Chance and purpose in the evolution of biospheres
Also in “ Scientific meeting”
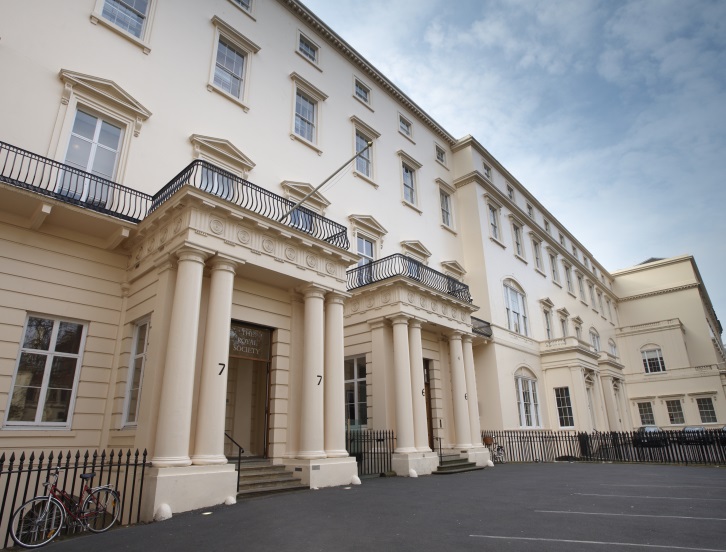
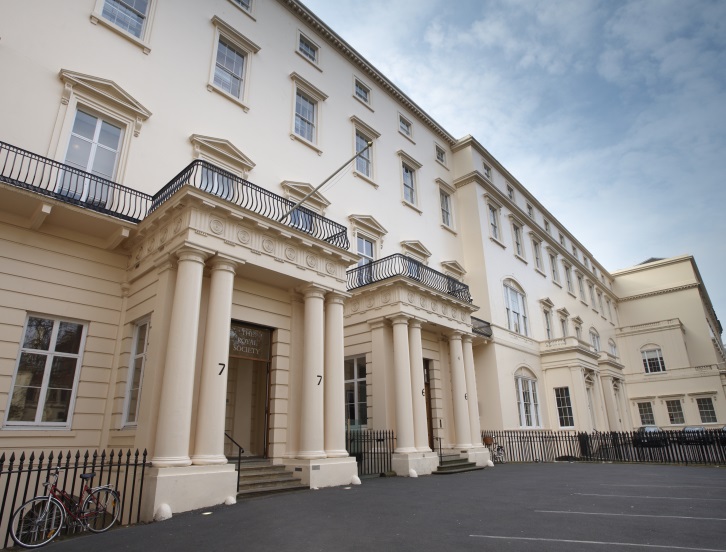
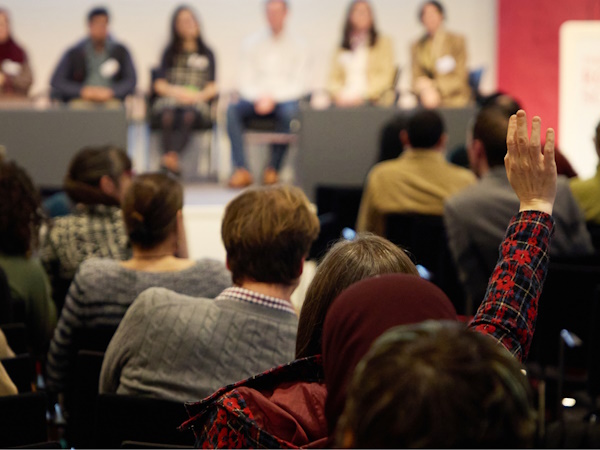
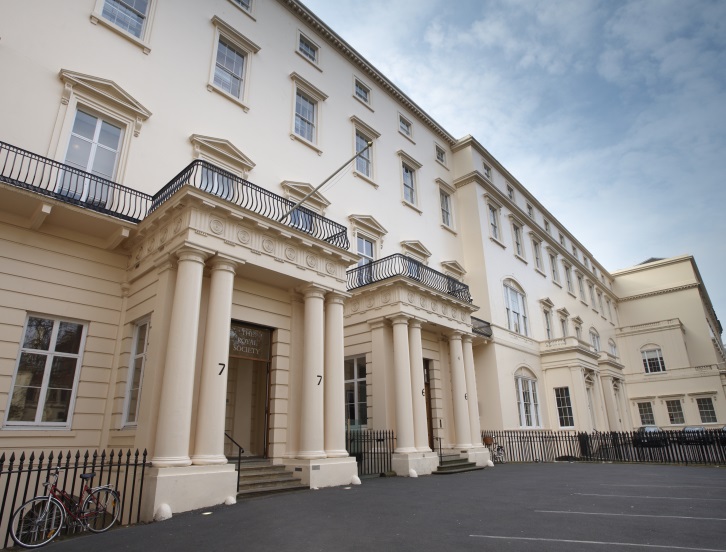
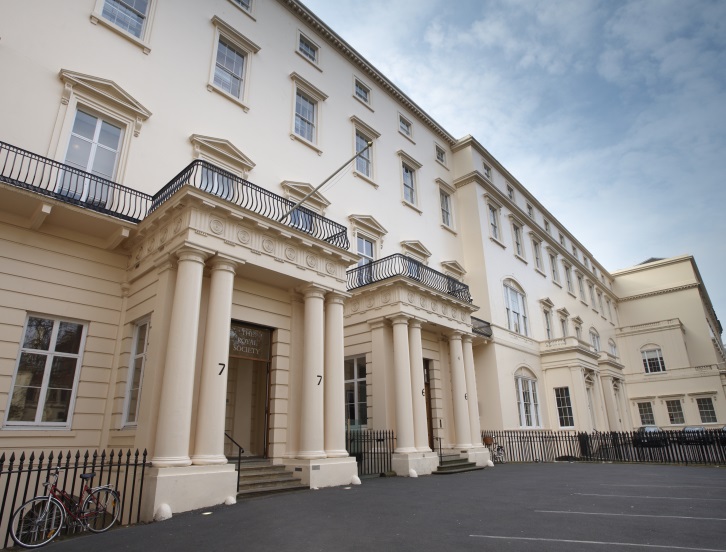
Discussion meeting organised by Professor Philip Donoghue, Professor Anja Spang, Professor Tim Lenton, Professor Samir Okasha, and Professor Graham Shields.
The meeting brought together philosophers and scientists from diverse backgrounds to address how stable biospheres evolve: whether they are a result of chance events or instead the inevitable consequence of the evolutionary interplay between the organisms and their environments. The meeting inevitably considered Earth history, but also exoplanets and simulated exobiospheres.
Organisers
Schedule
Chair
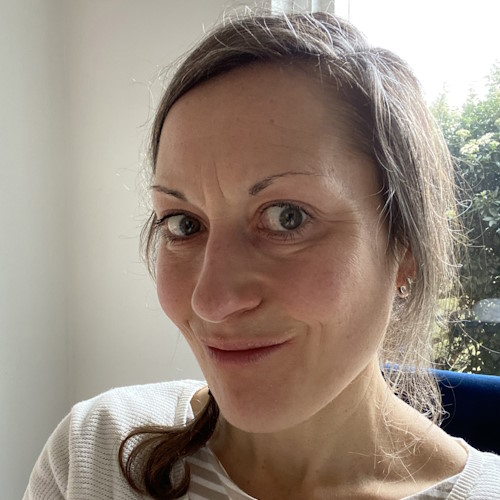
Professor Dr Anja Spang, Netherlands Institute for Sea Research, Netherlands
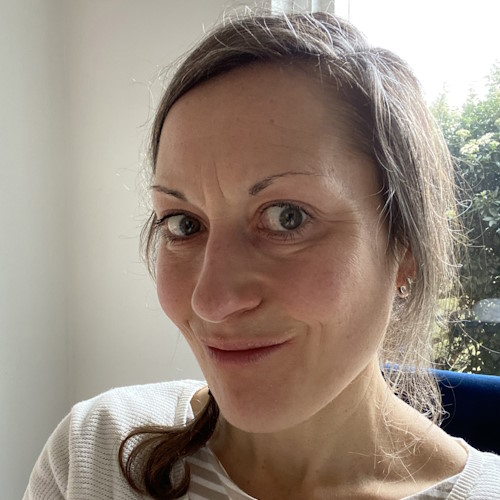
Professor Dr Anja Spang, Netherlands Institute for Sea Research, Netherlands
Professor Dr Spang is an evolutionary microbiologist with a key interest in deep cellular evolution, symbioses and archaea. She defended her PhD in 2013 at the University of Vienna, Austria, and did her post-doctoral studies with Dr Thijs Ettema in Uppsala, Sweden, contributing new insights into the role of Archaea in the evolution of eukaryotes. In 2017, she relocated to the Netherlands for a tenure track position at the Royal Netherlands Institute for Sea Research (NIOZ), where she and her team study the deep roots of microbial life on Earth, the origin and diversification of eukaryotes and the role of recently discovered archaeal symbionts in the evolution, ecology, and diversification of their hosts and ecosystem functioning. Since 2021, she is a tenured research leader at NIOZ and holds a Special Chair Professorship at the University of Amsterdam in Symbioses in Evolution.
09:00-09:05 |
Welcome by the Royal Society and lead organiser
|
---|---|
09:05-09:30 |
The evolution of biospheres
How can biospheres evolve? A biosphere is the clade of all Life coupled to non-living things, including the atmosphere, at a planetary scale. It is not like an organism, because it exchanges very little material with its surroundings, ‘eats’ its own waste products, and does not interact with other biospheres. Models suggest multi-level natural selection going on within a biosphere can produce nutrient recycling at local scales and regulation of heterogeneous environmental variables at ecosystem scales. But global properties such as intense biogeochemical (re)cycling and long-term regulation of atmosphere composition, which promote the flourishing and persistence of Life (on Earth at least), require a different explanation. A plurality of ‘evolutionary’ mechanisms can be at play. Acknowledging the weak Anthropic principle, there are at least two generic mechanisms. Cybernetics has long shown that when innovation within a system produces unstable behaviour (at the system level) this tends to be short-lived, as the system reorganises until it finds a self-stabilising attractor (or in rarer cases, the system goes extinct). This is a robust, though not infallible, mechanism for acquiring persistence-enhancing properties. Persistence can in turn increase the likelihood of acquiring further persistence-enhancing properties - a purely persistence-based form of selection. Models instantiating these mechanisms predict a finite set of generic pathways of biosphere evolution and make testable predictions about how Earth’s biosphere evolved. Thus, through the ongoing search for exobiology and ever deeper study of Earth’s history we are getting close to an empirically grounded theory of biosphere evolution and testing the Gaia hypothesis. ![]() Professor Tim Lenton, University of Exeter, UK
![]() Professor Tim Lenton, University of Exeter, UKProfessor Tim Lenton is the founding Director of the Global Systems Institute at the University of Exeter and Chair in Climate Change and Earth System Science. He has more than 25 years research experience, focused on modelling of the biosphere, climate, biogeochemical cycles, and associated tipping points. Tim is renowned for his work identifying climate tipping points, which informed the setting of the 1.5C climate target, associated net zero targets, and nationally determined contributions. Tim works with policymakers and businesses helping them assess the risks of climate change and nature loss and highlighting the opportunities for "positive tipping points" that can accelerate change towards net zero. In 2023, Professor Lenton led a team of more than 200 people from over 90 organisations in 26 countries to produce an authoritative assessment of the risks and opportunities of both negative and positive tipping points in the Earth system and society. The Global Tipping Points Report produced in partnership with Bezos Earth Fund was published at COP28. |
09:30-09:55 |
Atmospheric composition and climate on the early Earth
Earth’s early atmosphere is thought to have been composed mostly of N2 and CO2, with smaller amounts of reduced gases such as H2 and CO. CO2 is a greenhouse gas which could have helped counter ~25% reduced solar luminosity during the early Archean. Negative feedbacks in the carbonate-silicate cycle that controls atmospheric CO2 levels should have helped keep its concentration high (>0.01 bar). Methane (CH4) should have been present at appreciable levels, ~1000 ppmv, after life had evolved and could have contributed 10-12 degrees to the greenhouse effect. Atmospheric O2 levels would have remained low during the Archean except in the immediate vicinity of ‘oxygen oases’ where cyanobacteria were emitting it. A Great Oxidation Event, or GOE, occurred at roughly 2.2-2.4 Ga, after which O2 levels were much higher, probably of the order of 0.1 times the Present Atmospheric Level (PAL). This is the level at which oxidative weathering of organic carbon on the continents begins to provide strong negative feedback. Because of this relatively high atmospheric O2 level, an effective ozone screen should have been in place throughout most of the Proterozoic. A second rise in O2 near the end of that era paved the way for the evolution of animals during the Phanerozoic. ![]() Professor James Kasting, Pennsylvania State University, USA
![]() Professor James Kasting, Pennsylvania State University, USAJames "Jim" Kasting is a retired Professor of Geosciences from Penn State University, where he taught from 1988 to 2022. Before that, he worked at NASA Ames Research Center in California and at the National Center for Atmospheric Research in Colorado. He is interested in Earth’s early atmosphere and climate, as well as the possibility of life on Earth-like planets around other stars. In conjunction with the latter, he served on the study team for NASA’s proposed Terrestrial Planet Finder—Coronagraph (TPF-C), a direct imaging space telescope that NASA studied during the early 2000’s. Several of his students/ postdocs are now part of the study team for NASA’s Habitable Worlds Observer—a more modern update of TPF-C that could fly in the mid-2040’s. |
09:55-10:20 |
The evolution of the tree of life
The tree of life is one of the most important organising principles in biology. Updates and revisions historically derived from improved data capture and expanded taxon sampling. Tracing the changes in the tree of life over the molecular era (1990-present) highlights the evolution of biologists’ understanding of life on earth, and also serves as a foil placing the explosion of available data over this timeframe in context. Using current-day information, the evolution of the tree of life is explored through historic tree reconstruction. Key “tipping points” for identifying important structural divisions (eg two versus three domains) emerge. Using dissimilarity metrics, the proportion of changes each historic tree encompasses is calculated, identifying an approaching asymptote for revisions to the tree of life at the phylum level. Continued increases in data capture are now facilitating improvements in genome quality rather than diversity, as the rate of novel phylum discovery is slowing, for bacteria and archaea. This work clarifies the minimum data required to construct a stable tree of life and highlights the necessary taxa anchoring this stability. ![]() Associate Professor Laura A Hug, University of Waterloo, UK
![]() Associate Professor Laura A Hug, University of Waterloo, UKDr Hug’s research examines the diversity and function of microbial communities in contaminated sites using a combination of ‘omics approaches and enrichment culturing. Current research in her group is characterising the microbial communities colonising municipal landfills, with foci on methane cycling, bioplastics degradation, and community interactions. Dr Hug’s work has been featured in major news outlets including the New York Times, the Atlantic, Discover Magazine, and on Public Radio International’s The World. She was a featured scientist on a Télévision Français Ontario children’s show and the BBC Radio 4 program, Bacteria, the tiny giants in 2023. |
10:20-10:30 |
Discussion
|
10:30-11:00 |
Break
|
11:00-11:25 |
What the earliest evidence for life tells us about the early evolution of the biosphere
Life emerged on Earth within a specific geological context on what could be considered an “exoplanet”, so different it was from the planet Earth today. Lack of preservation of Hadean rocks prevents us from knowing exactly how, where and when life emerged for which, nevertheless, understanding of the environment of the early Earth, based on inherited geochemical signatures and well-preserved Palaeoarchaean rocks provide some constraints. What is important for the story of life on Earth is that these earliest rocks preserve traces of a flourishing biosphere, albeit anaerobic. The geochemical and morphological remains of prokaryote-like microbes abound in the Palaeoarchaean, predominantly shallow water, volcanic sediments of Barberton and the Pilbara. Already by 3.48 Ga, there is evidence for anoxygenic phototrophy with the formation of microbial mats on sediment surfaces in shallow waters. Geochemical and morphological signatures of chemotrophs occur in subsurface sediments and hydrothermal environments by 3.45 Ga. Other enigmatic structures, such as “spindles” make their appearance at this time. The oldest, well preserved rocks thus document a diversified and flourishing biome, suggesting that life emerged in the Hadean well before 3.5 Ga and was superbly adapted to the “extreme” environmental conditions of the early Earth, conditions that would have been common on early habitable bodies in the Solar System and elsewhere in the Universe. These early cells are similar to modern prokaryotes but not identical. They may not yet have developed cell walls and the various enigmatic structures, including spindles, may be evidence of this. As the oldest preserved evidence of life, these early traces provide the fundament upon which life and the biosphere evolved. ![]() Dr Frances Westall, CNRS-Centre de Biophysique Moléculaire, France
![]() Dr Frances Westall, CNRS-Centre de Biophysique Moléculaire, FranceFrances Westall is a geologist with a broad background in marine geology, sedimentology, geobiology, and prebiotic chemistry, specialising in the environment of the early earth, the earliest traces of life on Earth, the origin of life and the search for life on Mars. After BsC Hons from Edinburgh and a PhD from the University of Cape Town, South African born Westall has worked in many institutions around the world (Alfred Wegener Institute, Bremerhaven, University of Nantes, University of Bologna, NASA Johnson Space Center and the CNRS Centre de Biophysique Moléculaire in Orléans). She holds the Italian Chemistry Society Medal, the Belgian Alfred Dumont Medal, an is Fellow of the Institute for Advanced Studies, Bologna. |
11:25-11:50 |
Early fossil cells evidence major events in biospheric and planetary evolution
LUCA, the most recent ancestor of the three domains of life we know today, lived among other cellular lineages on the Hadean Earth (before 4 Ga). However, these and earlier events since the origin of life can only be inferred using molecular approaches because there is no preserved rock and fossil record to directly evidence the first cells. The body fossil record of cells starts in the Archean, more than 3.2 billion years ago, perhaps earlier, while consensual 3.4 Ga fossil-barren stromatolites build by microbial communities indirectly support the diversification of prokaryotic metabolisms and cells. The Proterozoic fossil record preserves more abundant and diverse cells in marine and continental environments, demonstrating the evolution and diversification of a persistent biosphere modifying its habitable planet, opening new ecological opportunities. These fossil cells directly evidence major events in biospheric and planetary evolution. The geological context preserving organic-walled microfossils inform on their age, ecology and the conditions that preserved, altered or erased their original biological properties (taphonomy). Micro to nano-scale analyses of the morphology, ultrastructure and chemistry of these fossil cells, combined with microbiology and experimental taphonomy of modern microorganisms, as well as information from molecular phylogenies permit to document the evolution of biological innovations and ecological interactions and in some cases, to identify specific microbial clades. This talk will illustrate how early fossil cells may provide unique insights into major evolutionary events such as the evolution of cyanobacteria and Earth oxygenation, eukaryogenesis, and eukaryotic diversification. ![]() Dr Emmanuelle J Javaux, University of Liège, Belgium
![]() Dr Emmanuelle J Javaux, University of Liège, BelgiumEmmanuelle Javaux is a biologist from the University of Liège in Belgium, with a PhD in Earth Sciences from Dalhousie University (Canada, 2000) and postdoc in Knoll’s lab at Harvard. She is a full professor at the University of Liège Geology Department, whose presidency she has assumed for 7 years. She leads the Early Life Traces and Evolution-Astrobiology lab and is director of the Astrobiology Research Unit. She received a Francqui Foundation Research professorship and a European Research Council Starting Grant, was elected member of Royal Academy of Belgium in 2014, member of the Société Philomathique de Paris in 2021, and ISSOL fellow in 2023. She presides the ISSI Scientific Committee and is a member of the EAI Board of trustees, ICDP Board of Governors, and the ExoMars mission. She and her team explore the micro- and nano-scale information preserved in fossil and modern cells to understand how early biospheres evolved on early Earth and possibly beyond. |
11:50-12:15 |
ATP synthase evolution on a cross-braced dated tree of life
The timeline of early cellular evolution is poorly resolved. Dating deep evolutionary events is challenging due to the lack of prokaryotic fossils and the limitations of current molecular clock methods to adequately model rate variation. Relative time constraints and cross-bracing can provide additional temporal information to molecular clock analyses, allowing for the propagation of limited fossil information across the tree and may help improve divergence time estimates otherwise complicated by a patchy and incomplete fossil record. Eukaryotes contain genetic contributions from both sides of the root of the tree of life, that is, from their archaeal and bacterial ancestors. For example, eukaryotes have inherited up to three distinct pools of ribosomes and ATP synthases from their prokaryotic ancestors, with the key catalytic components of the latter being the result of a gene duplication which is estimated to have predated the last universal common ancestor. Such gene duplications (ATP synthase) and endosymbioses (ATP synthase and ribosomal genes) result in phylogenetic node equivalency, where multiple nodes on a gene tree correspond to the same evolutionary event. These corresponding nodes can be cross-braced to the same unknown age in time, providing a tighter constraint on molecular clock estimates. Here, Dr Tara Mahendrarajah will present their recent work applying novel molecular dating approaches to reconstruct a dated tree of life and provide an absolute timeline for ATP synthase evolution, thereby providing new insights into deep cellular evolution. ![]() Dr Tara A MahendrarajahRoyal Netherlands Institute for Sea Research, Netherlands ![]() Dr Tara A MahendrarajahRoyal Netherlands Institute for Sea Research, Netherlands Dr Tara Mahendrarajah is an evolutionary microbiologist whose research examines cellular evolution across the tree of life from the last universal common ancestor to the events leading to eukaryogenesis. Her research interests include understanding the evolutionary processes underpinning key metabolic machines such as the ATP synthase and pathways at transition points across the tree of life. An element of her research includes assessing, expanding, and developing novel methodological approaches for marker gene selection, phylogenetic inference, and molecular dating. Her current work combines phylogenetic, comparative genomic, and gene tree-species tree reconciliation approaches to understand the evolution and lifestyles of ultrasmall DPANN archaea as well as the genetic contributions from prokaryotes to eukaryotes. |
12:15-12:30 |
Discussion
|
Chair
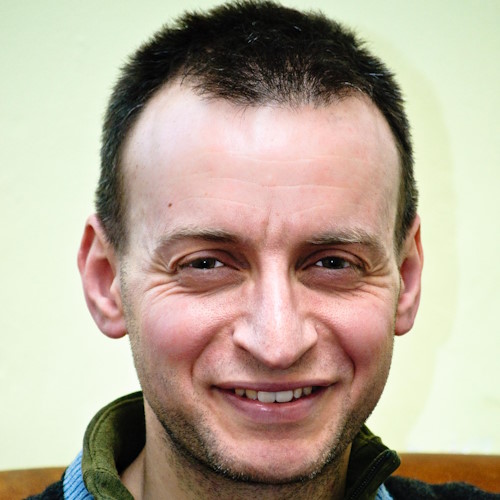
Professor Samir Okasha FBA, University of Bristol, UK
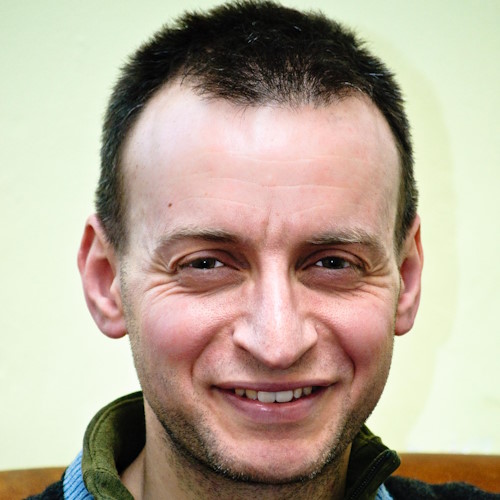
Professor Samir Okasha FBA, University of Bristol, UK
Samir Okasha FBA is Professor of Philosophy at the University of Bristol. He has spent much of his career working on issues in the philosophy of biology, in particular the foundations of evolutionary theory. He also worked on issues in epistemology and metaphysics of science, including causation, induction and probability.
Samir has published widely in both philosophical and scientific journals. His 2006 book Evolution and the Levels of Selection was awarded the Lakatos Prize for an outstanding contribution to the philosophy of science. His latest book, Agents and Goals in Evolution, was published in 2018. He is also the author of Philosophy of Science: a very short introduction, and Philosophy of Biology: a very short introduction, both published by Oxford University Press.
13:30-13:55 |
Hotspots and hot moments in deep time: exploring the role of interfaces in sustaining the early biosphere
Life requires chemical disequilibria, which are commonly encountered at the interfaces of differing water masses. Environmental settings such as coastal upwelling zones or estuaries where fluid mixing occurs therefore harbour some of the most active and diverse biological communities today. This concept has become known as ‘hotspots and hot moments’ in modern ecology, and it is underpinned by the substrate requirements of primary producers and the composition of natural waters. On the early Earth, prior to widespread oxygenation, it is conceivable that relevant environmental interfaces were distributed differently and displayed distinct characteristics. This talk will explore the interfaces around hydrothermal vents and rivers as biological drivers during key intervals in the Precambrian. Case studies reveal that hydrothermal settings in particular were not only the major sources of micronutrients during the Archean but also regenerated ammonium into the open ocean. In contrast, terrestrial and estuarine settings likely supplied less bioavailable nitrogen into marine ecosystems than they do today, but they likely offered niches for biological diversification. Collectively, these observations emphasise the importance of volcanic activity and crustal emergence for creating ‘hotspots and hot moments’ for the sustenance and diversification of early life on Earth. ![]() Dr Eva Stüeken, University of St Andrews, UK
![]() Dr Eva Stüeken, University of St Andrews, UKDr Eva Stüeken is a geobiologist and sedimentary geochemist with expertise in reconstructing environmental conditions and biogeochemical cycles in deep time. She obtained a BSc at Jacobs University Bremen, Germany, a PhD at the University of Washington in Seattle, USA, and worked as a NASA postdoctoral fellow at the University of California Riverside before moving to the University of St Andrews in the UK in 2017. Her interests revolve around studying the origin and early evolution of life on Earth as an analogue for other habitable worlds. Tools include a combination of field work, laboratory analyses, experiments and simple computational models. |
---|---|
13:55-14:20 |
Phylogenomic reconstruction of metabolic evolution
Metabolic pathways are a series of essential biochemical reactions and cycles responsible for sustaining life through: the facilitation of energy production; the synthesis of nucleotides, amino-acids, proteins, and cellular components; and the overall maintenance of the cellular environment, including growth and the removal of waste products. Understanding the evolution and origins of these different fundamental biochemical reactions, as well as how these pathways and cycles have changed over time, is important not only from an evolutionary or biological context but also in terms of the history of the Earth system itself. Understanding the evolution of metabolism helps us explain how we go from a lifeless geochemical planet to the lively Earth we enjoy today. Here, Dr Edmund R R Moody presents the use of a probabilistic approach to reconstructing metabolic cycles using gene-tree species-tree reconciliation in combination with molecular clock divergence time estimation analyses, allowing us to determine where different key gene families involved in metabolic cycles evolved on a species tree and when they evolved in terms of geological history. He will explore several case studies, such as reconstructions of the last universal, bacterial, and archaeal common ancestors in terms of their inferred metabolism in addition to the sum of prokaryotic metabolic cycles available at different important time slices across the history of the early Earth. ![]() Dr Edmund R R Moody, University of Bristol, UK
![]() Dr Edmund R R Moody, University of Bristol, UKDr Moody is a senior research associate at the University of Bristol, currently working on the evolution of microbial lipids used as proxies for palaeoclimate reconstruction in the School of Chemistry. His main area of research is deep time phylogenomics and the evolution of early life on Earth. His most significant papers include a probabilistic reconstruction of the last universal common ancestor (LUCA), in terms of genome, metabolism, and age, as well as the effect on the Earth system; and an investigation into the deepest branch on the tree of life, and the effects of marker gene verticality and conflicting evolutionary signal on the estimate of the divergence between Archaea and Bacteria. |
14:20-14:45 |
The link between Earth and life histories
Modern microbes play, and have been playing, crucial roles in all of Earth’s global geochemical cycles, contributing to global warming, influencing greenhouse gas emissions, fixing carbon and nitrogen from inorganic sources, and producing O2 for us to breathe. They have learned to use light, hydrogen, organic and inorganic forms of carbon-, nitrogen-, sulfur- compounds and transition metals, as electron donors and/ or electron acceptors to generate ATP. From an evolutionary perspective, it is clear that all of the extant microbial diversity cannot have arisen at the same point in time, there had to be a sequence of events. Hence, through microbial evolution, stepwise transitions, from simpler to more complex energy harnessing solutions must have existed. This naturally brings us to one of the key questions of this talk: how can such events be ordered? ![]() Associate Professor Filipa L Sousa, University of Vienna, Austria
![]() Associate Professor Filipa L Sousa, University of Vienna, AustriaFilipa Sousa is an evolutionary microbiologist with a focus on the evolution of metabolic processes. With a background in Chemical Engineering and a PhD in Biochemistry, her current research interests centre around the diversity of energy metabolism, particularly how various metabolic pathways have developed and transformed over time. By exploring the genomic space, she seeks to elucidate the intricate transitions from one metabolic solution to another, providing insights into the complex evolutionary history that has shaped the different ways organisms harness and utilise energy. Through her work, Filipa aims to continue to deepen our understanding of metabolic evolution and its implications for life on Earth. |
14:45-15:00 |
Discussion
|
15:00-15:30 |
Break
|
15:30-15:55 |
The role of cyanobacterial evolution in shaping Earth's oxygen history
Cyanobacteria have played a fundamental role in shaping the history of oxygen on Earth. Traditionally our knowledge of the planet's oxygen history has primarily derived from studies of the geological record. Evolutionary studies and genomic data have provided new insights into the origins of the cyanobacterial crown group and the key cyanobacterial lineages that radiated during the Great Oxidation Event (~2.4 Bya) and the Neoproterozoic Oxidation Event (~800-600 Mya) across different marine habitats. While the ancestors of two major cyanobacterial clades (i.e., Macro- and Microcyanobacteria) evolved in the lead-up to the GOE, marine planktonic clades (i.e., Trichodesmium, Synechococcus, Prochlorococcus) radiated around the NOE. Our analyses trace back the emergence of genes and biosynthetic pathways involved in nitrogen metabolism, multicellularity, motility, circadian rhythms, and salt tolerance, among others. Bayesian analyses predict a marine origin for the last common ancestor of cyanobacteria (LCA) at around ~3.1 Bya, with subsequent expansion into low-salinity and terrestrial habitats at around ~2.8 Bya. Biological events and the intricate evolution of the cyanobacterial phylum contributed to their ecological dominance, influencing the habitability of Earth and causing major disruptions in nutrient cycles (i.e., carbon, nitrogen, oxygen, and iron) in the Proterozoic. The ancestors of cyanobacteria were instrumental in transforming Earth’s atmosphere and biosphere. ![]() Professor Patricia Sánchez-BaracaldoUniversity of Bristol, UK ![]() Professor Patricia Sánchez-BaracaldoUniversity of Bristol, UK Patricia Sanchez-Baracaldo is currently a Professor of Phylogenomics and Microbiology at Bristol University and a Royal Society University Research Fellow. She obtained her PhD in the Department of Integrative Biology at the University of California, Berkeley, and conducted postdoctoral research on the molecular ecology of cyanobacteria at Bristol University. After a five-year career break, she resumed her scientific career with support from two prestigious awards: the Daphne Jackson and Dorothy Hodgkin Royal Society Fellowships. In 2016, she was awarded a Royal Society University Research Fellowship. Patricia pioneered the use of phylogenomics and molecular clocks to study cyanobacterial evolution, with research interests that include photosynthesis, biogeochemical cycles, microbial comparative genomics, and evolutionary biology. By employing genomics and sophisticated Bayesian methodologies, she has developed innovative approaches to explore the interplay between evolution, biochemistry, and the geological record. Patricia has also served on the Rosalind Franklin Award Committee and the Diversity Committee at The Royal Society. |
16:05-16:55 |
Poster flash talks
|
16:55-17:00 |
Discussion
|
17:00-18:00 |
Poster session
|
Chair
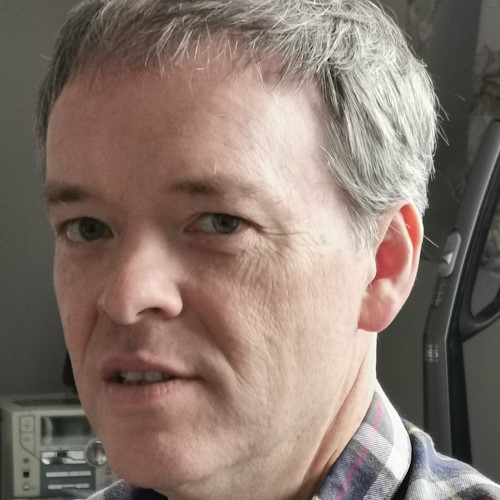
Professor Graham Shields, University College London, UK
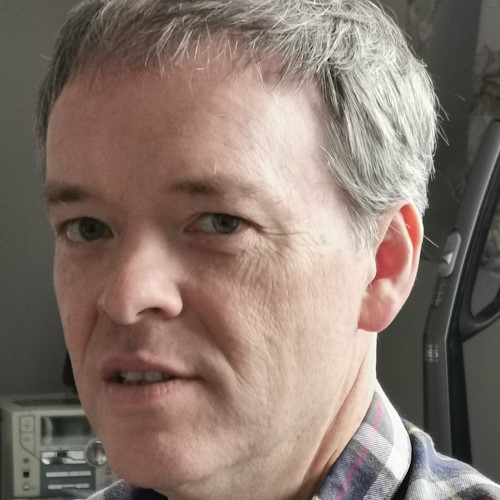
Professor Graham Shields, University College London, UK
Graham Shields is Professor of Chemical Geology at University College London. He received his PhD from the ETH Zurich in 1997 on isotope geology, since which time he has worked around the world on diverse aspects of earth history and sedimentary geochemistry. His research group’s work explores the interactions between the coupled biogeochemical cycles and biological evolution on geological time scales, focussing in particular on major stages in early life evolution. Graham is the author of the book Born of Ice and Fire (Yale University Press), which outlines how global glaciation and major tectonic events paved the way for the emergence and diversification of early animals.
09:00-09:25 |
The evolution of biogeochemical cycles
Our living planet is orders of magnitude more biologically productive than what could be supported if life was solely reliant on the slow release of critical nutrients from Earth’s crust. Highly efficient biogeochemical cycling of these nutrients in the ocean and on land, with an extraordinary efficiency of 99.9%, supports this elevated productivity. The thermodynamic drive for biogeochemical cycling arises from the creation of far-from-equilibrium organic matter produced by chemo-, and especially, photosynthesisers. This organic matter provides the chemical energy to drive a series of chemical reactions energetically dependent on available reactants (largely oxidants, themselves the product of organic matter synthesis) and kinetically accelerated by microorganisms. These boundary conditions set the stage for the emergence of biogeochemical cycles. An in-depth focus on the establishment of an oxygen-rich biosphere nearly 2.5 billion years ago highlights how biogeochemical cycles evolve across such global and irreversible transitions. Interactions among external forcings of Earth’s planetary ecosystems, namely increasing solar luminosity, continental growth, and decreasing volcanism, and internal feedbacks regulating biogeochemical cycles, create a system in which biotic innovation (eg the origin of oxygenic photosynthesis) is not immediately expressed as a change in environmental state (eg atmospheric oxygenation). Counterintuitively, we find that earlier origins of oxygenic photosynthesis lead to longer delays in atmospheric oxygenation. These findings influence considerations of planetary habitability and life detection on exoplanets. ![]() Lee R Kump, Pennsylvania State University, USA
![]() Lee R Kump, Pennsylvania State University, USALee Kump is a biogeochemist recognised for his contributions to our understanding of the factors that drove coupled biological and environmental evolution through Earth history, with a special focus on the carbon cycle, paleoclimates, mass extinctions, and the controls on atmospheric oxygen. He is Professor of Geosciences and John Leone Dean of the College of Earth and Mineral Sciences at Penn State. Kump is an elected member of the National Academy of Sciences, a fellow of the Geological Society of America, the American Geophysical Union, the Geochemical Society, the European Association of Geochemistry, and the Geological Society of London. He is the author of over 150 research publications and has co-authored three textbooks: The Earth System, Dire Predictions, and Mathematical Modeling of Earth’s Dynamic Systems. |
---|---|
09:25-09:50 |
Darwinising Gaia
After briefly describing James Lovelock’s Gaia Hypothesis, Dr Doolittle will argue that Gaia does not reproduce, or rather that it has what Peter Godfrey-Smith would term “too many parents” to undergo natural selection according to Lewontin’s Recipe. So it does not make sense to most Darwinians. If that recipe were extended to include differential persistence as well as differential reproduction, or if the “gene’s-eye view” of Richard Dawkins as further extended by David Hull and us were adopted, then the Gaia Hypothesis, and lesser claims about some multispecies communities, holobionts, and ecosystems, would make sense. That’s what the It’s the song not the singer(s) theory aimed to do. ![]() Dr W Ford Doolittle FRS, Dalhousie University, Canada
![]() Dr W Ford Doolittle FRS, Dalhousie University, CanadaDoolittle obtained his BA from Harvard and his PhD from Stanford, and has been at Dalhousie for 53 years. He is known for the “introns early” hypothesis, the “selfish DNA” hypothesis, stressing the importance of lateral gene transfer, and the “It’s the song not the singer(s)” hypothesis. Also for proving the endosymbiont hypothesis for plastids, developing genetics for Archaea and documenting lateral gene transfer. |
09:50-10:15 |
Were Precambrian oceans devoid of planktonic cyanobacteria? Insights from metabolism
Studies on biospheric evolution often assume planktonic cyanobacteria were present in Precambrian oceans but that their productivity was limited by a variety of ecological and geological factors. However, available evidence suggests that ancestors of extant planktonic cyanobacteria only colonised the ocean in the late Neoproterozoic or early Phanerozoic, geologically close to the start of a major period of atmospheric oxygenation that ultimately triggered the rise of animals. It is an open question whether earlier planktonic marine cyanobacteria existed but went extinct, possibly due to snowball Earth glaciations, and that records of their existence were erased, or that cyanobacteria first colonised the open ocean for the first time during this period. If the latter is true, we lack a consensus explanation for why cyanobacteria did not colonise the open ocean sooner. In this talk, Dr Braakman will review evidence on the metabolic evolution of marine picocyanobacteria, a monophyletic group that dominates extant cyanobacterial primary production, performing around ~25% of oceanic CO2-fixation. Metabolic innovations in this group provide insights into how the marine biosphere may have overcome earlier constraints on productivity, and how the rise of arthropods may have been the key facilitating factor that allowed cyanobacteria to make the evolutionary leap from the benthos to the open ocean, helping transform the biosphere and Earth as a whole. ![]() Dr Rogier Braakman, Massachusetts Institute of Technology, USA
![]() Dr Rogier Braakman, Massachusetts Institute of Technology, USADr Braakman studies metabolic evolution and the self-organisation of ocean microbial ecosystems over geologic time. His group takes an integrative, multi-scale approach to studying living systems using a variety of data and a combination of bioinformatic, experimental and theoretical approaches. He obtained his PhD at Caltech and completed postdoctoral fellowships at the Santa Fe Institute and MIT before moving into his current position as Research Scientist in the department of Earth, Atmospheric & Planetary Sciences at MIT. |
10:15-10:30 |
Discussion
|
10:30-11:00 |
Break
|
11:00-11:25 |
The evolution of metabolism: the source of power for life
In this talk, Dr Falkowski will discuss the biotic evolution of metabolism and the origin of life on Earth. Life derives energy from the external environment and, in so doing moves electrons, with or without protons; a process we call “metabolism”. There is a remarkably small subset of reactions responsible for these reactions. All are based on transition metals bound to a very limited subset of protein structures. These molecules, called “oxidoreductases”, ultimately are responsible for altering the gas composition of a planet. Dr Falkowski will explain the evolution of the protein structures and how these molecules came to make life a thermodynamic non-equilibrium system. ![]() Professor Paul Falkowski, Rutgers University, USA
![]() Professor Paul Falkowski, Rutgers University, USADr Paul Falkowski is a distinguished professor in the Departments of Marine and Coastal Sciences and Earth and Planetary Sciences at Rutgers University. He holds the Bennett L Smith Chair in Business and Natural Resources, served as founding director of the Rutgers Energy Institute, and is the 2018 recipient of the Tyler Prize for Environmental Achievement. |
11:25-11:50 |
The adaptation/ byproduct distinction and the logic of selective explanation: a critique of the Darwinised Gaia hypothesis
The distinction between adaptive significance (function) and “accidental by-product” is fundamental in evolutionary biology. A bird’s mating display may attract predators as well as mates, but the former is a by-product while the latter is the trait’s function, that is, the thing that it is “meant” to do. This distinction was central to the traditional neo-Darwinian objection to the Gaia hypothesis, which was precisely that the life-sustaining homeostatic mechanisms in question were at best a by-product, given the absence of any selection at the level of the whole biosphere. Proponents of the more recent “Darwinised Gaia” idea accept this point, but argue that nonetheless one can reconcile core aspects of the Gaia hypothesis with Darwinian theory. In this paper we turn the spotlight on the distinction between adaptive function and accidental by-product which underlies the debates over Gaia, old and new. We argue that the distinction is clear enough in paradigm cases, but that there is an important class of cases in which it is less clear. These cases arise where there is hierarchical structure, such that selection at one level yields a by-product that is manifest at a higher biological level; and where there is also a second process of selection taking place at the higher level, typically over a different timescale, acting on a higher-level trait for which the lower-level by-product is partly causally responsible. Examples of this selective mechanism are readily found. Where such a mechanism exists, it is unclear whether the biological features in question should be considered adaptive or not. This observation is used to offer fresh light on the validity of the Darwinised Gaia idea. ![]() Professor Samir Okasha FBA, University of Bristol, UK
![]() Professor Samir Okasha FBA, University of Bristol, UKSamir Okasha FBA is Professor of Philosophy at the University of Bristol. He has spent much of his career working on issues in the philosophy of biology, in particular the foundations of evolutionary theory. He also worked on issues in epistemology and metaphysics of science, including causation, induction and probability. Samir has published widely in both philosophical and scientific journals. His 2006 book Evolution and the Levels of Selection was awarded the Lakatos Prize for an outstanding contribution to the philosophy of science. His latest book, Agents and Goals in Evolution, was published in 2018. He is also the author of Philosophy of Science: a very short introduction, and Philosophy of Biology: a very short introduction, both published by Oxford University Press. ![]() Dr Margarida HermidaKing’s College London, UK ![]() Dr Margarida HermidaKing’s College London, UK |
11:50-12:15 |
Quasi-Darwinian persistence selection of biogeochemical cycle variants for their climatic impacts
The average long-term impact of Darwinian evolution on Earth’s habitability for life remains extremely uncertain, largely due to a separation of spatial and temporal scales between biological populations undergoing natural selection and planetary-climatic metrics for habitability. Recent attempts to bridge this gap by “Darwinising” non-replicating biogeochemical processes are unclear about the exact nature of the varying populations subject to putative persistence-selection. A model framework is presented to explicate persistence selection between distinct variants of global-scale biogeochemical cycles:
CBV-level persistence selection thus operates on quasi-Darwinian individuals defined by the existence of a connection between (1) and (2). The timescale separation in (3) distinguishes this process from a generic byproducts-based null hypothesis. Regions of parameter space supporting the above results are characterised by covariance between these two sets of properties, analogous to the covariance between fitness and traits required by conventional Darwinian selection. These general ideas broadly conform with aspects of the historical geochemical and phylogenetic records. ![]() Dr Richard Boyle, University of Exeter, UK
![]() Dr Richard Boyle, University of Exeter, UKRichard Boyle is interested in the long-term coevolution of life and the “Earth system”. He initially studied evolutionary biology before gaining a PhD in Earth system modelling. His research involves mathematical modelling of changes in biogeochemical feedbacks introduced by life, and the effect of climatic context on biological evolution, particularly transitions in the level at which selection operates. He is also interested in the feasibility and coherence of the various attempts to reconcile the “Gaia” hypothesis with Darwinian evolution. |
12:15-12:30 |
Discussion
|
Chair
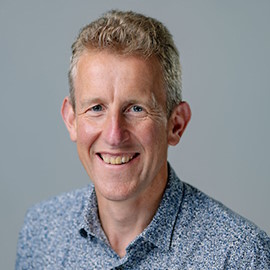
Professor Tim Lenton, University of Exeter, UK
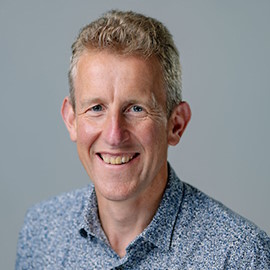
Professor Tim Lenton, University of Exeter, UK
Professor Tim Lenton is the founding Director of the Global Systems Institute at the University of Exeter and Chair in Climate Change and Earth System Science. He has more than 25 years research experience, focused on modelling of the biosphere, climate, biogeochemical cycles, and associated tipping points. Tim is renowned for his work identifying climate tipping points, which informed the setting of the 1.5C climate target, associated net zero targets, and nationally determined contributions.
Tim works with policymakers and businesses helping them assess the risks of climate change and nature loss and highlighting the opportunities for "positive tipping points" that can accelerate change towards net zero. In 2023, Professor Lenton led a team of more than 200 people from over 90 organisations in 26 countries to produce an authoritative assessment of the risks and opportunities of both negative and positive tipping points in the Earth system and society. The Global Tipping Points Report produced in partnership with Bezos Earth Fund was published at COP28.
13:30-13:55 |
Planetary habitability: from our biosphere to biospheres?
A plausibility argument is given for how Gaian regulation can evolve from sub-global competition. The earliest metabolisms of non-photosynthetic microbial mats running the Wood-Ljundahl Pathway backwards and forwards could regulate pre-existing atmospheric feedbacks, thus supporting the Gaian Bottleneck Hypothesis. Under specific plausible assumptions, the recent earlier estimates for the origin of life on Earth (~ 4.2 Gya rather than 3.8 Gya) increase the probability of life elsewhere. A connection is described between (i) the evolution of inanimate and animate catalysis (senso lato) and (ii) optimizing entropy production under thermal- and information-dependent constraints. We propose the concept of “entropy-driven gaia” in which the evolution of catalysis and global biogeochemical cycles on Earth (and elsewhere?) is a subset of ubiquitous and universal far from equilibrium dissipative systems. ![]() Professor Charles LineweaverAustralian National University, Australia ![]() Professor Charles LineweaverAustralian National University, Australia Astrobiologist, Charles H Lineweaver is an honorary associate professor at the Australian National University’s Research School of Astronomy and Astrophysics. His research areas include exoplanetology (the statistical analysis of exoplanets and their habitability), cosmobiology (using our new knowledge of cosmology to constrain life in the Universe) and cancer (developing a new atavistic model of oncogenesis). He recently completed an online video MOOC at arewealone.us. Dr Lineweaver earned a BA in history from the State University of New York at Binghamton, an MA in English from Brown University, a BS is physics from Ludwig Maximillian's University in Munich, and a PhD in physics at the University of California, Berkeley in 1994. He is the son of a high school biology teacher and has lived in or travelled through 85 countries, has spoken 4 languages semi-fluently at one time or another, and was a semi-professional soccer player in New England and Germany. |
---|---|
13:55-14:20 |
Searching for exobiospheres
The search for signs of life beyond Earth is a key motivator in exoplanet research. A suitable “biosignature gas” is one that: can accumulate in an atmosphere against atmospheric radicals and other sinks; has strong atmospheric spectral features; and has limited abiological false positives. We now have a long list of potential biosignature gases including isoprene which is produced by life in as high quantities as methane, and phosphine which on Earth is only associated with life. Despite promise from the successfully operational JWST, we are now confronted with practical challenges of tiny signals and M dwarf star contamination. Another severe challenge is the unknown exoplanetary environments likely vastly different from Solar System planets. We review the thousands of molecules produced by life on Earth in context with the phosphine on Venus and methane on Mars prescient backdrop for a reality check on the future of exoplanet biosignature gases. Our pace and history of milestone discovery in exoplanets in the last three decades, combined with new telescope paradigms, promises to eventually deliver on finding signs of life beyond Earth. ![]() Professor Sara Seager OC, Massachusetts Institute of Technology, USA
![]() Professor Sara Seager OC, Massachusetts Institute of Technology, USAProfessor Sara Seager is a Professor of Physics, Planetary Science, and Aeronautics and Astronautics at the Massachusetts Institute of Technology where she holds the Class of 1941 Professor Chair. Her ground-breaking research ranges from the foundation of exoplanet atmospheres to innovative theories about life on other worlds to development of novel space mission concepts. She was the Deputy Science Director of the NASA mission TESS; PI of the JPL-MIT CubeSat ASTERIA; and has had numerous leadership roles in concept development for space-based direct imaging missions to discover another Earth. She currently leads the Morning Star Missions to Venus to search for signs of life or life itself in the Venus clouds. Her many accolades include a MacArthur Foundation “genius” grant, the Kavli Prize in Astrophysics, and appointment as an Officer of the Order of Canada. She is the author of The Smallest Lights in the Universe: A Memoir. |
14:20-14:45 |
The hard steps model and whole Earth-system transitions
The hard steps model is a simple formulation of the evolution towards complex life on Earth and, by implication, on other planets. It assumes that, at the longest time scale, the rate at which evolution occurs is paced by the occurrence of difficult “critical” transitions that happen randomly and rarely. Applied to Earth, the model suggests there have been just a small number of such transitions (three or four, widely spaced in time) in the pathway to “intelligent observers”: humans. While the transitions in the model are usually envisaged as purely biological evolutionary innovations, this misses the reality that, on Earth, the evolution of life and the planetary environment have been inextricably linked. The scale of the transformations that have occurred on Earth suggests that the critical steps should be seen as whole-system transitions. Specifically, the Paleoproterozoic and Neoproterozoic glacial episodes are candidates for such transitions: they were both caused by, and drivers of, fundamental and wide-ranging biological evolutionary advances that were necessary for complex life to arise. The Paleoproterozoic glacial periods were probably triggered by the appearance of free oxygen in the atmosphere (itself the delayed result of oxygenic photosynthesis that originated hundreds of millions of years earlier). These glaciations set the stage and may have been necessary for the evolution of eukaryotes. Much later, the Neoproterozoic glaciations appear to have triggered the radiation of Metazoa, and the inception of the modern Earth inhabited by large and complex organisms. If the rise of a technologically adept species such as humans is considered another such transition, it is perhaps no surprise that it too is being accompanied by climate change, that could be as catastrophic as these past crises unless we act decisively to prevent it. ![]() Professor Andrew Watson FRS, University of Exeter, UK
![]() Professor Andrew Watson FRS, University of Exeter, UKAndy Watson is Royal Society Research Professor at the University of Exeter. He is an Earth System scientist, with a special interest in the processes controlling the composition of the atmosphere, especially carbon dioxide and oxygen concentrations, and their connection to the Earth’s climate. He has contributed to a wide variety of topics, including the atmospheres of other planets, the physics and biogeochemistry of the oceans, paleoclimatology and astrobiology. His research group at the University of Exeter specialises in making and interpreting ocean and atmospheric measurements to high accuracy. He was made a Fellow of the Royal Society in 2003. |
14:45-15:00 |
Discussion
|
15:00-15:30 |
Break
|
15:30-15:55 |
Modelling the evolution of exobiospheres
When searching for life in the galaxy we need to know what remotely detectable signatures life might leave for us to detect. The evolutionary pathway life takes on a planet will be specific to each planet, impacted by its bulk properties, and will dictate the planet’s biosignatures. As distance prevents us from directly observing any life on an exoplanet, instead we can use computer models informed by Earth history to reduce the number of assumptions needed to contemplate alien biospheres, and to make informed biosignature predictions. Using a 0D model based on early-Earth, this talk will demonstrate that, for biosignatures resulting from energy extraction by organisms, the metabolism of the organism is the most important factor in determining the biosignature 'strength' (eg the level of oxygen in Earth’s atmosphere). The abundance of the organism itself does not largely impact the resulting biosignature. This allows us to consider alien-life in terms of metabolisms that are possible on the planet. Then, using an abstract biological model of co-evolution, this talk will then demonstrate that the expected trajectory for any biosphere is to become more complex over time. This allows us to make the predictions on the complexity of exobiospheres and, thus, paves the way to assembling models of likely ecosystems for specific planets. Detailed biosignature predictions will need to be bespoke for each planet. The models presented in this talk present a way to construct these predictions using fewer assumptions. ![]() Dr Arwen Nicholson, University of Exeter, UK
![]() Dr Arwen Nicholson, University of Exeter, UKArwen’s PhD focused on developing models of Gaian regulation. She developed abstract models of life-environment coupled-systems, informed by Earth’s history, to study the influence of life on climate evolution. Since her PhD she has worked in the Physics Department at Exeter applying Gaia Theory to the search for alien life. This work has included determining the most important parameters required to quantify the abundance of biosignatures gases of nutrient limited biospheres, and developing models of how the presence of Gaian regulation might influence the habitable zone around planets. Arwen has also co-written public outreach articles on the science behind the slogan: There’s no planet B. |
15:55-16:20 |
Planetary responsibility in the Anthropocene
The energy balance of a planet is set by the sunlight entering and the heat radiating back from it. Earth’s biosphere adds new complexity as energy flows through global marine and terrestrial ecosystems altering physical and chemical systems, including global climate. Energy is essential for life, and the main constraint on abundance and productivity is the amount of energy an organism can harvest. When the first lifeforms evolved fire-making culture (there is evidence in our hominin ancestors, some 1 million years ago) it sparked a novel form of energy relationship: deliberate combustion, which would change the planet. The innate drive to harvest ever more energy changed the evolution of our species and transformed the world’s environment. Humans became the dominant species globally. In recent decades, however, the impact of humanity’s burning of ancient fossil life has altered the energy balance of the entire planet. As humans become ever more successful and ingenious at harnessing energy, our scope grows extraterrestrial: there are plans to colonise the Moon and Mars. Meanwhile, the destabilising impact of humanity’s energy exploits on Earth’s unique biosphere risk the survival of multiple ecosystems, and the future of our own species. As the dominant, and consciously capable, species, does this imbue us with a responsibility over other life on earth. ![]() Gaia Vince, UK
![]() Gaia Vince, UKGaia Vince is a science writer and broadcaster exploring the interplay between human systems and the planetary environment. She is an Honorary Senior Research Fellow at the Anthropocene Institute at UCL and a host of BBC Inside Science. Her first book, Adventures In The Anthropocene, won the Royal Society Science Book of the Year Prize. Her latest book, Nomad Century: How To Survive The Climate Upheaval, explores global migration and planetary restoration in a radical call to arms. |
16:20-17:00 |
Discussion
|