Complex rheology in biological systems
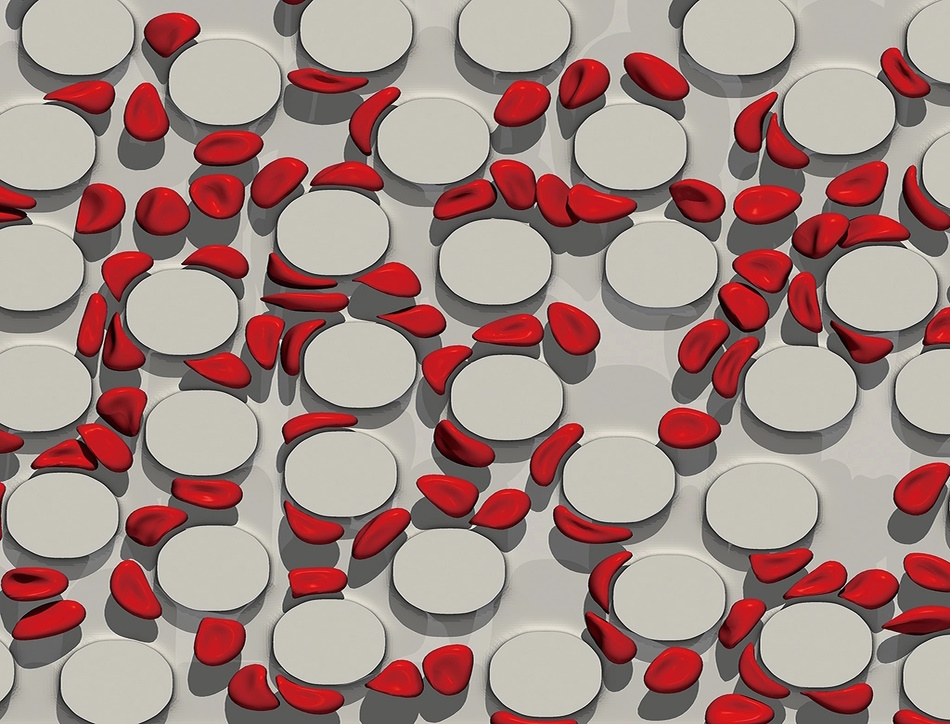
Theo Murphy meeting organised by Dr Charley Schaefer, Professor Lorna Dougan, and Professor Gareth McKinley FRS.
The meeting will bring together an international and interdisciplinary community of researchers working on rheology and rheophysics within biological systems. Examples include viscoelasticity of the intra- and extracellular medium, sinovial fluids, blood, saliva, mucus, silk etc, as well as new experimental, theoretical, and computational methods.
The schedule of talks and speaker biographies are available below. All speaker abstracts will be available closer to the meeting date.
Poster session
There will be a poster session on Monday 16 October. If you would like to apply to present a poster please submit your proposed title, abstract (not more than 200 words and in third person), author list, name of the proposed presenter and institution to the Scientific Programmes team no later than Friday 1 September 2023. Please include the text 'Poster submission' in the email subject line. Please note that places are limited and posters are selected at the scientific organisers' discretion. Decisions will be made by Friday 8 September 2023.
Attending the meeting
This meeting is intended for researchers in relevant fields and is a residential meeting.
This is an inclusive meeting that welcomes academics and industrial researchers at any stage in their career, and with any research interest that is related to the theme 'Complex Rheology in Biological Systems'. We encourage the participation of female researchers and researchers from under-represented groups. PhD students are also welcome to attend. Places for the event are limited, and to maximise interactions between participants, priority will be given to those who submit a poster abstract.
- Free to attend
- Registration is now closed
- This is an in-person meeting
- Catering options will be available to purchase during registration. Participants are responsible for booking their own accommodation
Enquiries: contact the Scientific Programmes team
Schedule
Chair
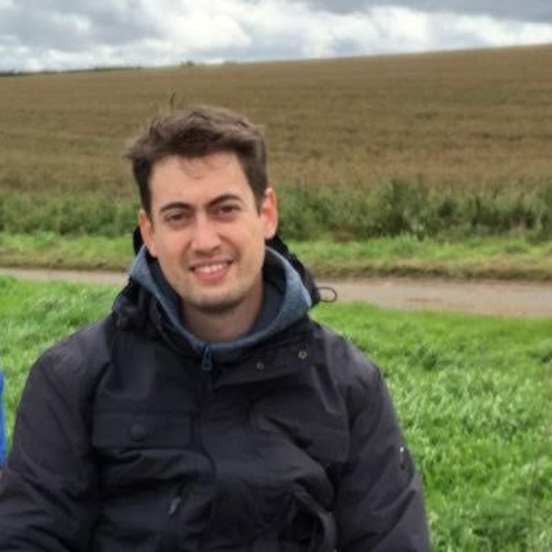
Dr Charley Schaefer, University of York, UK
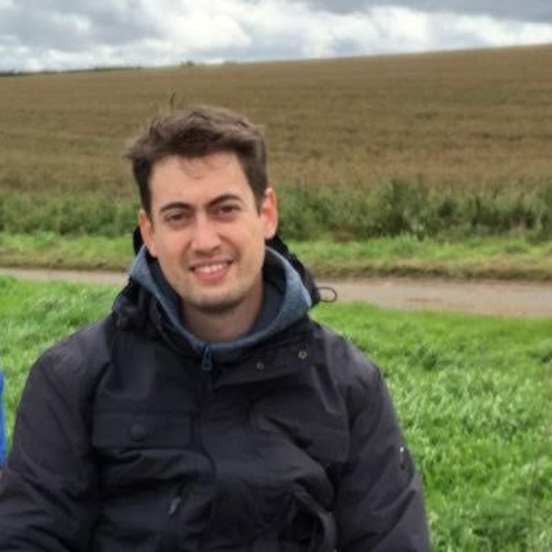
Dr Charley Schaefer, University of York, UK
Dr Schaefer is an Early Career researcher in the Physics of Life group in York who develops theoretical models and methodologies to study the collective dynamics of macromolecules in (biological) soft condensed matter. His interest in rheology is in the context of bio-polymer networks, in particular to understand the dynamical structure of multi-component bio-molecular condensates, and to understand the process by which solutions of silk proteins are deformed by flow and self-assemble into fibres.
10:00-10:25 |
Welcome coffee
|
---|---|
10:25-10:30 |
Introduction
|
10:30-11:00 |
Complex flow in the gastro-intestinal tract
The gastrointestinal (GI) tract actively transports food along different organs to mix it with secretions and break down food particles, which can then be absorbed by the body while waste is eliminated. This involves a variety of biological, chemical, and physical phenomena. Understanding flow phenomena in the GI tract is important for human health, as it can impact drug delivery, the spatiotemporal organisation of the microbiota, and lead to health problems related to GI motility dysfunction. This presentation demonstrates how the gastrointestinal tract handles complex flow by providing two examples: one at the organ scale and the other at the microscopic scale. The first example aims to demonstrate how complex fluid dynamics modelling of video defecography can aid in diagnosing defecation disorders. A two-dimensional patient-specific simulation was developed based on standard X-ray video defecographies to quantify velocity, pressure, and stress fields during defecation for patients with normal and pathological defecatory function. The results showed that normal defecation involved a proximal–distal pressure gradient from both the anorectal junction and the anal canal, with the flow dominated by shear-thinning viscous properties. Impaired defecations were also simulated and compared to normal defecation, leading to a discussion of critical factors that could aid in effective medical management. In the second example, the focus is on how fluid is transported and mixed at small scales using microscopic finger-like structures, the so-called villi. A numerical model was developed to simulate flow and mixing due to the active movement of villi. By using the physiological pattern of contractions, it was shown that villi can either transport fluid in the longitudinal direction of the small intestine and/or induce radial mixing. These phenomena are due to a combination of geometric effects (wave propagation) and inertia at moderate Reynolds number. ![]() Dr Clement de Loubens, Université Grenoble Alpes, France
![]() Dr Clement de Loubens, Université Grenoble Alpes, FranceClément de Loubens is a CNRS researcher based at Laboratoire Rhéologie et Procédés, part of the University of Grenoble Alpes. He has been working at CNRS since 2016 and has extensive experience in the field of complex fluid flows and soft matter physics, particularly those related to the digestive system. Clément has made significant contributions to our understanding of how complex fluids behave in the digestive system. His research involves using a combination of experimental and numerical approaches to investigate the flow and mixing of non-Newtonian fluids in the human digestive tract, both at the organ scale and at the microstructure level of the intestinal mucosa. Through his research, Clément de Loubens has shed light on the role of active microstructure, the so-called villi, in mixing and the importance of non-Newtonian properties of human feces in defecation problems. |
11:00-11:15 |
Discussion
|
11:15-11:45 |
Microrheology of mucus measured on lung tissue culture and on collected samples
Mucus is a viscoelastic fluid that participates to the protective barrier of many mammals' epithelia. In the airways, together with cilia beating, mucus rheological properties are crucial for lung mucociliary function, and, when impaired, potentially participate to the onset and progression of chronic pulmonary diseases. Human bronchial epithelium (HBE) cultures are highly reliable models to assess non contaminated mucus. Hallmarks of shear-thinning and elasticity are obtained at the macroscale whereas at the micro-scale, HBE mucus appears as a heterogeneous medium showing an almost Newtonian behaviour in some extended regions and an elastic behaviour and adhesion forces close to boundaries. Finally, Dr Massiera reports an original method to measure mucus microrheology directly on the tissue culture using optical tweezers, showing that mucus gradually varies in rheological response, from an elastic behaviour close to the epithelium to a viscous one far away. Taken together, all these results collectively support a structure composed of a network of elastic adhesive filaments with a large mesh-size, embedded in a very soft gel. ![]() Dr Gladys Massiera, Université de Montpellier, France
![]() Dr Gladys Massiera, Université de Montpellier, FranceGladys Massiera is a professor of the University of Montpellier, in Laboratoire Charles Coulomb. She completed a PhD in Physics in 2002 in the field of soft condensed matter and a post-doctoral research (2002-04) at the University of Pennsylvania, on cell mechanics. In 2004, she became assistant professor from the University of Grenoble and developed new research activities using biomimicry to understand cellular flow and adhesion. After joining the University of Montpellier in 2006, she patented a now widely used microencapsulation process. She also studied red blood cells, deciphering some aspects of parasites release and interactions with flow in the specific case of sickle cell anemia genetic disease. She became a professor in 2017. Over the last 8 years, her research has been focused on the physical aspects of the muco-ciliary function; mucus rheology and synchrony in cilia beating for mucus flow in collaboration with physicians and biologists from Montpellier. |
11:45-12:00 |
Discussion
|
12:00-12:30 |
Bacterial colonies as active fluids
Bacterial spreading via motility and growth plays a central role in agriculture, biotechnology, the environment, and medicine. These processes are typically studied in the lab in liquid cultures or on flat surfaces. However, many bacterial habitats, eg soils, sediments, and biological gels/tissues, are more complex and crowded 3D spaces. In this talk, Professor Datta will describe his group's work using tools and approaches from soft matter and rheology, imaging, and biophysical modelling to unravel how life in a complex 3D space influences how bacteria spread. He has developed the ability to (i) directly visualise bacteria from the scale of a single cell to that of an entire population, and (ii) 3D-print precisely structured multi-cellular communities, in crowded 3D media more akin to their natural habitats. His experiments using this platform have revealed previously unknown ways in which living in a complex space fundamentally alters how bacteria move and grow, both at the single cell and population scales. Moreover, our results indicate that bacterial colonies can be modelled as 'active fluids' whose rheology is governed by the coupling between cellular motility, growth, and external chemical signals. This provides new ways to predict and control the organisation of bacteria, and other forms of 'active matter', in complex environments more accurately. These findings could also potentially help provide quantitative guidelines for the control of these dynamics in processes ranging from bioremediation and agriculture to drug delivery. ![]() Professor Sujit Datta, Princeton University, USA
![]() Professor Sujit Datta, Princeton University, USASujit Datta is an Associate Professor and Director of Graduate Studies of Chemical and Biological Engineering at Princeton University. He earned a BA in Mathematics and Physics and an MS in Physics in 2008 from the University of Pennsylvania, and then a PhD in Physics in 2013 from Harvard, where he studied fluid dynamics and instabilities in soft and disordered media with Dave Weitz. His postdoctoral training was in Chemical Engineering at Caltech, where he studied the biophysics of the gut with Rustem Ismagilov. Datta joined Princeton in 2017, where his lab studies the dynamics, self-organisation, and applications of complex, soft ('squishy'), and living systems. Datta’s research has revealed and shed new light on the fascinating behaviours manifested by complex fluids and bacterial populations in complex environments, guiding the development of new approaches to environmental remediation, energy production, agriculture, water security, and biotechnology. He also actively leads outreach efforts in STEM to bring together diverse perspectives and provide access to researchers from traditionally under-represented groups in studies of soft and living systems. Datta’s scholarship has been recognized by awards from a broad range of different communities, reflecting its multidisciplinary nature, including through the AIChE 35 Under 35 Award, ACS Unilever Award, Camille Dreyfus Teacher-Scholar Award, three awards from the APS (Early Career Award in Biological Physics, Andreas Acrivos Award in Fluid Dynamics, and the Apker Award), the Arthur Metzner of the Society of Rheology, Pew Biomedical Scholar Award, NSF CAREER Award, and multiple commendations for teaching. |
12:30-12:45 |
Discussion
|
Chair
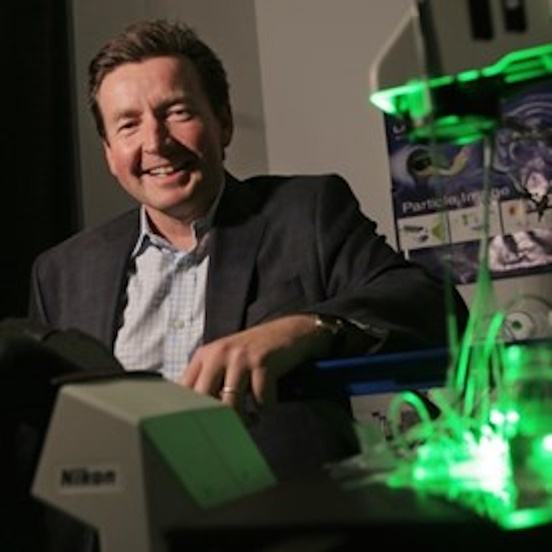
Professor Gareth McKinley FRS, MIT, USA
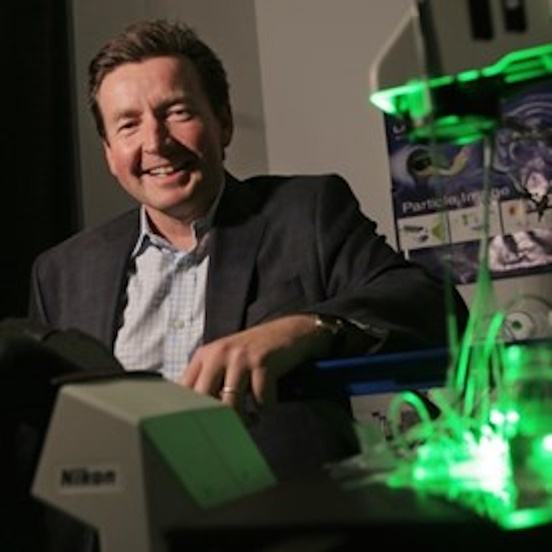
Professor Gareth McKinley FRS, MIT, USA
Gareth H McKinley was born in Hertford, England and studied at Downing College, Cambridge before moving to MIT for his PhD. His work focuses on understanding the rheology (ie the flow properties) of complex fluids such as surfactants, gels and polymers, which are ubiquitous in foods and consumer products. His research interests include non-Newtonian fluid dynamics, microfluidics, extensional rheology, field-responsive materials, super-hydrophobicity and the wetting of nanostructured surfaces.
13:45-14:15 |
Mechanical feedback in regulating the size of growing multicellular spheroids
How do cells sense and respond to the mechanical stress generated by their own growth? How do they achieve a finite and stable size of the organ they form? These are fundamental questions in biorheology that have implications for development, homeostasis and disease. In this talk, Dr Erlich will present a novel continuum model that incorporates an energetic cost of growth into the morphoelastic framework. This model allows for a local feedback mechanism between growth and stress that leads to size control of growing tissues. This feedback mechanism is called a continuum 'growth law', a rheological law that models growth analogously to plastic flow. He will apply this model to multicellular spheroids, which are spherical aggregates of cells that mimic some aspects of solid tumours. Dr Erlich will show how the model can reproduce the experimentally observed features of spheroids, such as residual stress, necrotic core and growth arrest. He will also discuss how the model can be used to explore the effects of different parameters and boundary conditions on the spheroid dynamics. This work demonstrates the importance and usefulness of rheological methods and models for understanding complex biological systems. ![]() Dr Alexander Erlich, Université Grenoble Alpes, France
![]() Dr Alexander Erlich, Université Grenoble Alpes, FranceAlexander Erlich is an applied mathematician who investigates how mechanics shapes and regulates living biological systems. He is currently a CNRS researcher at the Laboratoire Interdisciplinaire de Physique in Grenoble, France, where he focuses on modelling growth in living elastic tissues. He is especially interested in how feedback laws between growth, mechanical stress, and chemical fields determine the final size of an organism. Alexander obtained his PhD in non-linear elasticity and growth from the University of Oxford, where he worked with Alain Goriely and Derek E Moulton. He then did post-docs in fluid mechanics and transport at the University of Manchester, cell motility at LiPhy (Grenoble), and developmental biology at CENTURI (Marseille). His publications include topics such as growth-induced instabilities, placental transport, tissue folding, tumour spheroids, water lilies and Ammonites’ shells. He also collaborates with experimental biologists and physicists to test and validate his models. |
---|---|
14:15-14:30 |
Discussion
|
14:30-15:00 |
The role of the self-generated rheology and mechanical properties of biofilms
Bacterial biofilms generate their own internal stresses by growth and production of exopolysaccharides (EPS). A central question seems to be how these bacterial colonies reconcile the mechanical strength to protect themselves from the access to nutrients. Professor Vermant focuses on colonies which generate a significant amount of EPS. A first challenge he will address is to measure the rheological properties in situ, either macroscopically or using active microrheological measurement. Second, Professor Vermant will discuss the relevance of the rheological properties and their time evolution in the development and transport phenomena within the biofilm. ![]() Professor Jan Vermant, ETH Zürich, Switzerland
![]() Professor Jan Vermant, ETH Zürich, SwitzerlandDr Vermant studied Chemical Engineering at KU Leuven in Belgium, obtaining the doctoral degree in 1996 under the supervision of Professor Jan Mewis. He was a postdoctoral fellow of Elf Aquitaine (Stanford) and the Fund for Scientific Research – Vlaanderen. In 2000 he joined the faculty at the department of Chemical Engineering at KU Leuven, becoming a full professor in 2005. In 2014 he joined the Materials Department at the ETH Zürich where he now heads the laboratory of Soft Materials. He has held visiting appointments at Stanford University (USA), University of Delaware (USA), Princeton University (USA), the Forschungszentrum Jülich (G) and the ESPCI (F). His research at the ETH Zürich focuses on the rheology and applications of complex fluid-fluid interfaces, colloidal suspensions and the development of novel experimental methods and soft matter applications in materials science. |
15:00-15:15 |
Discussion
|
15:15-15:45 |
Break
|
15:45-16:15 |
Flow-driven biofilm assembly and rheology in porous systems
Biofilms are aggregates of microorganisms in which cells are embedded in a self-secreted matrix of polymeric substances, which protects the microbial community from chemical and mechanical insults, thus favouring its survival and evolutionary success. As a result, biofilms have a crucial impact in environmental, industrial, and medical settings. Despite this, there is a severe lack of understanding of how the environment shapes biofilms’ physico-chemical properties and, in turn, how their characteristics drive their response to environmental conditions. Dr Secchi investigates how environmental conditions drive biofilm assembly and the emergence of distinctive morphological and rheological properties. In particular, she focuses on fluid flow, given its ubiquities in the aquatic habitats where biofilms are found. Flow can influence several stages of biofilm formation, starting from surface colonisation. On curved surfaces, flow can promote the formation of colonisation hotspots, which lately impact biofilm formation. Surface geometry, flow shear, fluid rheology, and bacterial phenotype are the parameters controlling surface colonisation. She will also show that during biofilm maturation in porous systems, the interplay between biological functions, ie growth, and physics mechanisms, ie flow shear stress, controls biofilm morphology, rheology, and ultimately affects the physiological protective function of biofilms. By shedding light on this interplay, we can control biofilm development, showing the prominent role that physics can play in developing novel antifouling strategies. ![]() Dr Eleanora Secchi, ETH Zurich, Switzerland
![]() Dr Eleanora Secchi, ETH Zurich, SwitzerlandDr Eleonora Secchi is the Group Leader of the bioMatter Microfluidics Group at ETH Zurich, Switzerland. After earning her PhD from the Polytechnic University of Milan, she was a postdoctoral fellow at Ecole Normale Supérieure of Paris, working on measuring water flow from a single carbon nanotube. She was awarded an ETH Postdoctoral Fellowship in 2016. In 2018, thanks to a Swiss National Science Foundation PRIMA grant, Dr Secchi started her research group. Her research aims to understand the physical mechanisms and environmental factors controlling bacterial surface colonisation and biofilm formation. Biofilms are a widespread mode of growth of bacteria and have a crucial impact in environmental, industrial, and medical settings. She brings to this critical problem an interdisciplinary, cutting-edge toolbox: by applying tools from microfluidics, fluid dynamics, microbial ecology, and soft matter physics to characterise biofilm formation and rheology, she seeks to understand their behaviour and impact in natural and industrial systems. |
16:15-16:30 |
Discussion
|
16:30-17:00 |
Quantification of mechanical cell properties with a narrow gap rheometer
Cytoskeleton plays a critical role in the cell shape, locomotion, growth, and division in response to the surrounding environment. Since it may be affected by diseases and drugs, quantification of the rheological cell properties can help understanding their impact. In this study, the breast cancer cell line MCF-7 was treated with different concentrations of microtubule-active drugs. The cell vitality over 90% was possible to obtain in the concentration range far beyond the cytotoxicity level by treating the cells with low concentration of hydroxyurea prior to the drugs treatment. The stiffness of the drug treated cells in a monolayer was measured by a modified narrow-gap rheometer. With a precision for its gap error better than ±0.4μm, it allows detecting in oscillation the viscoelastic response of cells fixed to both rheometer plates at gap widths of micrometer scale down to the linear viscoelastic regime. With known cell coverage, the average cell moduli can be determined from single experimental runs. Studying the impact of bubbles and dust shows that these error sources have no significant impact on our data. Ms Lee found that the gap width has a strong impact on normal force and storage and loss moduli. She observed two regimes depending on the gap width, apparently caused by the cells’ nuclei and cytoskeleton. Yet, no clear impact of the microtubule-active drugs was observed in the linear viscoelastic range. ![]() Ms Suhyang Lee, LSTME Busan Branch, South Korea, and Friedrich-Alexander Universität Erlangen-Nürnberg, Germany
![]() Ms Suhyang Lee, LSTME Busan Branch, South Korea, and Friedrich-Alexander Universität Erlangen-Nürnberg, GermanyMs Suhyang Lee holds a Master of Science in chemical and bio engineering from Friedrich-Alexander Universität (FAU) Erlangen-Nürnberg, Germany. Her Masters' thesis focused on the topic of Light Distribution in Photo-bioreactor, where she simulated the light propagation with the application of geometrical optics. She is currently in a doctoral course at the Institute of Fluid Mechanics at FAU. With the interest of biomatter, she is studying cell rheology under the supervision of Professor Andreas Wierschem. Additionally, she is a full-time researcher at the biorheology group at LSTME-Busan Branch, a German engineering research and development centre in Busan, South Korea. |
17:00-17:15 |
Discussion
|
17:15-17:45 |
Poster flash talk
|
17:45-18:30 |
Poster session
|
Chair
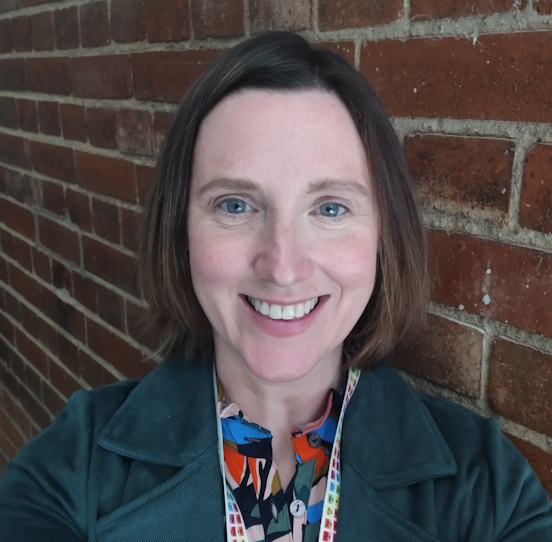
Professor Lorna Dougan, University of Leeds, UK
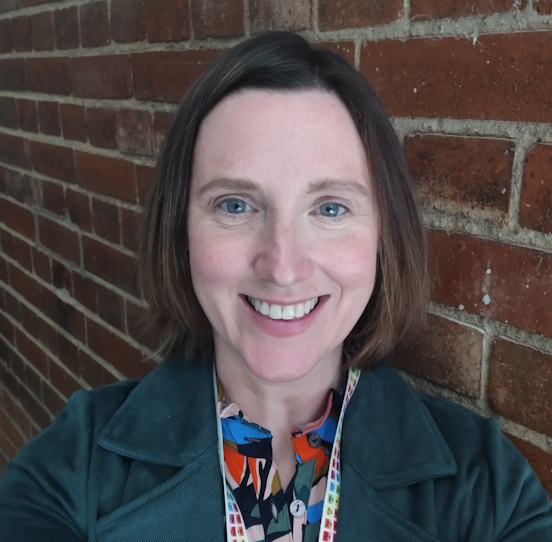
Professor Lorna Dougan, University of Leeds, UK
Lorna is a Professor of Physics and leads a multidisciplinary research group in soft matter and biological physics. She is developing experimental and modelling tools to explore multiscale structure and mechanics across length scales to understand the physics of living systems. She leads an EPSRC Fellowship to exploit engineered polyproteins in the modular design of functional biomaterials and an ERC Consolidator Fellowship (MESONET) to exploit protein unfolding to control network formation in functional protein hydrogels. A major goal of her group is to understand how the structural and mechanical response of a single protein translates to the collective response of a cross-linked network of proteins. To achieve this goal, her group are developing rheological, single molecule, scattering and modelling techniques to capture biomaterial network formation and exploit their properties in applications.
09:00-09:30 |
Animals as material engineers
Animals are under constant pressure to survive in their surrounding environment, and they have evolved countless strategies to adapt, colonise, and reproduce successfully in their habitats. Almost acting as materials scientists, animals directly manipulate complex fluids around them or secrete complex fluids themselves to fulfil a specific task. Here Professor Fischer demonstrates how animals learned to manipulate their habitat, eg sandfish and crabs that exploit the granular rheology of sand (exogen abiotic material) and showcase exotic animals that produce unique bio-fluids (endogen biotic materials), from deep-sea hagfishes to subterranean velvet worms. For unknown and uncharted phenomena, potential rheological properties of the involved complex fluids are suggested. During evolution, animals found ways to use the rheology and structure of complex fluids to gain advantage and increase their Darwinian fitness. Studying the design concepts from soft-matter, materials-science, and rheological points of view can help to understand animal behaviour, might yield new insights for mimicking biomaterials, and provide a quantitative approach toward ethology. ![]() Professor Peter Fischer, ETH Zurich, Switzerland
![]() Professor Peter Fischer, ETH Zurich, SwitzerlandPeter Fischer studied physics and received his PhD in 1995. Followed by a Postdoc position at Stanford University he moved in 1998 to ETH Zurich. Research activities focus on soft matter and food material sciences, in particular on interfaces and emulsions, viscoelastic surfactant solution, and biopolymers. Experimental techniques include rheology, interfacial rheology, microfluidics, neutron and x-ray scattering and reflectivity. |
---|---|
09:30-09:45 |
Discussion
|
09:45-10:15 |
Semen rheology and its relation to male infertility
Infertility affects 15% of couples of reproductive age worldwide. In spite of many advances in understanding and treating male infertility, there is still a number of issues that need further investigation and translation to the clinic. Although it is well recognised that altered rheological properties of semen can impair normal sperm movement in the female reproductive tract, routine semen analysis is mostly focused on number, motility and morphology of spermatozoa, and includes only an approximate, operator-dependent measure of semen viscosity. Here, Dr Tomaiuolo provides the first quantitative experimental evidence based on rheological measurements that post-liquafaction normospermic human semen behaves as a viscoelastic fluid and the shear moduli can be scaled according to the weak-gel model. ![]() Dr Giovanna Tomaiuolo, University of Naples Federico II, Italy
![]() Dr Giovanna Tomaiuolo, University of Naples Federico II, ItalyGiovanna Tomaiuolo graduated in Chemical Engineering in 2005 and received her PhD in 2009 in Industrial Biotechnological Sciences at the University of Napoli Federico II on the fluid dynamic behaviour of red blood cells under confined shear flow. During her doctoral studies she has worked at the University of Texas, Health Science Center and MD Anderson Cancer Center, Houston (TX) on modelling of breast cancer, and at the Physics of Complex Fluids Laboratory, Dept. Science and Technology, and MESA, University of Twente on soft-lithography techniques for microfluidics systems. She is currently Associate Professor at the Department of Chemical, Materials and Production Engineering at the University of Napoli Federico II. She is also affiliated at the CEINGE Advanced Biotechnologies Center. Her research activity is mainly devoted to the analysis of transport phenomena of complex fluids in microfluidic systems. In particular, the research activity shows a strong interdisciplinary nature, being built around complex biological fluids, such as blood, mucus and semen, multiphase flows, microfluidics, flow-chemistry and rheology. |
10:15-10:30 |
Discussion
|
10:30-11:00 |
Break
|
11:00-11:30 |
E-coli are still full of surprises: example of motion in liquid crystals
In many situations, microswimmers like bacteria, move in complex environments associated with non-Newtonian rheology. We can model these fluids using liquid crystals, where the kinetics of bacterial motion is constrained by the orientational molecular order of the fluid, leading to new dynamics. In this presentation, we will study the swimming reorientation of a single bacterium, E coli, first extremely slowed down in a solution of Ficoll400 (viscous Newtonian fluid), and secondly in the liquid crystal (anisotropic, viscous, elastic, non-Newtonian) confined to a planar cell. In such an environment, the spontaneous run and tumble motion of the bacterium gets frustrated: the elasticity of the liquid crystal prevents flagella from unbundling. Interestingly, in order to change direction, bacteria execute a reversal motion along the director field, driven by the relocation of a single flagellum to the other side of the bacterial body, coined as a frustrated tumble. Dr Goral will expose a detailed experimental characterization of this phenomenon, exploiting exceptional spatial and temporal resolution of bacteria and flagella dynamics during swimming, obtained using a two colour Lagrangian tracking technique. She will suggest a possible mechanism behind the frustrated run and tumble motion, accounting for these observations. ![]() Dr Martyna Goral, University of British Columbia, Canada
![]() Dr Martyna Goral, University of British Columbia, CanadaAfter graduating in fluid mechanics from Sorbonne University and Ecole Polytechnique, Martyna Goral did her PhD on low Reynolds number locomotion in a non-Newtonian fluid, which mixed fields of soft and active matter. Her experimental observation of E-coli bacteria in liquid crystals highlighted the variety of bacterial behaviour and how it can drastically be affected by its environment. This work was supervised by Anke Lindner and Teresa Lopez-Leon at ESPCI in Paris. She continued her academic path as a post-doctoral fellow in a more applicative domain of microfluidic pressure sensor; a novel technology based on a colour signal. Currently she is working with Ian Frigaard in Vancouver on rising bubbles through yield-stress fluids, an experimental modelling of gas emission of tailing ponds. |
11:30-11:45 |
Discussion
|
11:45-12:15 |
Microhaemodynamics shapes blood rheology in disordered porous networks
Red blood cells (RBCs), carrying oxygen around the body through different vessels and tissue structures, play a critical role in metabolism of the human body. However, in addition to the transport properties of RBCs, the presence of RBCs affects the rheology of blood flowing within small blood vessels. This non-Newtonian rheology can result in structural alterations such as capillary constriction, causing potentially transient changes to the vessel structure. Mathematical frameworks for blood flow within capillary-based vasculature currently exist, however, for biological tissue characterised by disordered porous structures, but the interplay between the tissue structure and haemodynamics is unclear. To better understand how the rheology of blood depends on factors such as microstructure connectivity and RBC volume fraction we use (i) microfluidic experiments with deflated PDMS capsules, (ii) immersed-boundary lattice-Boltzmann method (IB-LBM) to simulate RBC flow within porous structures, and (iii) a reduced-order network model to interpret experimental and simulation data in terms of biophysical mechanisms. Using IB-LBM in a range of ordered and disordered porous structures Dr Doman and her collaborators (including Dr Qi Zhou from the University of Edinburgh) observes that the relative apparent viscosity of the blood increases with structural disorder of the porous medium. Overall with all three techniques, Dr Doman and her collaborators observe that the presence of RBCs alters local flow distribution. This work contributes to a better understanding of the relationship between blood rheology and biological tissue structure. ![]() Dr Eleanor Doman, University of Manchester, UK
![]() Dr Eleanor Doman, University of Manchester, UKDr Eleanor Doman is a research associate in the Department of Mathematics at the University of Manchester. She gained her PhD in Applied Mathematics at University College London, where she used asymptotic methods to construct a multiscale model of a peripheral nerve. She now works as part of a multidisciplinary team between the University of Manchester and the University of Edinburgh aiming to better understand suspension flow within disordered media to mimic blood flow through biological tissues such as the intervillous space in the human placenta. |
12:15-12:30 |
Discussion
|
13:30-14:00 |
Correlating viscosity and molecular crowding with fluorescent nanobeads and molecular probes: in vitro and in vivo
In eukaryotes, intracellular physicochemical properties like macromolecular crowding and cytoplasmic viscoelasticity influence key processes such as metabolic activities, molecular diffusion, and protein folding. However, mapping crowding and viscoelasticity in living cells remains challenging. One approach uses passive rheology in which diffusion of exogenous fluorescent particles internalised in cells is tracked and physicochemical properties inferred from derived mean square displacement relations. Recently, the crGE2.3 FÓ§rster Resonance Energy Transfer (FRET) biosensor was developed to quantify crowding in cells, though it is unclear how this readout depends on viscoelasticity and the molecular weight of the crowder. Here, Professor Leake presents correlative, multidimensional data to explore diffusion and molecular crowding characteristics of molecular crowding agents using super-resolved fluorescence microscopy and ensemble time-resolved spectroscopy. He firstly characterised in vitro and then apply these insights to live cells of budding yeast Saccharomyces cerevisiae. It is, to his knowledge, the first time this has been attempted. Professor Leake demonstrates that these are usable both in vitro and in the case of endogenously expressed sensors in live cells. Finally, he presents a method to internalise fluorescent beads as in situ viscoelasticity markers in the cytoplasm of live yeast cells, and discuss limitations of this approach including impairment of cellular function. ![]() Professor Mark Leake, University of York, UK
![]() Professor Mark Leake, University of York, UKMark Leake is a physicist by training, addressing challenging biophysical and biochemical questions in a range of biological processes. He gained his biophysics PhD on muscle proteins using optical tweezers in King’s College London, later postdoctoral positions in Oxford and Heidelberg, and independence as a Royal Society URF in Oxford, leading research in single-molecule biophysics, prior to becoming Anniversary Chair of Biological Physics at the University of York in 2013. Mark was Director-Founder of the Biological Physical Sciences Institute and is Coordinator of the Physics of Life Group in the University of York and Chair of the UK Physics of Life network PoLNET. His research involves developing new biophysical instrumentation for addressing open biological questions, and coupling these to molecular biology/biochemistry to investigate single biomolecules under physiologically relevant environments. |
---|---|
14:00-14:15 |
Discussion
|
14:15-14:45 |
Rheology of topologically active DNA
Classic polymer physics successfully describes most of the polymeric materials we see every day. Yet, it relies on the assumption that polymers do not change topology (or architecture) in time or that if they do alter their morphology, they do so in equilibrium. This assumption spectacularly fails for DNA in vivo, which is constantly topologically re-arranged by ATP-consuming proteins within the cell. Inspired by this, Dr Michieletto's group studies entangled systems of DNA functionalised by certain proteins which can selectively alter DNA’s topology and architecture in time and may expend energy to do so. Solutions of 'topologically active' DNA are a new class of polymeric systems that display unconventional out-of-equilibrium viscoelastic behaviours and can be conveniently realised in vitro. ![]() Dr Davide Michieletto, University of Edinburgh, UK
![]() Dr Davide Michieletto, University of Edinburgh, UKDavide received his PhD from the University of Warwick in 2015. His PhD thesis on 'Topological Interactions in Ring Polymers' was awarded the Faculty of Science PhD Thesis Prize from the University of Warwick, the Outstanding PhD Thesis Prize from Springer Publishing Group and the Ian Macmillan Ward Prize from the Institute of Physics. During his PhD he coined a new shape of pasta, now known as 'anelloni' (from the Italian word 'anello' for ring). Davide then moved to Edinburgh, where he worked as PDRA with Professor Marenduzzo and Gilbert on computational models of genome organisation and on super-resolution microscopy of chromatin structure (2016-2019). In 2020, Davide was awarded first a Leverhulme Early Career Fellowship and then a Royal Society University Research Fellowship. In the same year he was awarded an ERC Starting Grant to establish his group on 'Topologically Active Polymers'. In 2021, Davide was awarded the Chancellor’s 'Rising star' award from the University of Edinburgh and the Early Career Award in Statistical Mechanics and Thermodynamics from the RSC. |
14:45-15:00 |
Discussion
|
15:00-15:30 |
Break
|
15:30-16:00 |
Modelling the rheology of biological tissue
The deformation and flow properties of biological tissue are important in processes such as embryo development, wound healing and tumour invasion. Indeed, processes such as these spontaneously generate stresses within a living tissue via active process at the single cell level. Tissues are also continually subject to external stresses and deformations from surrounding tissues and organs. The success of numerous physiological functions relies on the ability of cells to withstand stress under some conditions, yet to flow collectively under others. Biological tissue is furthermore inherently viscoelastic, with a slow time-dependent mechanics. Despite this rich phenomenology, the mechanisms that govern the transmission of stress within biological tissue, and its response to bulk deformation, remain poorly understood to date. Simplified vertex models of confluent tissue monolayers have uncovered a spontaneous liquid-solid transition tuned by cell shape. In this talk, Professor Fielding shall review some recent progress in modelling the rheology of biological tissue. First, she will discuss work predicting a strain-induced stiffening transition in a sheared tissue. Second, Professor Fielding shall discuss how the interplay of external deformations applied to a tissue as a whole with internal active stresses that arise locally at the cellular level, is predicted to lead to a host of fascinating rheological phenomena such as yielding, shear thinning, and continuous or discontinuous shear thickening. Third, she will explore the formulation of a continuum constitutive model that captures several of rich linear and nonlinear rheological phenomena noted above. ![]() Professor Suzanne Fielding, Durham University, UK
![]() Professor Suzanne Fielding, Durham University, UKSuzanne Fielding obtained her first degree in physics from Oxford University in 1997 and her PhD from University of Edinburgh in 2000. Following postdoctoral research at the University of Leeds, she spent four years lecturing in Applied Mathematics at the University of Manchester, before moving to the Department of Physics at Durham University in 2009. In 2010 she received the Arthur B Metzner award of the Society of Rheology. |
16:00-16:15 |
Discussion
|
16:15-17:00 |
Panel discussion
|