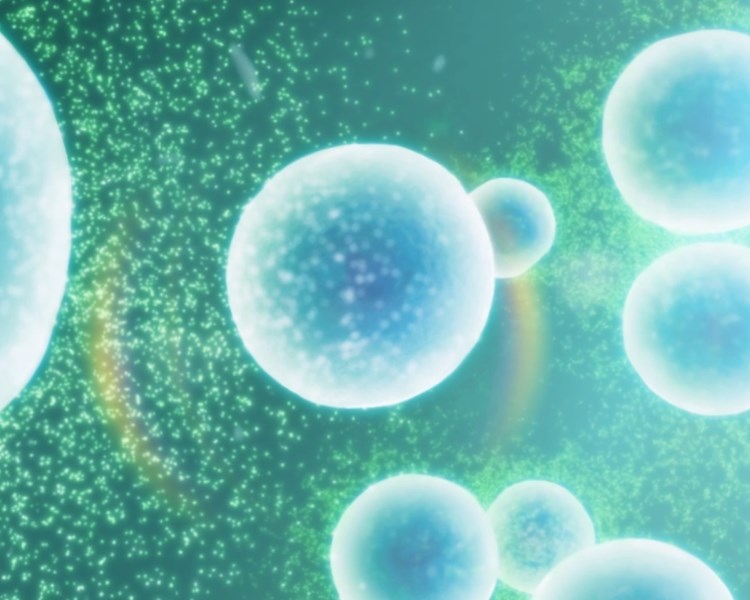
Theo Murphy meeting organised by Dr Barbara Ciani, Dr Paul Beales and Professor Stephen Mann FRS
This meeting brings together life and physical scientists to explore mechanisms of biological compartmentalisation and how these principles can be harnessed to develop smart technologies. We will address how repurposing of biological and synthetic components can be used to create artificial cells for development in areas such as fuel production, drug delivery and environmental remediation.
Speaker biographies and talk abstracts are available below. Recorded audio of the presentations will be available on this page after the meeting has taken place. Meeting papers will be published in a future issue of Interface Focus.
Enquiries: contact the Scientific Programmes team.
Organisers
Schedule
Chair
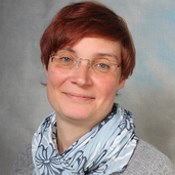
Dr Barbara Ciani, University of Sheffield, UK
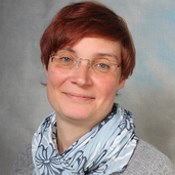
Dr Barbara Ciani, University of Sheffield, UK
Dr Ciani obtained a Laurea in Chemistry at University "La Sapienza" in Rome (1997). This was followed by a DPhil in Biochemistry at the School of Biological Sciences of the University of Sussex (2002). She was a postdoctoral research associate at the Department of Chemistry of the University of Nottingham (2001-2003), and at the Wellcome Trust centre for Cell Matrix research of the University of Manchester (2004-2008). In 2008, she was appointed as a Lecturer in Biophysical Chemistry at the Department of Chemistry of the University of Sheffield.
09:10 - 09:35 |
Navigating the endosome: the role of receptor ubiquitination and ubiquitin-binding adaptors
The endocytic pathway is the gateway between cells and their environment, and promotes diverse events such as nutrient uptake and cell migration. Membrane proteins (“cargoes”) enter endocytic vesicles and reach the endosome. From here they are recycled to the surface, or sorted to intralumenal vesicles (ILVs) to generate a multivesicular body (MVB) en route to the lysosome. The generation of transport intermediates at all points of the endocytic pathway involves the ordered recruitment of an array of membrane-sculpting adaptor proteins, which recognise cargo and coordinate the local induction of membrane curvature. The impairment of these adaptor proteins, or their defective regulation, gives rise to errors in compartmentalisation. One class of endocytic cargo that is transported to the MVB is exemplified by the epidermal growth factor receptor (EGFR). For these cargoes, the sorting signal is generated by receptor ubiquitination, a reversible post-translational modification. A broad range of ubiquitin-binding adaptor proteins recognise ubiquitinated cargoes and promote their endocytic sorting. These adaptors themselves are regulated by reversible ubiquitination, generating a network of ubiquitin homeostasis that governs endocytic trafficking. The key regulators of this network, ubiquitin ligases and deubiquitinating enzymes, must also coordinate their functions with membrane curvature. ![]() Professor Philip Woodman, University of Manchester, UK
![]() Professor Philip Woodman, University of Manchester, UKPhilip Woodman is Professor of Cell Biology at the University of Manchester UK. He obtained a PhD from Cambridge University in 1986, and after a post-doc with Graham Warren in Dundee and at the CRUK London research institute moved to Manchester in 1991 as an MRC Senior Research Fellow. He works on the sorting of signalling receptors within the endosome, with a particular interest in the mechanisms of epidermal growth factor receptor down-regulation. |
---|---|
09:45 - 10:10 |
Decision making in autophagy – How to loose specificity
Autophagy is a pivotal recycling pathway that operates in all eukaryotic cells. More than 40 AuTophaGy related (Atg) proteins are known in yeast and many of them are conserved in humans. In non-stressed, vegetative cells, autophagy mainly recycles unwanted or damaged cytoplasmic components by exclusively engulfing them into autophagic membrane sacks, termed phagophores. In stressed cells, however, autophagy loses its selectivity and degrades bulk cytoplasm. The regulation of this dramatic loss in selectivity is intimately intertwined with the onset of human diseases such as neurodegeneration or cancer. Its molecular bases remained, however, not well understood. Through a combination of classical biochemistry and cell biology with cutting edge in vitro reconstitutions, Dr Wollert and colleagues have been able to solve the long standing question how selectivity is regulated at a molecular level in yeast. They found that the decision is made at the earliest step in autophagy, i.e. nucleation of the phagophore. ![]() Dr Thomas Wollert, Institut Pasteur, France
![]() Dr Thomas Wollert, Institut Pasteur, FranceThomas Wollert studied Biochemistry and Biophysical Chemistry in Potsdam and Hannover (Germany) and obtained his Masters degree in 2003. He carried out his PhD studies at the Helmholtz Centre for Infection Biology, Braunschweig and obtained a PhD in structural biology from the University of Braunschweig (Germany). Thomas did his Postdoc studies in James Hurley’s Laboratory at the National Institut of Health, USA were he investigated the molecular mechanisms of membrane trafficking complexes. He became independent Max-Planck Research Group leader at the MPI of Biochemistry in Martinsried (Germany) in 2010 where he started his research on autophagy using a combination of biophysical, biochemical and cell biology approaches. In 2017, Thomas joined the Institut Pasteur Paris (France) as Directeur de Recherche continuing his research on autophagy. |
10:20 - 10:50 | Coffee |
10:50 - 11:15 |
Compartmentalisation and crowding in artificial membranes
Diffusion of biomolecules in both the cytosol and in the plasma membrane are not simple random walks, instead the measured diffusivity depends on the timescale of observation. Our understanding of why and how this anomalous diffusion exists remains incomplete, and the presence of slower-moving obstacles, pinning sites, and compartmentalisation have all been suggested as potential contributors to anomalous diffusion. Ultimately, this confinement and compartmentalisation must affect the reaction rates of biomolecules, and understanding and exploiting these effects would be a useful tool in designing artificial cellular systems. Here Mark Wallace will present his group’s recent work to build artificial model membranes with defined nanoscale topographic and topological properties to control anomalous behaviour. Mark exploits a combination of single-molecule Total Internal Reflection Fluorescence and Interferometric Scattering microscopies to characterise diffusion using single-particle tracking that spans over four orders of magnitude in time. ![]() Professor Mark Wallace, King's College London, UK
![]() Professor Mark Wallace, King's College London, UKMark studied Chemical Physics as an undergraduate at the University of Bristol, followed by a PhD in Chemistry at the University of Cambridge under the supervision of Professor David Klenerman. He was awarded the 2002 Gregorio Weber International Prize in Biological Fluorescence for this work. Mark then spent 2 years as a postdoctoral fellow at Stanford University, working with Professor Richard Zare. He returned to the UK in 2002 to undertake a second postdoctoral position at the National Institute for Medical Research with Dr Justin Molloy, before moving to Oxford in 2005 as a Royal Society University Research Fellow. He was subsequently appointed as a University lecturer and fellow of Wadham College in 2006. He joined King’s in 2016 as part of its expansion of Chemistry. In 2009 Mark was appointed to the steering committee of the British Biophysical Society. Patents arising from his work have also been licensed in the UK Mark has also been active in raising awareness of his group’s research beyond the lab, including video podcasting and participation in the 2014 “I’m a scientist get me out of here” competition. He was awarded the RSC Norman Heatley Award in 2015 in recognition of his work. He was made a Fellow of the RSC in the same year. |
11:25 - 11:50 |
Mimicking membrane scission in vitro
Eukariotic cells are highly compartmentalized to achieve local special functions, but nevertheless have to exchange lipids and proteins between compartments and with the exterior of the cell. Thus, traffic intermediates (vesicles, tubules) have to be formed and detached from donor membranes to be transported and deliver their cargos and membrane to an acceptor compartment. Cell compartmentalization thus works hand-in-hand with membrane scission to allow for these exchanges. Reconstituted membrane systems have been instrumental to reveal mechanisms that drive membrane scission of bud neck in vivo, such as constriction by the ATPase dynamin involved in endocytosis or by line tension at the edge of membrane domains. Reconstitution approaches have similarly allowed recently to reveal a new scission mechanism, not based on constriction but on the friction between a protein scaffold and the membrane that produces a viscoelastic-like response and a scission when membrane tension exceeds lysis tension: Friction-Driven Scission (FDS). Professor Bassereau will show how this mechanism was shown using a combination of reconstituted systems and theoretical modelling. ![]() Professor Patricia Bassereau, Institut Curie, France
![]() Professor Patricia Bassereau, Institut Curie, FrancePatricia Bassereau is currently CNRS Directrice de Recherche (equivalent to professor) at the Institut Curie in Paris where she is the leader of the group 'Membranes and cellular functions'. She started her carrier in Soft Matter in Montpellier (GDPC) and spent one year as a visiting scientist at the IBM Almaden Center (San Jose, USA). She moved to the Institut Curie in 1993 to work on questions related to 'Physics of the cell'. She develops a multidisciplinary approach, largely based on synthetic biology and on the development and study of biomimetic systems, as well as quantitative mechanical and microscopy methods to understand the role of biological membranes in cellular functions. Additionnally, she studies the mechanics of single filopodia in living cells, and in vitro the generation of cell protrusions. |
Chair
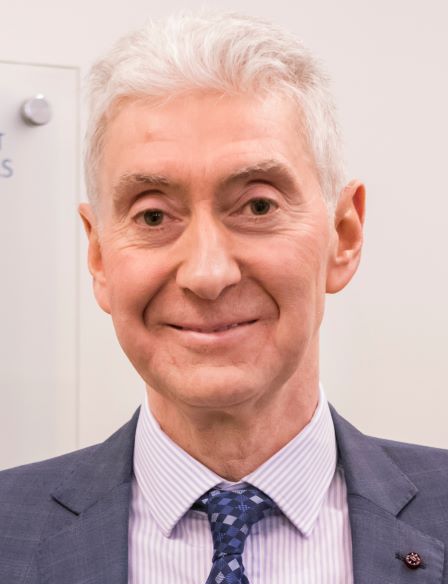
Professor Stephen Mann FRS, University of Bristol, UK
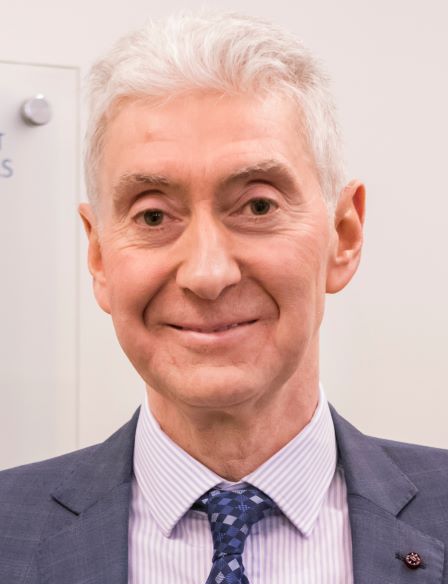
Professor Stephen Mann FRS, University of Bristol, UK
Stephen Mann is Professor of Chemistry, co-Director of the Max Planck Bristol Centre for Minimal Biology, and Director of the Centres for Organized Matter Chemistry and Protolife Research at the University of Bristol, UK. He is distinguished for contributions to biomineralization, bioinspired materials chemistry and protocell research. Professor Mann is a Fellow of the Royal Society UK, Fellow of the Royal Society of Chemistry, UK, and a Member of Academia Europaea and the European Academy of Sciences and Arts. He has received awards from the Royal Society of Chemistry (de Gennes Prize; SCF French-British Prize; Nyholm Medal) and was a visiting professor at the College de France and Harvard University. He was awarded the Royal Society Davy Medal in 2016. Professor Mann’s current research focuses on the development of synthetic protobiology and the transition from non-living to living matter.
14:10 - 14:35 |
Adaptive compartments with life-like behaviour
Compartmentalization is generally regarded as one of the key prerequisites for life. In living cells, not only the cell itself is a compartment, with its properties controlled by the semipermeable cell membrane, but also the organelles play a crucial role in protecting and controlling biological processes. To better understand the role of compartmentalization, there is clear need for model systems that can be adapted in a highly controlled fashion, and in which life-like properties can be installed. Polymer-based compartments are robust and chemically versatile, and as such are a useful platform for the development of life-like compartments. In this contribution both an artificial organelle and cell system will be discussed. The artificial organelles are composed of biodegradable amphiphilic block copolymers that self-assemble into vesicular structures. These so-called polymersomes are loaded with enzymes and are semi-permeable for small molecule substrates. Upon introduction in living cells, they affect metabolic pathways as artificial organelles. A different type of polymersome is created via a shape change process in which a bowl-shaped structure is obtained. Within the cavity of the bowl enzymes are loaded which provide the nanostructure with motility upon conversion of chemical energy into kinetic energy. The synthetic cell platform is composed of a complex polymer coacervate, stabilized by a biodegradable block copolymer. The specific feature of the polymer membrane is its semipermeable character. Enzymes inside the protocell can therefore still be reached by their substrates, and small molecule products can be excreted. This allows protocell communication with this robust synthetic platform. ![]() Professor Jan Van Hest, Technische Universiteit Eindhoven, The Netherlands
![]() Professor Jan Van Hest, Technische Universiteit Eindhoven, The NetherlandsJan van Hest obtained his PhD from Eindhoven University of Technology in 1996 with prof. E.W. Meijer. He subsequently worked as a postdoc with prof D.A. Tirrell at the University of Massachusetts. In 1997 he joined the chemical company DSM in the Netherlands. In 2000 he was appointed full professor in Bio-organic chemistry at Radboud University Nijmegen. As of September 2016 he holds the chair of Bio-organic Chemistry at Eindhoven University of Technology. He has won a number of awards, amongst which a Dutch Science Foundation VICI grant (2010) and an ERC Advanced grant (2016). In 2012 he was one of the main grantees of the 27 M€ Gravitation Program on functional molecular systems (FMS). He currently coordinates the Horizon 2020 ITN Network Nanomed, dedicated to developing smart delivery carriers in medicine. The group’s focus is to develop well-defined compartments for nanomedicine and artificial cell research. |
|
---|---|---|
14:45 - 15:10 |
Biomimetic synthesis of artificial magnetosomes
Magnetic nanoparticles (MNPs) have many applications, particularly over a range of emerging biomedicine (diagnostic and therapeutic). For such applications the MNPs are required to be biocompatible and have a mono-disperse size and shape distribution to ensure their magnetic behaviour is consistent, which can require chemical synthesis under harsh and toxic conditions, which may compromise biocompatibility. Magnet bacteria synthesis nanoparticles of magnetite within lipid vesicles (known as magnetosomes) within their cells. Magnetosomes show great potential for such biomedical applications, however, magnetosomes themselves may not be ideal with respect to economy of production and adaptability for different specifications. Sarah Staniland’s group have therefore taken inspiration from magnetic bacteria to create artificial magnetosome-like theranostic magnetic nanovesicles in situ under mild reaction conditions. Here Sarah describes how her group mimics the magnetosomes with iron ions imported into base filled vesicles to form a range of magnetovesicles, from magnetic nanoparticles embedded in the membrane to magnetic nanoparticle core liposome similar to a native magnetosome. Sarah will show how different magnetovesicles vary in their hyperthermic and MRI response and demonstrate how the group can control and tune the particle size and number to tailor them for different applications. ![]() Dr Sarah Staniland, University of Sheffield, UK
![]() Dr Sarah Staniland, University of Sheffield, UKSarah S Staniland obtained her undergraduate degree from the University of Edinburgh (MChem 2001) and stayed in chemistry there to complete a PhD in magnetic materials under the supervision of Profs. Andrew Harrison and Neil Robertson (2005). After her PhD she won a prestigious independent EPSRC life science interface fellowship (2005-2008) at the University of Edinburgh where she initiated the research she is currently active in. This helped her transition from the chemical material sciences to interdisciplinary work at the interface with biology. She took this opportunity to live and work in various places globally, from Cape Town to Tokyo, forming lasting collaboration with key leaders in their field. She moved to the University of Leeds to take up a Lectureship in Bionanoscience in the School of Physics and Astronomy where she was promoted to Associate Professor (2008-2013). She has now moved to Sheffield as a Senior Lecturer in Bionanoscience recently promoted to Reader (2016). Her research interests centres around the biomimetic synthesis of nanomaterials, particularly nanomagnetic particles and how they are produced within magnetic bacteria. From a basis of material chemistry and PhD in magnetic materials, Sarah has moved into a multidiscipline approach of using biology to control material synthesis. Key to this is mimicing the biomineralisaiton in magnetosomes to form precise mangetic nanoparticles within vesicles. |
|
15:20 - 15:50 | Tea | |
15:50 - 16:15 |
Model systems for membraneless subcellular organelles: Compartmentalization of biomolecules and reactions by liquid-liquid phase coexistence
Biological cells are highly organized with numerous subcellular compartments, many of which lack membranous boundaries such as those that surround organelles. Christine Keating’s group are developing simple experimental models for these membraneless organelles based on liquid-liquid phase separation, which is a common phenomenon in aqueous solutions of macromolecules. Solutes such as ions, small molecules, and biopolymers can become compartmentalized by partitioning into one of the phases. The group is studying mechanisms for, and consequences of, this type of compartmentalization using a variety of simple model systems composed of phase-separating aqueous polymer solutions. Through these types of studies, Christine hopes to uncover underlying physiochemical mechanisms in cellular organization and to identify new avenues for biomimetic systems for applications in biotechnology and materials science. For example, compartmentalization of catalysts and/or reactants into polymer-rich droplets can lead to control over the sites and rates of reactions. This approach has led to increased ribozyme reaction rates and development of liposome-stabilized water-in-water emulsions that act as artificial mineralizing vesicles. ![]() Professor Christine Keating, Penn State University, USA
![]() Professor Christine Keating, Penn State University, USAChristine D Keating received her BS in Biology and Chemistry from St Francis College (Loretto, PA) in 1991 and her PhD in Chemistry from Pennsylvania State University in 1997. She is currently a Professor of Chemistry at Pennsylvania State University and is affiliated with the Materials Research Institute and the Huck Institute for Life Sciences. She has been honored as a Sloan Research Fellow, Beckman Young Investigator, Camille Dreyfus Teacher-Scholar, and recipient of an NSF CAREER award. Dr Keating was named the Unilever Outstanding Young Investigator in Colloid and Surfactant Science (2004), the Bodil Schmidt Nielsen Fellow & Bioengineer in Residence at Mount Desert Island Biological Laboratory in 2010, and a Fellow of the American Association for the Advancement of Science in 2014. Dr Keating is author or coauthor of over 100 scientific articles. Her research interests combine materials science, colloid chemistry, and cell biology. |
|
16:25 - 16:50 |
Protein design in the cell: towards a synthetic proteome
Protein design, that is, the construction of entirely new protein sequences that fold into prescribed structures, has come of age. It is now possible to design proteins de novo using simple rules of thumb or computational design methods. The designs can be made rapidly via peptide synthesis or the expression of synthetic genes; and the resulting proteins can usually be characterised all the way through to high-resolution X-ray crystal structures. Contemporary questions in the protein-design field include: What do we do with these new-found skills? What protein structures and functions do we target? How far can we move past the confines of natural protein structures and functions? And, how can we take protein design from an exercise largely done in silico and in vitro into a truly synthetic biology and take it in vivo? This talk will address these questions with reference to simple through to complex and functional protein designs that we have explored over the past 5–10 years. These use a straightforward protein structure, called the alpha-helical coiled coil, which are bundles of 2 or more alpha helices found in many protein-protein interactions. As such it provides an excellent basis for building proteins from the bottom up. The vast majority of coiled-coil designs have been based on simple rules of thumb learnt from natural proteins or derived empirically through experiment. These rules relate sequence to structure to guide the specification of coiled-coil oligomerization state, strand orientation, partner selection, and, to some extent, stability. This has been extremely informative and productive, and design and engineering is probably more advanced for coiled coils than for any other protein structure. However, to move past the low-hanging fruit of coiled-coil design, and into the so-called dark matter of protein structures, we will all have to learn new tricks. To address this Professor Woolfson has begun to tackle coiled-coil design parametrically using computational methods. Professor Woolfson has developed easy-to-use computational modelling tools and a more-sophisticated suite of programs called ISAMBARD that allow the rapid generation and optimisation of protein designs in silico. The first part of the talk will describe how a serendipitous discovery of a 6-stranded alpha-helical barrel led his group to develop these computational methods, and how they have used these to deliver entirely new non-natural protein structures predictably. It will show the utility of this approach to make water-soluble protein-like barrels, which the group has engineered to form materials, bind small molecules, and catalyse simple reactions. Secondly, Professor Woolfson will demonstrate how we might take protein design in vivo with recent work with the Warren lab (Kent) and the Verkade lab (Bristol). He has engineered hybrids of a de novo heterodimer and a natural component of bacterial microcompartments. When expressed in E. coli, the hybrid assemblies to form a cytoscaffold that permeates the cells, and can act as a support for the co-localisation of functional enzymes. ![]() Professor Dek Woolfson, University of Bristol, UK
![]() Professor Dek Woolfson, University of Bristol, UKProfessor Dek Woolfson took his first degree in Chemistry at the University of Oxford, UK. He then did a PhD at the University of Cambridge followed by post-doctoral research at University College London and the University of California, Berkeley. After 10 years as Lecturer through to Professor of Biochemistry at the University of Sussex, he moved to the University of Bristol in 2005 to take up a joint chair in Chemistry and Biochemistry. Dek’s research has always been at the interface between chemistry and biology, applying chemical methods and principles to understand biological phenomena. Specifically, his group is interested in the challenge of rational protein design, and how this can be applied in synthetic biology and biotechnology. His particular emphasis is on making completely new protein structures and biomaterials for applications in cell biology and medicine. In 2011, Dek became the first recipient of the Medimmune Protein and Peptide Science Award of the Royal Society of Chemistry; and in 2014 he received a Royal Society Wolfson Research Merit Award. Dek is Director of the Bristol BioDesign Institute and Co-Director of BrisSynBio, a £13.6M BBSRC/EPSRC-funded Synthetic Biology Research Centre. |
Chair
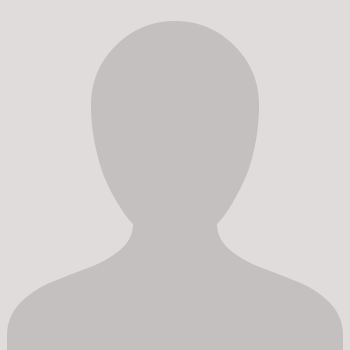
Professor Oscar Ces, Imperial College London, UK
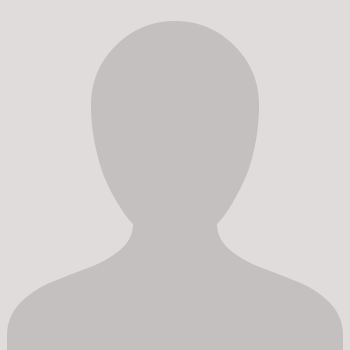
Professor Oscar Ces, Imperial College London, UK
Professor Oscar Ces, a Professor in Chemistry at Imperial College London is a specialist in soft condensed matter, chemical biology, microfluidics, artificial cells, single cell analysis and lipid membrane mechanics. He has active research programmes with industry including collaborations with AstraZeneca plc, GSK plc, P&G plc and Syngenta plc. His current roles include: Co-Director of the Imperial College Advanced Hackspace, Co-Director of FABRICELL, Director of Development and Advancement (Chemistry), Director of the Institute of Chemical Biology (ICB), Director of the EPSRC ICB Centre for Doctoral Training and Co-Director of the Membrane Biophysics Platform. He is also a member of the steering committees for Agri-Net and the IC Nutrition and Food Network.
09:00 - 09:25 |
Regenerating the nuclear envelope during exit from mitosis
During cell division, as well as separating their duplicated genomes, cells must also deconstruct, separate and then reconstruct many of their cytoplasmic organelles. Mammalian nuclei are surrounded by a double-membraned organelle called the nuclear envelope that is continuous with the endoplasmic reticulum. During mitotic exit, sheets of ER envelope the forming daughter nuclei and become fused together to generate a sealed nuclear envelope. In addition to its role in membrane abscission during cytokinesis, viral budding, endosomal sorting and plasma membrane repair, the endosomal sorting complex required for transport-III (ESCRT-III) machinery has recently been shown to participate in nuclear envelope sealing during mitotic exit. Nuclear envelope localisation of ESCRT-III is dependent upon the ESCRT-III component CHMP7 and the inner nuclear membrane protein LEM2. However, it is unclear how ESCRT-III actually engages nuclear membranes. Here, Dr Carlton shows that the N-terminus of CHMP7 acts as a novel membrane-binding module. This membrane-binding ability allows CHMP7 to bind to the endoplasmic reticulum (ER), an organelle continuous with the NE, and provides a platform to direct NE-recruitment of ESCRT-III during mitotic exit. Dr Carlton also identifies novel activities in the C-terminus of CHMP7 that restrict its activity to the inner-nuclear membrane and help us understand how this complex can help regenerate the nucleus. Dr Carlton finds that mutations that compromise CHMP7 function also prevent assembly of downstream ESCRT-III components at the reforming NE and proper establishment of post-mitotic nucleo-cytoplasmic compartmentalisation. These data identify a novel membrane-binding activity within an ESCRT-III subunit that is essential for post-mitotic nuclear regeneration. ![]() Dr Jeremy Carlton, KCL and Francis Crick Institute, UK
![]() Dr Jeremy Carlton, KCL and Francis Crick Institute, UKJeremy obtained a BA in Natural Sciences from Cambridge University and then a PhD from the University of Bristol, where he examined membrane trafficking pathways regulated by the phosphoinositide-binding family of Sorting Nexins in Pete Cullen’s lab. After his PhD, he moved to the laboratory of Professor Juan Martin-Serrano in the Infectious Diseases department of King’s College London to examine how HIV-1 hijacks the ESCRT-machinery to allow its release from cells. Here, Jeremy described a novel and unexpected role for the ESCRT-machinery in cytokinesis and characterised the involvement of ESCRT-III proteins in an Aurora-B regulated abscission checkpoint. After his postdoc, Jeremy moved to the Division of Cancer Studies at King’s College London as a Wellcome Trust Research Career Development Fellow and then a Wellcome Senior Fellow. In Jeremy’s lab, the group continue their focus on membrane trafficking machineries and have described a novel role for the ESCRT-machinery in rebuilding the nuclear envelope during cell division. |
|
---|---|---|
09:35 - 10:00 |
Synthetic biology of minimal cellular systems
In recent years, biophysics has accumulated an impressive selection of cutting-edge techniques to analyse biological systems with ultimate sensitivity and precision, down to the single molecule level. However, a strictly quantitative application of most of these techniques in living cells or organisms has been extremely challenging, because of the enormous complexity and redundancy of cellular modules and elements. The more physiological a system under study, the harder it is to define a manageable number of relevant control parameters. This renders it necessary to accumulate ever more sophisticated techniques and assays in order to master a single biological problem, and thus, often extends experiments and publications in the life sciences to hardly manageable sizes. An alternative approach is to limit the methodological toolbox in a biological study without sacrificing the biophysical standards of quantitation. Instead, the biological phenomenon will have to be reduced to its fundamental features by reconstituting it in a bottom-up approach. The strive for identifying such minimal biological systems, particularly of subcellular structures or modules, has in the past years been very successful, and crucial in vitro experiments with reduced complexity can nowadays be performed, e.g., on reconstituted cytoskeleton and membrane systems. As a particularly exciting example for the power of minimal systems, we recently demonstrated the self-organization of MinCDE, essential proteins of the bacterial cell division machinery, leading to a protein-based pacemaker and spatiotemporal cue for downstream events, such as the positioning of divisome proteins. In her talk, Petra Schwille will discuss some recent results of her group’s work on membrane-based systems, using single molecule optics and biological reconstitution assays. Petra will further discuss the perspective of assembling a minimal system to reconstitute cell division. ![]() Professor Petra Schwille, Max Planck Institute of Biochemistry, Germany
![]() Professor Petra Schwille, Max Planck Institute of Biochemistry, GermanyPetra Schwille is Director at the Max Planck Institute of Biochemistry in Martinsried near Munich, Germany, and Honorary Professor at the LMU Munich. She studied physics and philosophy in Stuttgart and Göttingen, and graduated 1993 with Diploma in Physics at the Georg August University, Göttingen. She obtained her PhD in 1996 performed at the MPI for Biophysical Chemistry, Göttingen. After a postdoctoral stay at Cornell University, Ithaca, NY, she returned to the MPI Göttingen as a research group leader. In 2002, she accepted a Chair of Biophysics at the TU Dresden. Since 2011, she is heading the department Cellular and Molecular Biophysics at the MPI of Biochemistry. Her scientific interests range from single molecule biophysics to the synthetic biology of reconstituted systems. |
|
10:40 - 11:05 |
Buckling of an epithelium growing under spherical confinement
Many organs, such as the gut or the spine are formed through folding of an epithelium. While genetic regulations of cell fates leading to epithelium folding have been investigated, mechanisms by which forces sufficient to deform the epithelium are generated are less studied. Here, Aurelien Roux shows that cells forming an epithelium on to the inner surface of spherical elastic shells protrude inward while growing. By measuring the pressure and local forces applied onto the elastic shell, Aurelien shows that this folding is induced by compressive stresses arising within the epithelial layer: while growing under spherical confinement, epithelial cells are subjected to lateral compression, which induces epithelium buckling. While several fold initiations can be observed within one capsule, final shapes often show a single fold. These findings are recapitulated by an analytical model of the epithelium buckling from which the Roux group can estimate local compressive forces and rigidity. As proposed for gastrulation or neurulation, this study shows that forces arising from epithelium proliferation are sufficient to drive epithelium folding. ![]() Dr Aurelien Roux, University of Geneva, Switzerland
![]() Dr Aurelien Roux, University of Geneva, SwitzerlandAs a PhD student (Curie Institute, Paris, 2000-2004) he worked between cell biologists (Bruno Goud’s lab) and physicists (Patricia Bassereau’s lab) studying how membrane properties influence various stages of membrane traffic. He found that Lo lipids, characterized by an increased bending rigidity of the liquid-ordered (Lo) phase they form compared to liquid-disordered (Ld) phase, were partially excluded from membrane tubules pulled from giant liposomes by molecular motors. This is due to the increased energy requirement for incorporating such lipids into curved structures (Roux et al., EMBO J, 2005). Even though postulated in the 80s, this study is the first experimental proof of curvature-dependent lipid sorting. He went on with a post-doctoral work in Pietro de Camilli’s laboratory (2004-2007, Yale, USA). He created an assay with which he could monitor membrane fission induced by Dynamin (Roux et al., Nature 2006). After addition of GTP, he observed the contraction of the tubules and the formation of super-coils. This suggested a twisting activity of dynamin that he confirmed by attaching a small bead that rotated around the dynamin tubules during GTP hydrolysis. This study was the first study to show full reconstitution of membrane fission in an in vitro assay. More recently, as CNRS staff scientist (2007-2010) and assistant professor of Biochemistry, Geneva (2010-present), he showed, together with his first PhD student Sandrine Morlot, that dynamin mediated-membrane fission occurs at the edge of its helix, explaining why constriction is required but not sufficient for fission (Morlot et al. Cell 2012). In 2015, he has been promoted to associate professor the University of Geneva, and has in the meanwhile focused on two other protein assemblies, the ESCRT-III complex and the clathrin coat. He showed that clathrin assembly can be counteracted by membrane tension and rigidity (Saleem et al., Nat Commun. 2015), and that ESCRT-III proteins assemble into a spiraling filament which behaves like a spiral spring to deform the membrane (Chiaruttini et al., Cell 2015). Roux is a recipient of several prizes, including the 2013 Friedrich Miescher prize from the Swiss Biochemistry Society, and of an ERC grant. |
|
11:15 - 11:40 |
Signalling reactions on membrane surfaces: the roles of space, force, and time
Most intracellular signal transduction reactions take place on the membrane. The membrane provides much more than just a surface environment on which signalling molecules are concentrated. There is a growing realization that multiple physical and chemical mechanisms allow the membrane to actively participate in the signalling reactions. Using a combination of single molecule imaging and spectroscopic techniques, Professor Jay Groves’ research seeks to directly resolve the actual mechanics of signalling reactions on membrane surfaces both in reconstituted systems and in living cells. These observations are revealing new insights into cellular signalling processes as well as some unexpected functional behaviours of proteins on the membrane surface. The Groves’ lab has recently discovered a type of signalling reaction phenomenon that enables geometrical features of the membrane surface to couple directly to the outcome of a signalling process. ![]() Professor Jay Groves, University of California, Berkeley, USA
![]() Professor Jay Groves, University of California, Berkeley, USAJay Groves obtained a BS in Physics and Chemistry from Tufts University and a PhD in Biophysics from Stanford University. He has been a Professor in the department of Chemistry at the University of California, Berkeley since 2010. Jay has a broad background in physical chemistry and materials science as well as a long history of applying these skills to solve important problems in cell membrane biology and signal transduction. He invented the patterned supported membrane technology as well as its integration with living cells to create spatial mutations, in which the geometrical arrangement of molecules inside otherwise chemically identical living cells is altered in a controlled ways. Additionally, his research program has emphasized the advancement of optical tools including fluorescence spectroscopy, microscopy, and single molecule imaging to quantitatively analyze biological signaling processes on both reconstituted and living cell membranes. Jay’s research group is correspondingly diverse with students and postdocs from Physics, Chemistry, Biology and Engineering all working as a team. He has served as PI or co-PI on extramurally funded programs from NIH, NSF, DOE, DOD and NRF (Singapore) as well as a number of private foundations. |
|
11:50 - 12:15 |
Harnessing Nature's ability to create membrane compartmentalisation
A biological cell can be thought of as a complex chemical reactor where vast numbers of interactions are simultaneously taking place. To prevent unwanted cross-talk and interference within the ‘noise’ of all these concurrent chemical pathways, a cell compartmentalises these processes localizing different functions within individual membrane-bound structures (organelles). Confinement of chemical processes also allows a cell to maintain incompatible environments that are optimal for each organelle's function, which would not be possible within a single ‘pot’. If we are to mimic this complexity within synthetic ‘nanoreactors’, we need to develop ways of mimicking cellular compartmentalisation within synthetic structures. Here, Dr Barbara Ciani and Dr Paul Beales will show that it is possible to create multi-compartment architectures, in vitro, using a purified membrane remodelling protein complex. Barbara and Paul will also show how this in vitro system allows us to learn what controls the membrane shaping action of these proteins and therefore regulate the encapsulation of cargo. ![]() Dr Paul Beales, University of Leeds, UK
![]() Dr Paul Beales, University of Leeds, UKPaul is an Associate Professor in physical chemistry at the University of Leeds. With a background in soft matter and biological physics, his current research interests are encompassed by the physical properties and applications of biomimetic membranes. Specific recent and current research topics that contribute to the synthetic biology toolbox for development of artificial cells include membrane texturing through phase separation, artificial DNA adhesion receptors, hybrid lipid-polymer membrane systems for enhanced stability and lifetime, encapsulation of feedback-responsive systems and controlled generation of eukaryote-like membrane architectures. ![]() Dr Barbara Ciani, University of Sheffield, UK
![]() Dr Barbara Ciani, University of Sheffield, UKDr Ciani obtained a Laurea in Chemistry at University "La Sapienza" in Rome (1997). This was followed by a DPhil in Biochemistry at the School of Biological Sciences of the University of Sussex (2002). She was a postdoctoral research associate at the Department of Chemistry of the University of Nottingham (2001-2003), and at the Wellcome Trust centre for Cell Matrix research of the University of Manchester (2004-2008). In 2008, she was appointed as a Lecturer in Biophysical Chemistry at the Department of Chemistry of the University of Sheffield. |
Chair
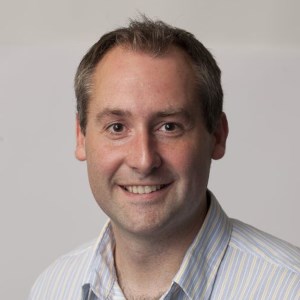
Dr Paul Beales, University of Leeds, UK
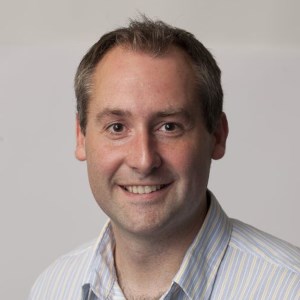
Dr Paul Beales, University of Leeds, UK
Paul is an Associate Professor in physical chemistry at the University of Leeds. With a background in soft matter and biological physics, his current research interests are encompassed by the physical properties and applications of biomimetic membranes. Specific recent and current research topics that contribute to the synthetic biology toolbox for development of artificial cells include membrane texturing through phase separation, artificial DNA adhesion receptors, hybrid lipid-polymer membrane systems for enhanced stability and lifetime, encapsulation of feedback-responsive systems and controlled generation of eukaryote-like membrane architectures.
13:45 - 14:10 |
A cellular transition to evolutionary dynamics
Complex chemical systems contain not only diverse chemistry, but also couple molecular processes to the environment via dynamical interactions. Whereas the formation of dissipative chemical fluxes in polymer systems, soft materials, or inorganic crystal gardens are controlled by macroscopic dynamics that can be fine-grained, the exploration of the development of complexity at the molecular level has not been widely explored. In this talk Professor Cronin will present his group’s research in this area that aims to drive the development of complexity in molecular systems by coupling them to non-equilibrium dynamics associated with proto-cell systems. The ultimate aim is to explore how evolutionary dynamics and complex molecular machinery might emerge giving a new avenue for the design of molecular machines and autonomous life-like systems. To explore this Professor Cronin has developed a series of fully autonomous chemical robots aim to look at flows, proto-cells, and self-replicating chemistry. ![]() Professor Lee Cronin, University of Glasgow, UK
![]() Professor Lee Cronin, University of Glasgow, UKLeroy (Lee) Cronin FRSE was born in the UK in 1973 was appointed to be Regius Professor of Chemistry in Glasgow in 2013 after being a professor (2009 & 2006) and reader in Glasgow since 2002. Between 2000-2002 he was a lecturer at the University of Birmingham. Alexander von Humboldt research fellow (Uni. of Bielefeld); 1997-1999: Research fellow (Uni. of Edinburgh); 1997: Ph.D. Bio-Inorganic Chemistry, Uni. of York; 1994 BSc. Chemistry, First Class, Uni. of York. Prizes include 2015 RSC Tilden Prize, 2013 BP/RSE Hutton Prize, 2012 RSC Corday Morgan, 2011, Election to the Royal Society of Edinburgh in 2009. His research has three main aims 1) the construction of an artificial life form / work out how inorganic chemistry transitioned to biology / searching for new life forms; 2) the digitization of chemistry; and 3) the use of artificial intelligence in chemistry including the construction of ‘wet’ chemical computers. |
---|---|
14:20 - 14:45 |
Microfluidic technologies for the bottom-up construction of artificial cells
This talk will outline microfluidic strategies for bottom-up synthetic biology that are being used to construct multi-compartment artificial cells where the contents and connectivity of each compartment can be controlled. These compartments are separated by biologically functional membranes that can facilitate transport between the compartments themselves and between the compartments and external environment. These technologies have enabled us to engineer multi-step enzymatic signalling cascades into the cells leading to in-situ chemical synthesis and systems that are capable of sensing and responding to their environment. In addition, we have developed hybrid systems fusing living and non-living components to generate higher order systems. Finally, this talk will provide an overview of microfluidic single cell analysis tools for counting the protein copy number in single cells and artificial cells. ![]() Professor Oscar Ces, Imperial College London, UK
![]() Professor Oscar Ces, Imperial College London, UKProfessor Oscar Ces, a Professor in Chemistry at Imperial College London is a specialist in soft condensed matter, chemical biology, microfluidics, artificial cells, single cell analysis and lipid membrane mechanics. He has active research programmes with industry including collaborations with AstraZeneca plc, GSK plc, P&G plc and Syngenta plc. His current roles include: Co-Director of the Imperial College Advanced Hackspace, Co-Director of FABRICELL, Director of Development and Advancement (Chemistry), Director of the Institute of Chemical Biology (ICB), Director of the EPSRC ICB Centre for Doctoral Training and Co-Director of the Membrane Biophysics Platform. He is also a member of the steering committees for Agri-Net and the IC Nutrition and Food Network. |
14:55 - 15:25 | Tea |
15:25 - 15:50 |
Collective behaviour in synthetic protocell communities
Recent progress in the chemical construction of micro-compartmentalized colloidal objects comprising integrated biomimetic functions is paving the way towards rudimentary forms of artificial cell-like entities (protocells) for modelling complex biological systems, exploring the origin of life, and advancing future proto-living technologies. Although several new types of protocells are currently available, the design of synthetic protocell communities and investigation of their collective properties has received little attention. In this talk, Professor Mann reviews some recent experiments undertaken in his laboratory that demonstrate simple forms of higher-order dynamic behaviour in synthetic protocells. Professor Mann will discuss four new areas of investigation: (i) enzyme-powered motility and collective migration in buoyant organoclay/DNA protocells; (ii) artificial predatory behaviour in mixed populations of proteinosomes and coacervate micro-droplets; and (iii) artificial phagocytosis behaviour in a binary population of magnetic and silica colloidosomes. He will use these new model systems to discuss pathways towards chemical cognition, modulated reactivity, basic signalling pathways and non-equilibrium activation in compartmentalized artificial micro-ensembles. ![]() Professor Stephen Mann FRS, University of Bristol, UK
![]() Professor Stephen Mann FRS, University of Bristol, UKStephen Mann is Professor of Chemistry, co-Director of the Max Planck Bristol Centre for Minimal Biology, and Director of the Centres for Organized Matter Chemistry and Protolife Research at the University of Bristol, UK. He is distinguished for contributions to biomineralization, bioinspired materials chemistry and protocell research. Professor Mann is a Fellow of the Royal Society UK, Fellow of the Royal Society of Chemistry, UK, and a Member of Academia Europaea and the European Academy of Sciences and Arts. He has received awards from the Royal Society of Chemistry (de Gennes Prize; SCF French-British Prize; Nyholm Medal) and was a visiting professor at the College de France and Harvard University. He was awarded the Royal Society Davy Medal in 2016. Professor Mann’s current research focuses on the development of synthetic protobiology and the transition from non-living to living matter. |
16:00 - 16:30 | Final discussion/Overview |