Links to external sources may no longer work as intended. The content may not represent the latest thinking in this area or the Society’s current position on the topic.
Unity and diversity of ciliary systems in locomotion and transport
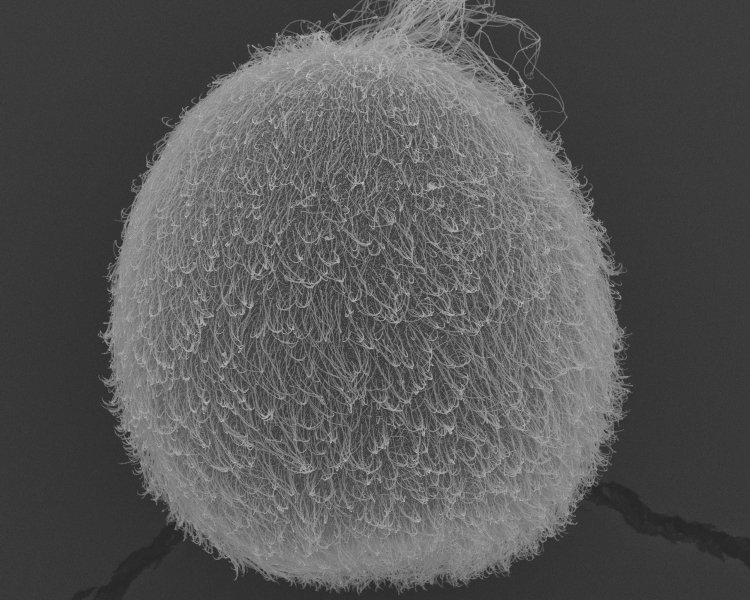
Theo Murphy international scientific meeting organised by Professor Gáspár Jékely, Professor Raymond Goldstein FRS and Dr Kirsty Wan.
Cilia are specialised cellular organelles that are united in structure and ubiquitously implicated in key life processes including sensing, locomotion, and the production of physiological flows. This meeting brought together biologists, biophysicists, and mathematicians to highlight the remarkable diversity of systems in which motile cilia fulfil vital functions, and to inspire and define novel interdisciplinary strategies for future research.
Speaker biographies and abstracts can be found below. Recorded audio of the presentations can also be found below. An accompanying journal issue for this meeting was published in Philosophical Transactions of the Royal Society B.
Attending this event
This event has taken place.
Enquiries: contact the Scientific Programmes team
Organisers
Schedule
Chair
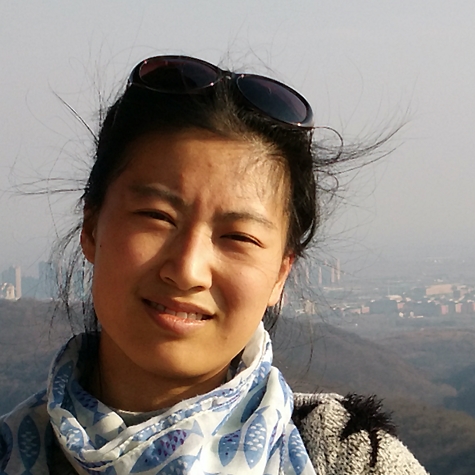
Dr Kirsty Wan, Living Systems Institute, University of Exeter, UK
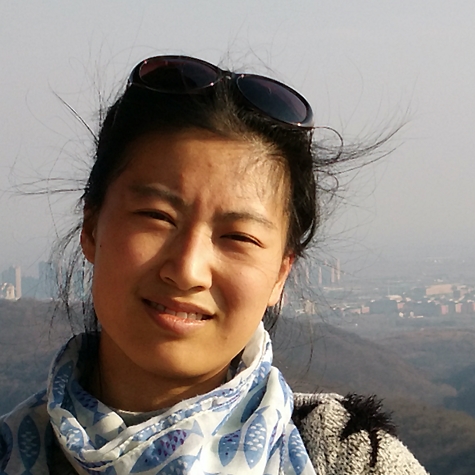
Dr Kirsty Wan, Living Systems Institute, University of Exeter, UK
Kirsty Wan is a Research Fellow and faculty member at the University of Exeter’s Living Systems Institute. Her main research interests concern the motile behaviour and dynamics of ciliated and flagellated microorganisms, with emphasis on exploring novel interdisciplinary approaches and connections to human disease. She received her undergraduate and masters degrees in mathematics from the University of Cambridge, and subsequently her PhD from the same institution, supervised by Professor Raymond Goldstein. This was followed by a postdoc in the same lab, during which she was awarded a Nevile Junior Research Fellowship from Magdalene College (2014-2017).
09:05 - 09:25 |
Mechanics of ciliary motility in Chlamydomonas
The regular, snake-like beating of sperm flagella and the breaststroke-like swimming of cilia require spatially and temporally coordinated activity of the axonemal dyneins. It is thought that coordination is mediated by stresses or strains that build up within the moving axoneme, but it is not known which components of stress or strain are involved, nor how they feed back on the dyneins. To answer this question, the group has measured the beating patterns of isolated, reactivate axonemes of the unicellular alga Chlamydomonas reinhardtii. The group compared the measurements in wild-type and mutant cells with models derived from different feedback mechanisms. They found that regulation by changes in axonemal curvature was the only mechanism that accords with the measurements. The group suggests that distortions due to bending of twisted axonemes may provide a mechanism by which the motors sense curvature. To facilitate modelling studies of axonemal beats, the group have published a simplified version of their model. ![]() Professor Joe Howard, Yale University, USA
![]() Professor Joe Howard, Yale University, USAJonathon (Joe) Howard is best known for his research on motor proteins and the cytoskeleton, and the development of techniques for observing and manipulating individual biological molecules. His research interests include the sensation of forces by specialized receptor cells, the segregation of the chromosomes during mitosis, the motility of cilia, and the branching morphogenesis of neurons. Brought up in Australia, where he studied mathematics and neurobiology, he was professor of Physiology at the University of Washington in Seattle and a Director of the Max Planck Institute of Molecular Cell Biology & Genetics in Dresden, Germany before joining the faculty at Yale. |
|
---|---|---|
09:25 - 09:30 | Discussion | |
09:30 - 09:50 |
From 3D beat to 3D swimming
Biological microswimmers are propelled by the beat of thin filaments protruding from the cell body – called cilia or flagella. One of the best-characterised flagellated microswimmers are sperm from sea urchin. To find the egg, sperm register egg-derived chemicals and translate the sensory flow into steering. Even though sperm navigation often occurs in three dimensions (3D), our knowledge is mostly restricted to the two dimensional (2D) scenario. For a better understanding of sperm navigation in 3D, the group employs caged chemoattractants to establish rapidly well-defined chemical gradients and digital holographic microscopy to resolve the 3D swimming path and the underlying 3D flagellar beat. They also apply these photonic techniques to advance our understanding of the differences among sperm species. Sperm species not only vary at the level of signalling components but also vary in shape and motility behaviours. These differences might be attributed to the different conditions under which fertilisation happens. For example, marine invertebrates, such as echinoderms or tunicates, release their gametes (eggs and sperm) into the ocean (external fertilisers). In contrast, mammalian species release sperm in the reproductive tract of the female, where they interact with the lining of the surrounding tissue (internal fertilisers). The group characterises sperm motility in 3D for internal and external fertilisers to better understand sperm behaviours across different species. ![]() Dr Luis Alvarez, Caesar, Germany
![]() Dr Luis Alvarez, Caesar, GermanyLuis Alvarez was born in Spain, Madrid, where he began in 1997 his studies in physics (Universidad Autónoma de Madrid). He continued his studies at the Università degli Studi di Firenze (Italy) during an Erasmus, and he did a Master at the Université Louis Pasteur in Strasbourg (France) in physics of soft and condensed matter. After that, Luis did his PhD in biophysics on the topic of viral infection spreading at the Laboratoire de Physique Statistique (École Normale Supérieure, Paris) under the supervision of Dr Didier Chatenay. Following his PhD, he made a Postdoc in the lab of Professor Benjamin Kaupp at the research centre Jülich (Germany) studying sperm chemotaxis. Since 2010, Luis is a group leader for the 'Biophysics of Cell Motility' at Caesar in Bonn, an institute affiliated with the Max Planck Society. His current interests are understanding cellular information-processing and navigation. |
|
09:50 - 09:55 | Discussion | |
09:55 - 10:15 |
Sperm chemotaxis – signalling at the physical limit
Sperm from marine invertebrates navigate to the egg in a chemoattractant gradient. The sperm flagellum serves as an antenna that registers the chemoattractant, as a motor that propels the cell, and as a rudder that steers sperm in the chemical landscape. Sperm are exquisitely sensitive: they can register the binding of a single chemoattractant molecule and translate binding events into a Ca2+ response that controls the flagellar beat and thereby the steering response. Professor Kaupp will discuss the cGMP-signalling pathway that endows sperm with single-molecule sensitivity. The dynamics of cellular responses, including changes in voltage, pHi, and Ca2+, is optically recorded in motile sperm. The group finds that, during navigation, sperm perform a surprisingly rich variety of computational operations; they can count, differentiate, integrate, and reset the signalling pathway. Furthermore, Professor Kaupp will decipher how such cell algebra is embodied by biochemical and electrical mechanisms. ![]() Professor U Benjamin Kaupp, Caesar, Bonn, Germany
![]() Professor U Benjamin Kaupp, Caesar, Bonn, GermanyProfessor Kaupp is Emeritus Director at the Center of Advanced European Studies and Research (caesar), an institute of the Max Planck Society, in Bonn, Germany and head of the Department of Molecular Sensory Systems. Professor Kaupp studied Chemistry at the Universities of Tübingen and Berlin. He received his PhD at the Max-Volmer-Institute of the Technical University Berlin and did his 'Habilitation' in Biophysics at the University of Osnabrück in 1983. From 1988 to 2007, Kaupp was director at the Institute of Neuroscience and Biophysics (INB) of the Helmholtz Research Centre Jülich, and from January 2008 to September 2015, he was the managing director of caesar. Professor Kaupp serves on several scientific boards. He is a Scientific Member of the Max Planck Society and a member of the German Academy of Science-Leopoldina. Kaupp`s research priorities lie in the fields of Sensory Systems, Molecular Neurobiology, Chemotaxis, Ion Channels, and Membrane Receptors. His fundamental research results have found their way into many textbooks. |
|
10:15 - 10:20 | Discussion | |
10:20 - 11:00 | Coffee | |
11:00 - 11:20 |
Cilia-driven fluid flow and particle transport in the embryonic left-right organising structure
The organised internal left-right asymmetry of the developing vertebrate embryo is initiated by asymmetric fluid dynamics (‘nodal flow’) in an enclosed transient organising structure – the ventral node in mice, Kupffer’s vesicle of zebrafish, and primary node of humans. Rotating cilia, tilted with respect to already-established axes, cause leftward transport of particles, including for example morphogen-carrying vesicles. Mechanical viscous forces may also play a critical role – either through mechanosensory effects or stress-induced exocytosis. Despite years of research, the precise nature of the conversion of the nodal flow to asymmetric development is unresolved; one key part of the challenge is that due to the microscopic nature of the system it is challenging to measure flow velocities and flow-induced stresses directly. Professor Smith will discuss methods for mathematical modelling of the flow which integrate experimental data on the shape of the organising structure with data on the cilia beat pattern and frequency. These models enable the extraction of mechanical parameters such as the time-varying flow field and the viscous forces, in addition to understanding how the flow affects the release, transport and capture of particles. This talk will focus on two specific issues: (1) cell-level modelling of stress-induced release of vesicular particles, (2) organ-level modelling of how elongation of the structure, and the convexity/concavity of the floor, modify particle paths across species, and possible implications. The open-source simulation framework, based on the NEAREST numerical scheme for the discretization of the regularized stokeslet boundary integral equation, is highly extensible to other ciliated systems. ![]() Professor Dave Smith, University of Birmingham, UK
![]() Professor Dave Smith, University of Birmingham, UKDave is Professor of Applied Mathematics at the University of Birmingham, UK with primary interests in the fluid mechanics of cilia and flagella. Following PhD study with Professors John Blake and Eamonn Gaffney on mathematical modelling of muco-ciliary transport, Dave was funded as a Medical Research Council Fellow with Centre for Human Reproductive Science with Dr Jackson Kirkman-Brown, combining fluid dynamics and high speed imaging of human sperm interacting with physiological rheology fluids. Alongside this research he pioneered 3D time-resolved models of flow in the left-right organising structure of the vertebrate embryo. Following appointment to a lectureship in 2009, he has been funded by EPSRC, BBSRC and MRC to lead projects ranging from cilia and flagellar flow, to cancer diagnosis and therapy, to pathogen detection. He is currently funded by an EPSRC Healthcare Technologies Challenge Award, and collaborates with experimental groups in Birmingham, Manchester, Nottingham, Edinburgh and Lisbon. |
|
11:20 - 11:25 | Discussion | |
11:25 - 11:45 |
Cilia and mechanosensing
Primary cilia of kidney epithelial cells are believed to act as mechanosensors for kidney-duct fluid flow. Genetic defects localising to the primary cilia are connected to polycystic kidney disease (PKD), a fatal monogenic disorder. Mechanical parameters such as flexural rigidity must modulate ciliary mechanosensation. It has been shown that there is a basal rotation component to ciliary deflection, which has the potential to affect aspects of mechanosensation. To investigate this aspect of ciliary mechanical response, the group has deflected primary cilia of Madin-Darby canine kidney (MDCK-II) cells with an optical trap and recorded their relaxation dynamics with video microscopy. Professor Schmidt presents a simple mechanical model including basal compliance, basal drag, and flexural rigidity that describes the bi-exponential relaxation behaviour seen in the data. The measured values imply a mixing of relaxation mode time scales, depending on cilia length, and suggest a possible mechanical filtering mechanism in the cilium's response. ![]() Professor Christoph Schmidt, Georg-August-Universität, Germany and Duke University, USA
![]() Professor Christoph Schmidt, Georg-August-Universität, Germany and Duke University, USAProfessor Schmidt received his Diploma in Physics and his doctorate from the Technical University Munich. Following this he held postdoctoral positions at Harvard University and The Rowland Institute for Science, after which he became Associate Professor of Physics at the University of Michigan, Ann Arbor. He was Professor of Physics at the Vrije Universiteit Amsterdam and a Member of Directorate at the Center for Research on Complex Systems. Currently Professor Schmidt is Professor of Physics at the University of Göttingen and Duke University. Professor Schmidt is a Fellow of the American Physical Society, a Member of the Göttingen Academy of Sciences and Humanities, and received an ERC Advanced Grant in 2014. |
|
11:45 - 11:50 | Discussion | |
11:50 - 12:10 |
Cilia mechanics
Motile cilia are micron-scale hair-like protrusions from epithelial cells that beat collectively to transport fluid. Individual cilia are driven into oscillatory motion by dynein molecular motors acting on an intricate structure of microtubule doublets referred to as the central axoneme. On the tissue level, the coordinated beating of cilia serves diverse biological functions, from mucociliary clearance in the airways to cerebrospinal fluid transport in the brain ventricles. Yet the relationship between cilia structure and organisation and their biological function remains elusive. Here, Professor Kanso will present a series of physics-based models that take into account minimal cilia features in order to examine: (1) the emergence of self-sustained oscillations in individual cilia; (2) the coordinated beating of neighboring cilia; and (3) the role of cilia-driven flows in transport and mixing. Professor Kanso will conclude by commenting on the implications of these models to understanding the biophysical mechanisms underlying the interaction of ciliated tissues with microbial partners. ![]() Professor Eva Kanso, University of Southern California, USA
![]() Professor Eva Kanso, University of Southern California, USAEva Kanso is a professor and the Z.H. Kaprielian Fellow in Aerospace and Mechanical Engineering at the University of Southern California. Prior to joining USC, Kanso held a two-year postdoctoral position in Computing and Mathematical Sciences at Caltech from 2003 to 2005. She received her PhD and MS in Mechanical Engineering as well as her MA in Mathematics from UC Berkeley. Kanso is the recipient of an NSF early CAREER development award in 2007, a Junior Distinguished Alumnus award from the American University of Beirut in 2014 and a USC Graduate Student Mentoring Award in 2016. |
|
12:10 - 12:15 | Discussion | |
12:15 - 12:35 |
How flagellated protists feed
Many aquatic unicellular protists are equipped with flagella and cilia that facilitate not only motility of the cells but – maybe more importantly – their capture and handling of prey. These flagellates and ciliates typically feed on sub-micron sized bacteria and other small cells, and their fundamental problem in encountering prey is that viscosity impedes predator-prey contact at the small Reynolds numbers at which they operate. The cilia or flagella essentially create a flow of water past the cell that, one way or another, facilitate predator-prey contact, often in ways that are not understood. Feeding currents also disturb the water, thereby attracting flow-sensing predators. Therefore, the flagellation as well as flagella beat patterns and kinematics in free-living protists must have evolved to optimise the fundamental trade-off between resource acquisition (near field flow) and survival (far field flow). Professor Kiørboe will first describe two examples where we now understand the fluid dynamics of prey encounter, namely filter feeding in choanoflagellates and interception feeding in dinoflagellates. He will then show flow fields created by a large range of protists with diverse flagellation and kinematics, and demonstrate the feeding-survival trade-off. Professor Kiørboe will use simple analytical as well as CFD models to provide a mechanistic understanding of the observations. Simple point-force models often provide good descriptions of far-field flow patterns and, hence, predation risk, but the near-cell flow field, essential for prey capture, relies on observations or CFD. ![]() Professor Thomas Kiørboe, Danish Technical University, Denmark
![]() Professor Thomas Kiørboe, Danish Technical University, DenmarkThomas Kiørboe is an Ocean Ecologist, graduated from the University of Copenhagen (PhD 1982; Dr. Scient. 1988), and currently a professor of Ocean Ecology at the Technical University of Demark (DTU) and director of the Centre for Ocean Life (Villum Center of Excellence) at DTU. His main interests are the functional ecology of marine plankton organisms and in achieving a mechanistic understanding of their functioning as well as on their functional roles in pelagic ecosystems. His current work focuses on the small-scale physical interactions between microscopic plankton and their fluid environment, on how these interactions governs the fundamental trade-offs between foraging and surviving, and finally in using this information to build trait-based models of pelagic ecosystems. |
|
12:35 - 12:40 | Discussion |
Chair
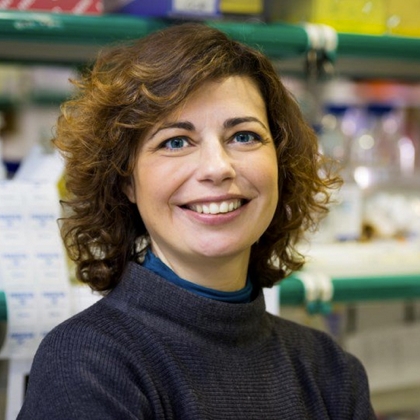
Dr Mónica Bettencourt-Dias, Instituto Gulbenkian de Ciência, Portugal
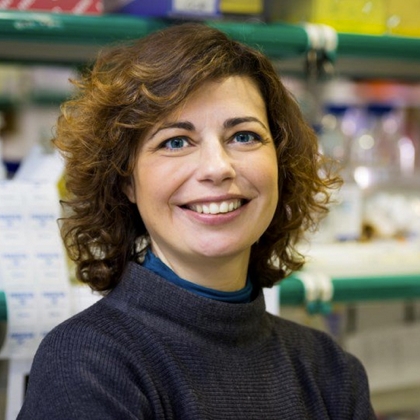
Dr Mónica Bettencourt-Dias, Instituto Gulbenkian de Ciência, Portugal
Mónica Bettencourt-Dias is Director of the Instituto Gulbenkian de Ciência since February 2018. She is also group leader at IGC since 2006. With a PhD in Biochemistry and Molecular Biology by the University College London (UK), Mónica also holds a post graduate degree in Science Communication by the Birkbeck College London. She was awarded two grants from the European Research Council in 2010 and 2015 and was also elected member of the European Molecular Biology Organisation (EMBO).
14:00 - 14:20 |
Probing the molecular organisation and function of cilia using cryo-electron microscopy
Motile cilia and flagella are highly conserved organelles composed of more than 600 different proteins that are essential for the normal development and health of many eukaryotes including humans. The group integrates cryo-electron tomography with EM-visible labelling, comparative genetics and biochemical methods to reveal the in situ 3D structure of cilia, and the mechanism and regulation of their motility. Intact ciliated cells or isolated axonemes are rapidly frozen to provide outstanding sample preservation, and both high structural and time resolution of dynamic cellular processes. This study of active sea urchin sperm flagella directly visualised the macromolecular complexes and their structural changes during flagellar beating. The group resolved distinct conformations of dynein motors and regulators, and showed that many of them are distributed in bend-direction-dependent fashion in active flagella. These results provide direct evidence for the conformational switching predicted by the 'switch-point-hypothesis'. However, they also reveal a fundamentally different mechanism of generating motility by inhibiting, rather than activating, selected dyneins causing an asymmetric distribution of force and thus bending. This provides a new understanding of the distinct roles played by various dyneins and regulators in ciliary motility and suggest a molecular mechanism for robust beating. ![]() Dr Daniela Nicastro, UT Southwestern Medical Center, USA
![]() Dr Daniela Nicastro, UT Southwestern Medical Center, USADaniela Nicastro received her PhD in Biology from the Ludwig-Maximilians University in Munich, Germany in 2000. Following 3 years in the lab of Professor Baumeister at the Max-Planck Institute for Biochemistry in Munich (1998-2001), she took a postdoctoral fellow position in the National Center for Research Resources for 3D Electron Microscopy of Cells at the University of Colorado in Boulder. From 2006-2015, she was an Assistant and then tenured Associate Professor of Biology and director of the 'Correlative Light and Electron Microcopy' (CLEM) facility at Brandeis University near Boston. Since July 2015, she is an Associate Professor at the University of Texas Southwestern (UTSW) Medical Center in Dallas with appointments in the Departments for Cell Biology and Biophysics. She has 25 years of experience in electron microscopy of cellular structures and is a leading expert in cellular cryo-electron tomography. The research interest of the Nicastro lab is focused on studying the three-dimensional structure and function of cytoskeletal assemblies, molecular motors, organelles and cells using a combination of cutting-edge methods to elucidate the structure-function relationships of macromolecular complexes in situ, ie in their native environment. |
|
---|---|---|
14:20 - 14:25 | Discussion | |
14:25 - 14:45 |
The multiple roles and addresses of VFL3/CCDC61 in Chlamydomonas
The VFL3 gene in Chlamydomonas is required for the assembly of the centrin-based distal striated fiber (DSF) that tethers the two mature basal bodies. A nonsense mutation in VFL3 leads to the loss of the DSF and to a variable number of basal bodies and cilia. VFL3 has other localisations and functions. The CCDC61 protein was crystalised. The first ~630 amino acids form seven β-sheets that dimerise and require F128 and D129 (FD). The C-terminal domain forms a coiled coil that requires 5 basic residues to dimerise. The tagged wild-type transgene localises at the basal bodies and probasal bodies as four dots. The signal is distal to BLD10/CEP135. The FD mutant transgene shows partial rescue of the mutant phenotypes. The 5E mutant, which changes the 5 basic residues to glutamates, fails to rescue the phenotypes. The basal body localisation in both mutants is diffuse, and four distinct four dots are not observed. The VFL3 protein also localises to the proximal region of the cilia. This ciliary localisation occurs in both wild-type and the FD mutant but not in the 5E mutant. The VFL3 locus encodes at least two mRNA isoforms. The short form makes a 30kD protein, and it fails to rescue the mutant phenotypes. It has an asymmetric distribution between two basal bodies. The group hypothesises that this short version may play a role in licensing basal body duplication and provide another important layer of regulation for basal body duplication/assembly that is similar to the mini CEP135. ![]() Professor Susan K Dutcher, Washington University in St Louis, USA
![]() Professor Susan K Dutcher, Washington University in St Louis, USAAs a graduate student at the University of Washington, Dutcher worked on the role of cell division cycle genes in spindle pole body duplication with Dr Lee Hartwell. As a postdoctoral fellow at Rockefeller University, she switched to cilia and centrioles in the unicellular alga, Chlamydomonas. She investigates the assembly and function of centrioles and cilia using genetics, biochemistry, light and electron microscopy, and computational biology. Stemming from comparative genomics approaches, the discovery of many cilia/centriole based diseases has helped to illustrate the incredible breath of roles that these organelles play in human health from polycystic kidney disease to respiratory infections to microcephaly to obesity. The key questions include how these microtubule-based structures are assembled and how they function to influence cellular biology, development, and human health. Recently, her lab has become interested in how the substructures in a cilium find the 'right address' in the cilia and centrioles. |
|
14:45 - 14:50 | Discussion | |
14:50 - 15:10 |
Centrosomes and cilia in development and disease
The centrosome is an important microtubule-organising centre (MTOC) in animal cells. It consists of two barrel-shaped structures, the centrioles, surrounded by the pericentriolar material (PCM), which nucleates microtubules. Centrioles can also serve as a template for cilia formation, which plays an important role in signalling and motility. Dr Bettencourt-Dias will focus this talk on the regulation of the centriole structure, in particular on variations in the structure that naturally occur to ensure the formation of different cilia types, as well as variations that occur in disease, and are associated with abnormal cell division. ![]() Dr Mónica Bettencourt-Dias, Instituto Gulbenkian de Ciência, Portugal
![]() Dr Mónica Bettencourt-Dias, Instituto Gulbenkian de Ciência, PortugalMónica Bettencourt-Dias is Director of the Instituto Gulbenkian de Ciência since February 2018. She is also group leader at IGC since 2006. With a PhD in Biochemistry and Molecular Biology by the University College London (UK), Mónica also holds a post graduate degree in Science Communication by the Birkbeck College London. She was awarded two grants from the European Research Council in 2010 and 2015 and was also elected member of the European Molecular Biology Organisation (EMBO). |
|
15:10 - 15:15 | Discussion | |
15:15 - 15:50 | Coffee | |
15:50 - 16:00 | Selected short presentation | |
16:00 - 16:20 |
Revisiting the '2' in '9+2'
Nearly all motile cilia have a '9+2' axoneme containing a central apparatus (CA), consisting of two central microtubules with projections, that is essential for motility. To date, only 22 proteins are known to be CA components. To identify new candidate CA proteins, the group used quantitative mass spectrometry to compare the proteomes of isolated axonemes of wild-type Chlamydomonas and a CA-less mutant. The group identified 44 novel candidate CA proteins, of which 14 are conserved in humans. To validate the results, five of the latter were studied more closely. They obtained mutants defective for these proteins, and rescued the mutants with genes expressing tagged versions of the proteins. Some of the mutants had distinct and novel phenotypes, demonstrating that the disrupted proteins have important and specific roles in the control of ciliary motility. The group then used structured-illumination microscopy to localise the tagged proteins within the axoneme. The group found that all five are indeed CA components; thus, it is likely that most of the other candidates also are CA proteins. Many of the 44 proteins could be assigned to specific locations within the CA. These results reveal that the CA is far more compositionally complex than previously recognised, and provide a greatly expanded knowledge base for studies to understand the architecture of the CA and how it functions. The discovery of the new conserved CA proteins will facilitate genetic screening to identify patients with a form of primary ciliary dyskinesia that has been difficult to diagnose. ![]() Professor George Witman, University of Massachussetts Medical School, USA
![]() Professor George Witman, University of Massachussetts Medical School, USAGeorge Witman is the George F. Booth Chair in the Basic Sciences and a professor in the Division of Cell Biology and Imaging, Department of Radiology, at the University of Massachusetts Medical School. He studies cilia and flagella to understand how they are assembled and function and why defects in specific ciliary proteins cause human diseases. Most of his research utilizes Chlamydomonas reinhardtii, which has major advantages for studies of flagella. Dr Witman pioneered the application of biochemical and molecular approaches to the study of Chlamydomonas flagella. His current research is focused on discovery and characterization of novel axonemal proteins, and the mechanism of intraflagellar transport (IFT). Advanced techniques used in the Witman lab include super-resolution structured-illumination microscopy, total internal reflection fluorescence microscopy, cryo-electron tomography, and mass spectrometry. |
|
16:20 - 16:25 |
Discussion
|
|
16:25 - 16:55 |
The flagellar length control system in Chlamydomonas
The motile and sensory functions of cilia and flagella require them to be of defined length, but the mechanisms by which such a complex machine is built to a particular length are not obvious. Quantitative imaging has shown that intraflagellar transport is regulated as a function of length, raising the question of how this system senses ciliary length. The group has tested three classes of mechanisms based on time of flight, diffusion, and calcium signalling, using a combination of quantitative live-cell imaging, Chlamydomonas genetics, and computational modelling. Two additional control elements, the length dependent loading of cargo and the transcriptional regulation of ciliary proteins during growth will also be discussed and integrated into a complete length control system. ![]() Professor Wallace F Marshall, University of California, San Francisco, USA
![]() Professor Wallace F Marshall, University of California, San Francisco, USAWallace Marshall studied Electrical Engineering and Biochemistry at SUNY Stony Brook, and then obtained his PhD at UCSF in the lab of John Sedat, where he analyzed interphase chromatin motion. He then moved to Yale for a postdoc with Joel Rosenbaum where he developed his interest in cilia and flagella. Since 2003 he has been a faculty member at USCF, where he now directs the Center for Cellular Construction. His lab uses a combination of imaging, computational modelling, molecular biology, and genetics, to study fundamental questions of cell geometry, in many cases using cilia and flagella as model organelles. |
|
16:55 - 17:00 | Discussion |
Chair
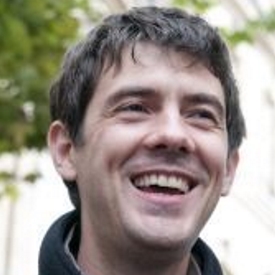
Dr Julien Vermot, Institut de génétique et de biologie moléculaire et cellulaire, France
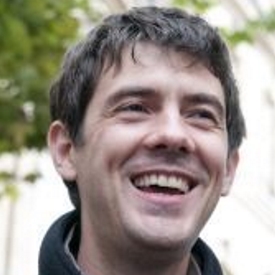
Dr Julien Vermot, Institut de génétique et de biologie moléculaire et cellulaire, France
Julien Vermot graduated from the University of Strasbourg. After a post training in the US, Julien Vermot established his own lab at the IGBMC of Strasbourg. He explores the roles of mechanical forces in the process of morphogenesis and their impact on the genetic program.
09:00 - 09:20 |
Co-opting the cell cycle to build a multiciliated cell
Cell division and differentiation depend on massive and rapid organelle remodelling. The mitotic oscillator, centered on the cyclin-dependent kinase 1–anaphase-promoting complex/cyclosome (CDK1-APC/C) axis, spatiotemporally coordinates this reorganisation in dividing cells. The group recently showed that differentiating mouse-brain multiciliated cells could also implement this mitotic clocklike regulatory circuit to orchestrate subcellular reorganisation associated with their differentiation. The postmitotic progenitors fine-tune mitotic oscillator activity to drive the orderly progression of centriole production, maturation, and motile ciliation while avoiding the mitosis commitment threshold. Insufficient CDK1 activity hinders differentiation, whereas excessive activity accelerates differentiation yet drive postmitotic progenitors into mitosis. Dr Meunier will further discuss how these postmitotic cells can redeploy and calibrate the mitotic oscillator to uncouple cytoplasmic from nuclear dynamics to drive centriole amplification. ![]() Dr Alice Meunier, CNRS, Ecole Normale Supérieure de Paris, France
![]() Dr Alice Meunier, CNRS, Ecole Normale Supérieure de Paris, FranceAfter a PhD in 2006 at Pierre and Marie Curie University, she completed a post-doctorate on motile cilia polarisation at the Ecole Normale Supérieure in Paris where she currently works. Since then, she has been studying how multiciliated cells amplify centrioles, the structures that anchor on the cell membrane to nucleate motile cilia. She developed cell culture systems and imaging tools to unveil the dynamics and molecular control of centriole amplification in mouse brain multiciliated cells. |
|
---|---|---|
09:20 - 09:25 | Discussion | |
09:25 - 09:45 |
Dynamics of adaptive phototaxis in uni- and multicellular green algae
One of the most fundamental issues in understanding the earliest stage of the evolution of multicellularity is how the simplest such organisms can exhibit coordinated behaviour in the absence of a central nervous system. Green algae belonging to the lineage that spans from Chlamydomonas to Volvox can serve as model organisms for studying many phenomena in biological fluid dynamics, and are remarkably well-suited to this issue. In this lecture, Professor Goldstein first reviews the group's earlier work on phototaxis in Volvox, which showed by experiment and theory how a rather precise tuning between the timescale of adaptation in the flagellar photoresponse and the organism's rotational period allows for accurate steering. Professor Goldstein then discusses extensions of this work to Chlamydomonas and the fascinating 16-cell organism Gonium. Taken together, these investigations shed light on the evolutionary steps involved in multicellular phototaxis. ![]() Professor Ray Goldstein FRS, University of Cambridge, UK
![]() Professor Ray Goldstein FRS, University of Cambridge, UKRay Goldstein received undergraduate degrees in physics and chemistry from MIT, and a PhD in theoretical physics from Cornell University. Following postdoctoral work at the University of Chicago and faculty positions in physics and applied mathematics at Princeton University and the University of Arizona, he moved to Cambridge University as the Schlumberger Professor of Complex Physical Systems in 2006. His research interests span from statistical physics to nonlinear dynamics and geophysics, with particular emphasis on biological physics, both theoretical and experimental. His work has been recognized by the Stephanos Pnevmatikos Award in Nonlinear Science, an Ig Nobel Prize (with Patrick Warren and Robin Ball) for explaining the shape of ponytails, the G.K. Batchelor Prize in Fluid Mechanics and the Rosalind Franklin Medal of the Institute of Physics. He is a fellow of the American Physical Society, the Institute of Physics, the Institute of Mathematics and its Applications, and the Royal Society. |
|
09:45 - 09:50 | Discussion | |
09:50 - 10:10 |
Cilia dynamics in the left-right organizer
Cilia motility in the left-right organiser (LRO) is a common feature for generating the directional flow necessary for embryonic symmetry breaking. Cilia-mediated flow operates in the LRO to control and maintain the establishment of the internal organ asymmetric polarity. In zebrafish, the LRO is called the Kupffer’s vesicle (KV). The group's goal is to understand the dynamics and the roles of biological flow during the process of symmetry breaking. The group uses live imaging techniques, cell biology and genetic analysis to characterise the physical stimuli and the molecular mechanisms that specify cell responses to flow forces. Here Dr Vermot will discuss how cilia-mediated directional flow is obtained in the KV and its biophysical origin in vivo. Cilias' spatial orientation is a key functional feature for the generation of directional flow and LR determination. Dr Vermot will also discuss the group's multi-scale live-imaging based methodology to study cilia features in 3D developed to understand the potential mechanism(s) behind cilia orientation. Overall this work provide key insights into the dynamics of motile cilia orientation during LR determination and potential mechanisms controlling it. ![]() Dr Julien Vermot, Institut de génétique et de biologie moléculaire et cellulaire, France
![]() Dr Julien Vermot, Institut de génétique et de biologie moléculaire et cellulaire, FranceJulien Vermot graduated from the University of Strasbourg. After a post training in the US, Julien Vermot established his own lab at the IGBMC of Strasbourg. He explores the roles of mechanical forces in the process of morphogenesis and their impact on the genetic program. |
|
10:10 - 10:15 | Discussion | |
10:15 - 11:00 | Coffee | |
11:00 - 11:20 |
Waves in the airways - fluid motion from motile cilia
In mammalian brains, airways and fallopian tubes, a multitude of motile cilia beat in synchrony enabling efficient fluid transport.Professor Cicuta will review our understanding of this process based on simple physical models where hydrodynamic interaction leads to large-scale collective dynamics. In the biological systems, the nature of the interaction between cilia is still under debate. Professor Cicuta will report on recent experiments showing frequency synchronisation of cilia motility in multiciliated cells from brain ventricles, studied using controlled oscillatory external flows. The group finds that the hydrodynamic forces required for synchronisation strongly depend on the number of cilia per cell. Cells with few cilia (up to 5) show high susceptibility to external flow and are entrained with flow comparable to the cilia-driven flow reported in vivo. The group proposes that hydrodynamic forces between near beating cilia are sufficient to lead their synchronisation and this reduces their effective coupling with an external flow as the number of cilia per cell increase. Professor Cicuta supports this view with simulations of a minimal model of cilia interacting hydrodynamically, showing the same trends observed experimentally. Overall the group suggests that hydrodynamic forces between cilia are sufficient as a mechanism behind the synchronisation of motile cilia in the brain of mammals. ![]() Professor Pietro Cicuta, University of Cambridge, UK
![]() Professor Pietro Cicuta, University of Cambridge, UKPietro Cicuta studied physics in Milan (Italy) and took his PhD in Cambridge (2003). His research combines single cell measuring on micro-organisms, including studies of cell size, adhesion, gene regulation; microfluidic fabrication and automation of imaging and optical measurements; quantitative mechanistic/physical modelling. Developing new experimental techniques to study the mechanics and dynamics of soft and biological systems is the heart of his research. Recent work includes studies of model bacteria and pathogens in host/pathogen interactions, physiology of ciliated epithelia and mechanics of lipid and cell membranes. He is currently PI on an ERC Consolidator grant (2013-2018) focusing on collective cilia motion in the airways, on a GCRF project developing compact microscopes (2017-2018), Co-I on an EPSRC programme grant (CAPITALS 2011-2018), on an HFSP project on bacteria gene regulation (2014-2018), an EU ITN training network BIOPOL on cell polarization (2014-2018), a GSK project on pathogens of the airways (2017-2019). Recently he was Co-I on an HFSP project on gene regulation (2010-2013), and on two other ITNs. He has also been PI on an MRC discipline hopping grant (2009) developing microfluidic devices. |
|
11:20 - 11:25 | Discussion | |
11:25 - 11:45 |
Cilia-bacteria interactions
Ciliated mucosal surfaces, including the human airways, regularly harbour microbial symbionts. The biomechanical aspects of this association process are not well understood. The group proposes that topography and kinematics of ciliated surfaces can generate diverse microhabitats for the recruitment and establishment of the microbiome. In a case study presented here, the group studied the fluid mechanics of cilia-microbiome interactions in an accessible model system, the Hawaiian bobtail squid, which reliably captures and isolates their bacterial symbiont Vibrio fischeri from inhaled seawater. In joint experimental and computational work the group reveals that two distinct cilia populations act together to produce a complex flow field that facilitates recruitment of symbionts. Specifically, the flow sieves Vibrio-sized particles into a sheltered microniche where local ciliary currents enhance the diffusion of biochemical signals and promote biofilm formation. Dr Nawroth will present these results and introduce the methods, models and metrics the group developed to assess cilia-bacteria interactions. Finally, Dr Nawroth will discuss how these findings might inform our understanding of cilia-microbiome and cilia-pathogen interactions on mucosal surfaces in other organisms, including humans. ![]() Dr Janna Nawroth, Emulate Inc, USA
![]() Dr Janna Nawroth, Emulate Inc, USADr Nawroth is conducting cross-disciplinary research in the field of bacteria-host interactions and cilia functions in aquatic organisms as well as human organ tissues to understand basic mechanisms and develop novel research tools. Currently, Dr Nawroth is Principal Investigator and Research & Development (R&D) Lead at Emulate, Inc., Boston, MA, USA. Based on their Organs-on-Chips technology, Emulate is developing a new living system that places cells within micro-engineered environments, allowing researchers to understand how different diseases, medicines, chemicals and foods affect human health. At Emulate, Dr Nawroth heads the development of the Bronchial Airway-Chip which emulates the biomechanics of ciliary mucus transport. Nawroth has more than six years' experience in academia and industry in leadership roles in basic research, discovery, and technology development. Prior to joining Emulate, she led the development of engineered cardiac muscle as a Technology Development Fellow at the Wyss Institute for Biologically Inspired Engineering at Harvard University. Nawroth earned her PhD in biology from the California Institute of Technology in collaboration with Harvard School of Engineering and Applied Sciences. She has co-authored over 15 peer-reviewed publications and multiple patents. |
|
11:45 - 11:50 | Discussion | |
11:50 - 12:10 |
Controlling ciliary polarity in the multiciliated epidermis of flatworms
Some animal species such as the planarian flatworm Schmidtea mediterranea use multiciliated cells for locomotion. Motile cilia beat in a whip-like pattern at the surface of the ventral multiciliated epidermis to allow animal translocation of planarians along the substrate. Directional locomotion requires the proper polarisation of cilia along the polarity axes of the body plan. In planarians, polarisation of cilia is mediated by the evolutionary conserved Wnt/Planar Cell Polarity pathway acting along the anterior-posterior axis, superimposed with a polarisation component towards the body edge mediated by the Fat/Dachsous pathway. Polarity proteins regulate cytoskeleton architecture to coordinate the rotational polarity of centrioles, from which cilia are assembled, with the planar polarity of the epithelium. Cytoskeletal networks are anchored at the centrioles via two types of centriolar appendages, which are essential for the proper polarisation and organization of centrioles. The group has identified proteins required for the assembly of centriolar appendages in planarians, and found that they allow organising a centriole network with remarkable left-right asymmetric properties. The ODF2 and VFL1/3 proteins are necessary for balancing left-right asymmetric forces that likely result from an asymmetric attachment of the centrioles to the cytoskeletal network. These results provide novel insights into how animals can build mirror symmetric body plans from chiral cellular constituents. ![]() Dr Juliette Azimzadeh, Institut Jacques Monod, France
![]() Dr Juliette Azimzadeh, Institut Jacques Monod, FranceJuliette Azimzadeh studied cytoskeleton organization in plants during her PhD. She later developed an interest in centrioles and cilia and joined Michel Bornens at the Curie Institute before joining Wallace Marshall at UCSF. In 2013 she started her own group at the Jacques Monod institute in Paris. Her current research focuses on understanding how centrioles polarize in response to signalling pathways using planarian flatworms as a model system. She is also interested in the evolution of the molecular architecture and function of centrioles and centrosomes. |
|
12:10 - 12:15 | Discussion | |
12:15 - 12:35 |
A matter of multiciliation: towards nervous control of motile appendages
The ability for self-movement is a primary signature of life: it is the means by which the simplest organisms migrate toward the 'good' and away from the 'bad', moving up gradients of nutrients or light, escaping from harmful chemicals or predators. Among the most evolutionarily ancient of these strategies is motility through a fluid environment using slender, actively-actuated appendages called cilia and flagella. In different species, these occur in diverse forms and configurations. However wherever multiple cilia arise, there is a clear need to coordinate these appendages effectively for swimming, navigation, or generation of directional flows past surfaces. Even the simplest unicellular organisms have been found capable of exacting precise and surprising patterns or gaits reminiscent of the breaststroke, trot, pronk, gallop etc, which are normally only associated with vertebrates. Here, the group reveals at the single-cell level the extent to which these gaits of ciliary locomotion are subject to intracellular control, producing motive behaviours that are modulated dynamically depending on perceived changes in environmental circumstances. Finally, Dr Wan shall demonstrate the functional limits of speed and sensitivity in this unique and ancient sensory-motor apparatus. ![]() Dr Kirsty Wan, Living Systems Institute, University of Exeter, UK
![]() Dr Kirsty Wan, Living Systems Institute, University of Exeter, UKKirsty Wan is a Research Fellow and faculty member at the University of Exeter’s Living Systems Institute. Her main research interests concern the motile behaviour and dynamics of ciliated and flagellated microorganisms, with emphasis on exploring novel interdisciplinary approaches and connections to human disease. She received her undergraduate and masters degrees in mathematics from the University of Cambridge, and subsequently her PhD from the same institution, supervised by Professor Raymond Goldstein. This was followed by a postdoc in the same lab, during which she was awarded a Nevile Junior Research Fellowship from Magdalene College (2014-2017). |
|
12:35 - 12:40 | Discussion |
14:00 - 14:20 |
Cilia in the developing zebrafish ear
The inner ear, which mediates the senses of hearing and balance, derives from a simple ectodermal vesicle in the vertebrate embryo. In the zebrafish, every cell of the otic vesicle is ciliated, and at least three different ciliary subtypes can be distinguished on the basis of axoneme length and motility. Long, immotile kinocilia on the sensory hair cells tether the otoliths, biomineralised aggregates of calcium carbonate and protein. Motile cilia at the poles of the otic vesicle contribute to the accuracy of otolith tethering, but neither the presence of cilia nor ciliary motility are absolutely required for this process. Instead, otolith tethering is dependent on the presence of hair cells and the function of the glycoprotein Otogelin. Professor Whitfield will present imaging of cilia in the zebrafish ear and discuss the search for the elusive otolith precursor-binding factor, proposed to be located at the kinociliary tip. ![]() Professor Tanya Whitfield, The University of Sheffield, UK
![]() Professor Tanya Whitfield, The University of Sheffield, UKTanya Whitfield is Professor of Developmental Biology at the University of Sheffield. She studied for a PhD with Chris Wylie at the University of Cambridge, followed by postdoctoral work with Julian Lewis at the ICRF in Oxford and London, and EMBO short-term fellowships with Christiane Nüsslein-Volhard in Tübingen, Germany. Her lab in Sheffield studies the developing vertebrate inner ear, using the zebrafish as a model system. The ear is a fascinating system for study, due to its complex three-dimensional arrangement of interlinked ducts and chambers, and multitude of different cell types, including neurons, sensory hair cells, supporting and secretory cells. The entire organ develops from otic vesicle, an epithelium bearing both motile and immotile cilia. |
|
---|---|---|
14:20 - 14:25 | Discussion | |
14:25 - 14:45 |
Neuronal coordination of locomotor cilia in Platynereis larvae
In ciliary swimmers, ciliary beating, arrests, and changes in beat frequency are often coordinated across extended or discontinuous surfaces. To understand how such coordination is achieved, the group studied the ciliated larvae of Platynereis dumerilii, a marine annelid. Platynereis larvae have segmental multiciliated cells that regularly display spontaneous coordinated ciliary arrests. With whole-body connectomics, activity imaging, transgenesis, and neuron ablation the group characterised the entire ciliomotor circuitry of Platynereis. The circuit consists of cholinergic, serotonergic, and catecholaminergic ciliomotor neurons. The synchronous rhythmic activation of cholinergic cells drives the coordinated arrests of all cilia. The serotonergic cells are active when cilia are beating. Serotonin inhibits the cholinergic rhythm, and increases ciliary beat frequency. Based on their connectivity and alternating activity, the catecholaminergic cells may generate the rhythm. The ciliomotor circuitry thus constitutes a stop-and-go pacemaker system for the whole-body coordination of ciliary locomotion. The cholinergic neurons can also be activated upon hydrodynamic stimulation. This response is part of the startle reaction that contributes to predator avoidance. ![]() Dr Gáspár Jékely, Living Systems Institute, University of Exeter, UK
![]() Dr Gáspár Jékely, Living Systems Institute, University of Exeter, UKGáspár Jékely studied Biology and obtained his PhD in 1999 at the Eötvös Loránd Universities in Budapest. He then worked as a postdoc at the EMBL, Heidelberg in the laboratory of Pernille Rorth and then Detlev Arendt. Between 2007-2017 he was a group leader at the Max Planck Institute for Developmental Biology in Tübingen, Germany. He moved to the Living Systems Institute at the University of Exeter as Professor of Neuroscience in 2017. His research interests include the structure, function and evolution of neural circuits in marine ciliated larvae and the origin and early evolution of nervous systems. |
|
14:45 - 14:50 | Discussion | |
14:50 - 15:10 |
Uncovering the role of ciliary beating in the nervous system
Coordinated beating of motile cilia leads to a directional fluid flow, which is important for various biological processes from respiration to reproduction. In the nervous system, motile ciliary beating of ependymal cells allows the cerebrospinal fluid (CSF) to flow through the ventricular system. Such flow plays a central role in the nervous system as human patients or animal models with ciliary defects develop neurological features including hydrocephalus and spine curvature. Still, very little is known about how the nervous system generates and regulates specific flow patterns and how flow controls neural activity and animal behaviour. Here, Dr Jurisch-Yaksi will first describe the mechanisms used by motile cilia to generate specific flow pattern in the zebrafish olfactory epithelium and the function of the flow in olfactory processing. Later, she will discuss how motile cilia and other physiological factors act jointly to regulate cerebrospinal fluid flow dynamics in the brain ventricles and their importance for brain development. Altogether, the group's long-term goal is to understand the role of this increasingly popular organelle in the brain, which has recently attracted much attention from diverse disciplines of neuroscience. ![]() Dr Nathalie Jurisch-Yaksi, Norwegian University of Science and Technology, Norway
![]() Dr Nathalie Jurisch-Yaksi, Norwegian University of Science and Technology, NorwayNathalie Jurisch-Yaksi was trained as a molecular biologist at the University of Lausanne in Switzerland. For her PhD, she pursued research on cancer biology and transcription regulation at the German Cancer Research Center. In 2009, she moved to Massachusetts General Hospital in Boston, where she used zebrafish and the tools of chemical biology to identify novel mechanisms regulating the development of germ cell progenitors. Since 2010, Dr Jurisch-Yaksi is studying the role of the cilium in the development and function of the nervous system, first during her postdoc at VIB in Belgium and now as a senior researcher and team leader associated with the Yaksi lab at the Kavli Institute for Systems Neuroscience in Norway. |
|
15:10 - 15:15 | Discussion | |
15:15 - 15:50 | Coffee | |
15:50 - 16:00 | Selected short presentation | |
16:00 - 16:20 |
Cilia driven flows in the brain ventricles
The ventricular system consists of four interconnected cavities whose inner surface is covered by an array of motile cilia that transport cerebral spinal fluid (CSF) in a complex pattern through the ventricles. CSF is the 'blood of the brain' and is produced by the choroid plexii, secretory epithelia that reach deep into the ventricles. CSF harbours myriads of dissolved and vesicle-enclosed bioactive components (here referred to as 'cargo') that have diverse physiological functions in the brain. Professor Eichele shows how vesicular cargo (liposomes and exosomes) rapidly passes through the ventricles following precise trajectories. Transported cargo is capable of interacting with ciliary membranes and ependymal and subependymal tissues. The group characterises the structural and genetic basis of the cilia-driven flow. Mutations in regulatory proteins controlling physiological timing evoke specific changes in the flow pattern and hence in the transport of cargo. To elucidate the function of vesicular cargo on brain tissue, the group develop cell-based in vitro assays in which exosomes are tested for their ability to control the structure and function of neuronal networks. Importantly these networks are formed from neurons that arise from neuronal stem cells located in the proximity of the ventricles. The group's multipronged approach suggests a sequential step model in which cargo in the form of exosomes is first secreted by the epithelium of the choroid plexi. Subsequently, cargo is directionally transported by cilia-driven flows to selected regions. In such regions, exosomes locally interact with the ependyma and are prepared for passage towards targets areas deeper in the brain such as subependymal tissues and brain parenchyma. ![]() Professor Gregor Eichele, Max Planck Institute for Biophysical Chemistry, Germany
![]() Professor Gregor Eichele, Max Planck Institute for Biophysical Chemistry, GermanyGregor Eichele studied chemistry and structural biology and received his doctorate in 1980. Subsequently, he did a postdoctoral fellowship in developmental biology with Bruce Alberts at UCSF. Between 1985 and 1998, he was a faculty member at the Harvard University School of Medicine in Boston and at the Baylor College of Medicine in Houston. In 1997, he became a scientific member of the Max Planck Society and first took the position as a director at the Max Planck Institute for Experimental Endocrinology and subsequently at the Max Planck Institute for Biophysical Chemistry (Goettingen, Germany) where he is currently the director of the department 'Genes and Behavior'. For his research he has received numerous awards, among them the Friedrich Miescher Prize, the McKnight Neuroscience Development Award as well as the Innovation Award in Functional Genomics. |
|
16:20 - 17:00 | Discussion |